Relation of Prefrontal Cortex Dysfunction to Working Memory and Symptoms in Schizophrenia
Abstract
OBJECTIVE: The dorsolateral prefrontal cortex has been implicated in both working memory and the pathophysiology of schizophrenia. A relationship among dorsolateral prefrontal cortex activity, working memory dysfunction, and symptoms in schizophrenia has not been firmly established, partly because of generalized cognitive impairments in patients and task complexity. Using tasks that parametrically manipulated working memory load, the authors tested three hypotheses: 1) patients with schizophrenia differ in prefrontal activity only when behavioral performance differentiates them from healthy comparison subjects, 2) dorsolateral prefrontal cortex dysfunction is associated with poorer task performance, and 3) dorsolateral prefrontal cortex dysfunction is associated with cognitive disorganization but not negative or positive symptoms. METHOD: Seventeen conventionally medicated patients with schizophrenia and 16 healthy comparison subjects underwent functional magnetic resonance imaging while performing multiple levels of the “n-back” sequential-letter working memory task. RESULTS: Patients with schizophrenia showed a deficit in physiological activation of the right dorsolateral prefrontal cortex (Brodmann’s area 46/9) in the context of normal task-dependent activity in other regions, but only under the condition that distinguished them from comparison subjects on task performance. Patients with greater dorsolateral prefrontal cortex dysfunction performed more poorly. Dorsolateral prefrontal cortex dysfunction was selectively associated with disorganization symptoms. CONCLUSIONS: These results are consistent with the hypotheses that working memory dysfunction in patients with schizophrenia is caused by a disturbance of the dorsolateral prefrontal cortex and that this disturbance is selectively associated with cognitive disorganization. Further, the pattern of behavioral performance suggests that dorsolateral prefrontal cortex dysfunction does not reflect a deficit in the maintenance of stimulus representations per se but points to deficits in more associative components of working memory.
Schizophrenia has long been considered a disease of brain function that is reflected in a host of cognitive impairments in addition to the classic symptoms on which the diagnosis is based. For example, patients with schizophrenia show a range of cognitive deficits, particularly in measures of memory and executive functions (1), and differences in brain structure (2) and functional activity in regions thought to support such functions (3). Emphasis has been placed on the dorsolateral prefrontal cortex and its relation to working memory (3–6), a set of cognitive processes involved in actively maintaining and manipulating information in the mind in order to guide behavior (e.g., reference 7) and critical to high-level cognition (e.g., language, problem-solving, and reasoning). The relevance of working memory dysfunction to schizophrenia is evident in aspects of its symptoms, including formal thought disorder (8) and attentional disturbances (9–12), as might be predicted given the role of working memory in aspects of attention and goal-directed behavior. Accordingly, several behavioral studies have pointed to links between dorsolateral prefrontal cortex dysfunction, working memory, and cognitive disorganization, reflected in symptoms of poor attention and conceptual disorganization (10, 11, 13, 14).
The dorsolateral prefrontal cortex has been implicated in both working memory and the pathophysiology of schizophrenia. Evidence for the role of the dorsolateral prefrontal cortex in working memory comes from a variety of clinical and experimental domains, including human neuropsychological and cognitive psychological studies (7), brain lesion and functional brain imaging studies (15–17), and lesion and physiological studies in nonhuman primates (6, 18). Evidence for a role of the dorsolateral prefrontal cortex in the pathophysiology of schizophrenia also comes from a variety of domains. For example, patients typically show deficits on tests sensitive to frontal lobe damage, such as the Wisconsin Card Sorting Task (19), and on the Stroop (11) and delayed-response tasks (20, 21). Studies using brain imaging techniques have also demonstrated structural (2) and resting (22) functional abnormalities of the dorsolateral prefrontal cortex. Resting studies of brain activity have yielded mixed findings regarding the dorsolateral prefrontal cortex and schizophrenia, but more recent studies using functional neuroimaging methods coupled with neuropsychological or cognitive psychological tasks (4, 23, 24) have more reliably demonstrated evidence of dorsolateral prefrontal cortex dysfunction in subjects with schizophrenia.
Although considerable evidence implicates the dorsolateral prefrontal cortex in the pathophysiology of schizophrenia, a direct link between working memory dysfunction and deficits in brain activity remains elusive. This is, in part, because a number of functional neuroimaging studies of schizophrenia that ostensibly focus on working memory suffer from several important limitations.
First, much of the research has used experimental tasks such as the Wisconsin Card Sorting Task because of their sensitivity to frontal lobe function in traditional neuropsychological testing. Although these tasks clearly tap working memory, they engage other cognitive processes (e.g., learning, reinforcement) in addition to those typically considered central to working memory. The use of such tