Functional Disconnection Between the Prefrontal and Parietal Cortices During Working Memory Processing in Schizophrenia: A [15O]H2O PET Study
Abstract
OBJECTIVE: The prefrontal-parietal circuits have been reported to play an important role in working memory. The purpose of this study was to use direct neuroimaging to address whether interregional correlation in the prefrontal-parietal circuits is impaired in schizophrenia. METHOD: [15O]H2O positron emission tomography was used to compare regional blood flow changes in 12 schizophrenic and 12 healthy comparison subjects during the n-back sequential-picture working memory task. Interregional correlation was assessed by correlating the regional activation of the lateral prefrontal area with that of other activated areas in each subject group. RESULTS: Dorsolateral prefrontal activation was observed around the right superior frontal sulcus in the healthy subjects, and ventrolateral prefrontal activation below the right inferior frontal sulcus was observed in the schizophrenic patients. Activation in the right lateral prefrontal cortex was significantly correlated with activation in the bilateral inferior parietal region in the healthy subjects but was not correlated with any regional activation in the patients. CONCLUSIONS: The findings suggest prefrontal-parietal functional disconnection, particularly prefrontal dissociation and abnormal prefrontal-parietal interaction, during working memory processing in schizophrenia.
Working memory, the process of holding and manipulating information online in the brain (1), is a critical component of normal cognition. Working memory deficits have been considered a cardinal cognitive dysfunction and the possible basis of the diverse clinical symptoms of schizophrenia (2). Previous functional imaging studies have shown that working memory is subserved by the lateral prefrontal cortex and its reciprocal cortical connections with other brain regions, such as the cingulate and temporal and parietal cortices (3, 4). In contrast, the most consistent finding during working memory tasks, such as the n-back test, in patients with schizophrenia has been subnormal activations of the lateral prefrontal cortex and other regions in the working memory network (5, 6). However, these subnormal activations, including “task-related hypofrontality,” have been challenged as a possible artifact resulting from the poor performance of schizophrenic subjects.
In general, the majority of findings from cognitive activation studies have been interpreted in terms of the distributed neural circuits, and dysfunctional activation patterns in patients with schizophrenia have been considered to be associated with a circuit disconnection (7). A failure in interactive functional connectivity between distant cortical areas in schizophrenia may curtail the cortical circuit integration needed in working memory (8, 9). However, despite the abundance of dysfunctional findings in multiple regions, there is no direct neuroimaging evidence of abnormal cortico-cortical functional connectivity related to working memory in schizophrenia.
This study was designed to determine whether functional connectivity in the working memory network is impaired in schizophrenia when patients perform routine working memory tasks. We hypothesized that subjects would show lateral prefrontal activation during n-back working memory challenges but that patients with schizophrenia would show unique patterns of prefrontal and other cortical activations, including evidence of functional disconnection within the working memory network. Functional connectivity was assessed by correlating the degree of regional activation detected by means of [15O]H2O positron emission tomography (PET) during working memory tasks between the lateral prefrontal cortex and other brain areas.
Method
Subjects
The subjects were 12 patients with schizophrenia and 12 age- and sex-matched healthy volunteers. Each group contained six men and six women, all of whom were right-handed. The mean ages of the patients and the healthy volunteers were 26.2 years (SD=4.0, range=20–32) and 25.6 years (SD=4.8, range=19–35), respectively. The number of years of education (mean=14.7 years, SD=1.6, in the patient group and mean=15.3 years, SD=1.6, in the healthy group) and parental socioeconomic status (mean=2.9, SD=1.1, and mean=3.2, SD=1.0, respectively, on the Hollingshead Index of Social Position) were not significantly different between the two groups.
Patients were recruited from the inpatient unit and outpatient clinics at the Seoul National University Hospital. They fulfilled the DSM-IV criteria for schizophrenia, as diagnosed by using the Structured Clinical Interview for DSM-IV (SCID) (10). The exclusion criteria for the study were any lifetime history of neurological or significant medical illnesses and any past history of substance abuse. The healthy volunteers were recruited from the local community and were screened for any current or lifetime history of a DSM-IV axis I disorder by using the SCID. The study was carried out under the guidelines established by the institutional review board of Seoul National University Hospital for research involving human subjects, and written informed consent was obtained from all subjects.
At the time of PET scanning, the patients had a mean duration of illness of 2.8 years (SD=3.2). Symptom severity was assessed with the Positive and Negative Syndrome Scale (11); the patients’ mean scores for positive (mean=14.1, SD=6.6, range=8–31), negative (mean=17.6, SD=6.8, range=7–30), and general (mean=31.3, SD=6.0, range=20–44) symptoms and mean total score (mean=62.9, SD=10.7, range=45–78) were mostly in the questionable to mild range. All patients received one or two atypical neuroleptics, such as risperidone, olanzapine, and clozapine.
Cognitive Tasks
This study was performed as a part of six consecutive PET scans; the tasks and imaging procedures have been described in detail elsewhere (12). Task stimuli were visually projected as simple pictures, such as a circle, rectangle, or triangle, in white on the black background of a liquid crystal display computer monitor. Under the experimental condition (2-back working memory task), the subjects were required to continuously monitor a sequence of stimuli and to respond whenever a circle was presented a second time after one intervening stimulus. The control condition (focused attention) involved responding whenever a circle was presented. Each task consisted of 80 stimulus items, including 28 targets, and stimuli were projected for 500 msec at 1.5-second intervals. The frequency and distribution of targets were matched in the experimental and control tasks. The order of the tasks was varied among subjects. To prevent subjects’ poor performance from introducing a confounding factor, all subjects participated in an explanatory session before PET scanning. All subjects read instructions elucidating the rules of the task and practiced the procedure by using printed illustrations until they achieved adequate performance.
Imaging Data Acquisition and Data Processing
Scans were obtained by using an ECAT EXACT 47 scanner (Siemens-CTI, Knoxville, Tenn.). After a 7-minute transmission scan for attenuation correction, emission scans were recorded during the performance of cognitive tasks after an intravenous bolus injection of 40–50 mCi of [15O]H2O in 5–7 ml of saline and were continued for 100 seconds in 20 5-second frames. On the basis of time-activity curves, only the 12 frames reflecting the 60 seconds after peak arrival of the bolus of contrast agent were summed. Spatial preprocessing and statistical analysis were performed by using statistical parametric mapping (SPM 99) (Institute of Neurology, University College London). All reconstructed images were realigned and transformed into a standard stereotactic anatomical space (13) by using affine and nonlinear transformations. Spatially normalized images were smoothed by convolution with an isotropic Gaussian kernel with 16 mm full width at half maximum.
Statistical Analyses
To remove the effect of variance due to global activity within and between subjects, the global cerebral blood flow (CBF) of each subject was calculated as mean regional CBF (rCBF) over all intracerebral voxels. The adjusted rCBF of each subject for each voxel was generated by proportional scaling to a global CBF of 50 ml/minute per deciliter. A voxel-based comparison of the adjusted rCBF values under the different conditions was performed in each group by using paired t tests. The resulting t values were transformed to z scores. Only regions showing increased rCBF were considered to be activated. Statistical test results with an uncorrected p level <0.005 were considered significant. Clusters of at least 50 contiguous significant voxels were interpreted as an activated region.
Post hoc analysis was performed to evaluate interregional correlations. The adjusted mean regional activity was counted by averaging values of the condition-specific adjusted rCBF for all voxels within the region of interest corresponding to the cluster that was composed of significant contiguous voxels in the comparison described earlier. Percentage changes in regional activity were calculated with the following formula: 100 × (POST – PRE)/PRE, in which POST was the adjusted mean regional activity during the working memory task and PRE was the adjusted mean regional activity during the control task. Spearman correlations between lateral prefrontal activation and other regional activations were computed in each group. The significance of the correlations was accepted when p<0.05.
Subsequent analyses were performed to test for group differences in interregional correlations. Activity within the regions of interest corresponding to the clusters with significant activation in either study group was counted for the opposite group in both the working memory and the control conditions. The ratios of the differences in activity between the lateral prefrontal cortex and each of the other regions of interest in each of the study conditions were calculated for every subject. Group differences in the ratios were analyzed by using the Wilcoxon rank sum test. Significance was accepted when p<0.05.
Results
Behavioral performance was monitored to ascertain how well the subjects paid attention to the tasks and to examine the differences among the task conditions. The mean rate of correct responses for the experimental and control tasks were 99.3% (SD=1.0%) and 99.8% (SD=0.5%), respectively, in the healthy group and 97.9% (SD=3.5%) and 99.6% (SD=0.6%), respectively, in the patient group. The difference in the rate of correct responses was not significant (F=2.5, df=3, 44, p=0.07).
Table 1 shows the results of subtracting the adjusted mean activity in the control condition from that in the experimental condition for the regions of interest in the two study groups. As shown in Figure 1, lateral prefrontal activation was observed in the dorsal portion around the right superior frontal sulcus (Brodmann’s area 8) in the healthy group and in the ventral portion below the right inferior frontal sulcus (Brodmann’s area 45) in the patient group. Left frontal activation was observed in the frontal pole in the healthy group but in the inferior frontal gyrus in the patient group. Parietal activation was identified bilaterally in the healthy group but only in the left side in the patient group. On the other hand, cerebellar activation was found only in the left side in the healthy group but was found bilaterally in the patient group. Small but significant activations in the right anterior cingulate and the left putamen were found in the healthy group but not in the patient group.
Regional activity changes and their correlations with right lateral prefrontal activity change in each group are also presented in Table 1. Activations in the left frontal pole and both sides of the inferior parietal cortex were significantly correlated with right lateral prefrontal activation in the healthy group, whereas no significant correlation was observed in the patient group. As shown by the p values reported for the Wilcoxon rank sum test in Table 1, the statistical significance of the intergroup comparisons of interregional correlations of activation was similar for the healthy and the schizophrenic subjects.
Discussion
Although numerous studies have addressed the possibility of “hypofrontality” in patients with schizophrenia, a recent PET study demonstrated that hyper- and hypoactive regions intermingle in the prefrontal area during the resting state (14). Task-related hypofrontality has been reported in schizophrenic patients while they performed the n-back working memory challenge (5, 6), but debate has continued because poor performance in patients may be responsible for the findings of hypoactivity. Neuroimaging findings during poor performance may reflect poor effort or motivation, a feeling of being overwhelmed, or simply guessing, and thus disengagement from the task. To observe activation patterns related to working memory in schizophrenia in the absence of the confounding factor of task performance, we trained all subjects in the working memory task before PET scanning. All subjects performed the task well, as shown by the results.
As expected, the patients with schizophrenia in the current study showed a distinct prefrontal activation pattern rather than “hypofrontality.” Right ventrolateral prefrontal cortex activation below the inferior frontal sulcus was found in the patients, and right dorsolateral prefrontal cortex activation around the superior frontal sulcus was found in the healthy subjects. These findings suggest that healthy and schizophrenic subjects use different lateral prefrontal regions for performing the working memory task. Given that the lateral prefrontal cortex has functional subdivisions that are specialized for some component processes of working memory (15, 16), the distinct activation pattern for the schizophrenic subjects in the current study may reflect the use of different strategies in the executive control process. Although the patients and the healthy subjects had similarly good performance on the working memory task as a result of the pretask training, the patients might still have had some problems, such as peculiar responses, reduced effectiveness, or delayed responsiveness in the performance of working memory tasks.
Alternatively, the activation pattern of the schizophrenic subjects may be more relevant to a dorsal/ventral dissociation, particularly reflecting different lateral prefrontal regions required for different operations. For example, a two-stage model that includes two processing systems within the lateral prefrontal cortex has been proposed (17). In that model, the dorsal prefrontal cortex is recruited when active manipulation or monitoring within working memory is required, whereas the ventral prefrontal cortex is the site where active comparisons of information held in working memory are made. If such functional subdivision of executive manipulation versus active maintenance is also relevant to our findings, it may suggest that performing working memory in patients with schizophrenia tends to depend on a mnemonic process rather than a normally dominant executive process. A recent functional magnetic resonance imaging study reported dysfunction of the right dorsolateral prefrontal cortex in patients with schizophrenia who performed poorly during n-back working memory tasks (18), suggesting deficits in more associative and executive components of working memory rather than a deficit in the maintenance or rehearsal of stimulus representations. Therefore, to reach normal performance for working memory, patients with schizophrenia may use the intact ventrolateral prefrontal cortex more actively than the dysfunctional dorsolateral prefrontal cortex.
In addition, this distinct pattern of prefrontal activation can be interactively related to distinct activations within the parietal cortex, i.e., the absence of normal bilateral inferior parietal activation in patients. Interregional correlation, a key issue of the current study, was addressed by computing correlations of degrees of activity changes between the lateral prefrontal activation area and other activated areas during the performance of the working memory task. Our results provide direct neuroimaging evidence of the parallel activation of the prefrontal and inferior parietal cortices in healthy subjects. These two regions have been reported to often show conjoint activation and to be important nodes in a neural network mediating spatial and verbal working memory (19, 20). The prefrontal and inferior parietal cortices are connected by particularly dense fibers and have common cortical and subcortical target regions (21, 22).
In addition to lacking inferior parietal activation, the schizophrenic subjects in this study did not show any significant correlations between the lateral prefrontal cortex and other activation sites. These findings suggest underlying deficits in the integration of the dorsolateral prefrontal and inferior parietal circuits in schizophrenia and thus support the concept that schizophrenia is pathophysiologically a disconnection syndrome (7, 23). In fact, several functional imaging studies addressing functional connectivity in schizophrenia have focused on reduced functional connectivity of the frontotemporal circuits rather than frontoparietal connectivity (24, 25). Even in a PET study investigating functional connectivity during working memory, disruptions of frontotemporal connectivity were found (9). Evidence of the disconnection during working memory tasks among circuit regions including the parietal lobe was suggested in a recent electrophysiological study (26), although the significance of the findings is limited because of poor spatial resolution. Our data more precisely point to a functional disconnection between the right dorsolateral prefrontal cortex and the inferior parietal cortex in schizophrenia.
The apparent lack of prefrontal-parietal correlations in patients may be simply due to less dense connectivity between the ventral, compared with the dorsal, prefrontal cortex and parietal cortex. This difference was evident in findings related to cortico-cortical connectivity between the dorsal and ventral prefrontal cortex in the monkey. When a retrograde tracer was injected into the dorsal region (Brodmann’s area 8) in the monkey brain, distant projections included the caudal part of the lower bank of the intraparietal sulcus. However, when a tracer was injected into the ventral region (Brodmann’s area 45), distant projection to the parietal lobe was not observed (27). Therefore, absence of interregional correlation in the dorsolateral prefrontal-inferior parietal circuit in schizophrenia appears to be closely related to a dorsal-ventral prefrontal dissociation of dysfunctional dorsal and relatively intact ventral function.
In summary, our findings show a difference in the right lateral prefrontal cortex associated with working memory in patients with schizophrenia that is closely related to a deficit in functional connectivity to the inferior parietal cortex. This finding may support the disconnection hypothesis in the pathophysiology of schizophrenia. Future studies will be necessary to evaluate whether the disconnection stems from an interactive plastic change or from an abnormal innervation related to a developmental anomaly.
![]() |
Received May 6, 2002; revision received Oct. 29, 2002; accepted Oct. 31, 2002. From the Department of Psychiatry and Nuclear Medicine, Seoul National University College of Medicine, Seoul, Korea; and the Department of Psychiatry, Yonsei University College of Medicine, Seoul, Korea. Address reprint requests to Dr. Kwon, Department of Psychiatry, Seoul National University Hospital, 28 Yongun-dong, Chongno-gu, Seoul, Korea 110-744; [email protected] (e-mail). Supported by grant HMP-00-B-21300-0201 from the Korea Health 21 R&D Project of the Ministry of Health and Welfare, Republic of Korea.
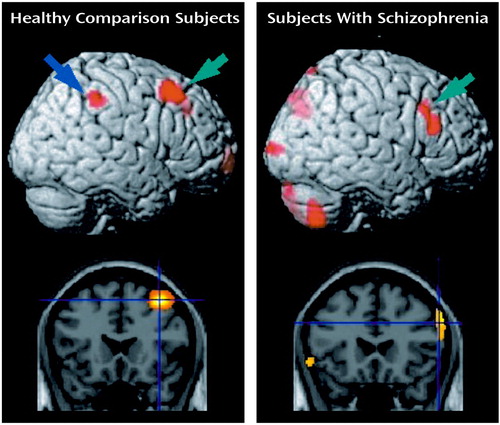
Figure 1. Images of Significant Changes in Regional Cerebral Blood Flow During a Working Memory Task in Healthy Comparison Subjects (N=12) and Subjects With Schizophrenia (N=12)a
aLateral prefrontal activation as indicated by green arrows in the rendered brain and by cross-hairs in the coronal section was observed around the right superior frontal sulcus in the healthy comparison subjects but below the right inferior frontal sulcus in the subjects with schizophrenia. Inferior parietal activation (blue arrow) was identified in the healthy comparison subjects but not in the subjects with schizophrenia.
1. Baddeley A: Working memory. Science 1992; 255:556-559Crossref, Medline, Google Scholar
2. Goldman-Rakic PS: Working memory dysfunction in schizophrenia. J Neuropsychiatry Clin Neurosci 1994; 6:348-357Crossref, Medline, Google Scholar
3. Courtney SM, Petit L, Maisog JM, Ungerleider LG, Haxby JV: An area specialized for spatial working memory in human frontal cortex. Science 1998; 279:1347-1351Crossref, Medline, Google Scholar
4. Jonides J, Smith EE, Koeppe RA, Awh E, Minoshima S, Mintun MA: Spatial working memory in humans as revealed by PET. Nature 1993; 363:623-625Crossref, Medline, Google Scholar
5. Carter CS, Perlstein W, Ganguli R, Brar J, Mintun M, Cohen JD: Functional hypofrontality and working memory dysfunction in schizophrenia. Am J Psychiatry 1998; 155:1285-1287Link, Google Scholar
6. Menon V, Anagnoson RT, Mathalon DH, Glover GH, Pfefferbaum A: Functional neuroanatomy of auditory working memory in schizophrenia: relation to positive and negative symptoms. Neuroimage 2001; 13:433-446Crossref, Medline, Google Scholar
7. Andreasen NC, Paradiso S, O’Leary DS: “Cognitive dysmetria” as an integrative theory of schizophrenia: a dysfunction in cortical-subcortical-cerebellar circuitry? Schizophr Bull 1998; 24:203-218Crossref, Medline, Google Scholar
8. Friston KJ, Frith CD, Fletcher P, Liddle PF, Frackowiak RS: Functional topography: multidimensional scaling and functional connectivity in the brain. Cereb Cortex 1996; 6:156-164Crossref, Medline, Google Scholar
9. Meyer-Lindenberg A, Poline J-B, Kohn PD, Holt JL, Egan MF, Weinberger DR, Berman KF: Evidence for abnormal cortical functional connectivity during working memory in schizophrenia. Am J Psychiatry 2001; 158:1809-1817Link, Google Scholar
10. First MB, Spitzer RL, Gibbon M, Williams JBW: Structured Clinical Interview for DSM-IV Axis I Disorders (SCID). New York, New York State Psychiatric Institute, Biometrics Research, 1996Google Scholar
11. Kay SR, Fiszbein A, Opler LA: The Positive and Negative Syndrome Scale (PANSS) for schizophrenia. Schizophr Bull 1987; 13:261-276Crossref, Medline, Google Scholar
12. Kim JJ, Kim MS, Lee JS, Lee DS, Lee MC, Kwon JS: Dissociation of working memory processing associated with native and second languages: PET investigation. Neuroimage 2002; 15:879-891Crossref, Medline, Google Scholar
13. Talairach J, Tournoux P: Co-Planar Stereotaxic Atlas of the Human Brain: Three-Dimensional Proportional System. New York, Thieme Medical, 1988Google Scholar
14. Kim J-J, Mohamed S, Andreasen NC, O’Leary DS, Watkins GL, Boles Ponto LL, Hichwa RD: Regional neural dysfunctions in chronic schizophrenia studied with positron emission tomography. Am J Psychiatry 2000; 157:542-548Link, Google Scholar
15. Smith EE, Jonides J, Koeppe RA: Dissociating verbal and spatial working memory using PET. Cereb Cortex 1996; 6:11-20Crossref, Medline, Google Scholar
16. Belger A, Puce A, Krystal JH, Gore JC, Goldman-Rakic P, McCarthy G: Dissociation of mnemonic and perceptual processes during spatial and nonspatial working memory using fMRI. Hum Brain Mapp 1998; 6:14-32Crossref, Medline, Google Scholar
17. Petrides M: Frontal lobes and working memory: evidence from investigations of the effects of cortical excisions in nonhuman primates, in Handbook of Neuropsychology, vol 9. Edited by Boller F, Grafman J. Amsterdam, Elsevier Science, 1994, pp 59-82Google Scholar
18. Perlstein WM, Carter CS, Noll DC, Cohen JD: Relation of prefrontal cortex dysfunction to working memory and symptoms in schizophrenia. Am J Psychiatry 2001; 158:1105-1113Link, Google Scholar
19. Friedman HR, Goldman-Rakic PS: Coactivation of prefrontal cortex and inferior parietal cortex in working memory tasks revealed by 2DG functional mapping in the rhesus monkey. J Neurosci 1994; 14:2775-2788Crossref, Medline, Google Scholar
20. Jonides J, Schumacher EH, Smith EE, Koeppe RA, Awh E, Reuter-Lorenz PA, Marshuetz C, Willis CR: The role of parietal cortex in verbal working memory. J Neurosci 1998; 18:5026-5034Crossref, Medline, Google Scholar
21. Petrides M, Pandya DN: Projections to the frontal cortex from the posterior parietal region in the rhesus monkey. J Comp Neurol 1984; 228:105-116Crossref, Medline, Google Scholar
22. Chafee MV, Goldman-Rakic PS: Inactivation of parietal and prefrontal cortex reveals interdependence of neural activity during memory-guided saccades. J Neurophysiol 2000; 83:1550-1566Crossref, Medline, Google Scholar
23. Friston KJ: The disconnection hypothesis. Schizophr Res 1998; 30:115-125Crossref, Medline, Google Scholar
24. Fletcher P, McKenna PJ, Friston KJ, Frith CD, Dolan RJ: Abnormal cingulate modulation of fronto-temporal connectivity in schizophrenia. Neuroimage 1999; 9:337-342Crossref, Medline, Google Scholar
25. Lawrie SM, Buechel C, Whalley HC, Frith CD, Friston KJ, Johnstone EC: Reduced frontotemporal functional connectivity in schizophrenia associated with auditory hallucinations. Biol Psychiatry 2002; 51:1008-1011Crossref, Medline, Google Scholar
26. Peled A, Geva AB, Kremen WS, Blankfeld HM, Esfandiarfard R, Nordahl TE: Functional connectivity and working memory in schizophrenia: an EEG study. Int J Neurosci 2001; 106:47-61Crossref, Medline, Google Scholar
27. Pandya DN, Yeterian EH: Comparison of prefrontal architecture and connections, in The Prefrontal Cortex: Executive and Cognitive Functions. Edited by Roberts AC, Robbins TW, Weiskrantz L. New York, Oxford University Press, 1998, pp 51-66Google Scholar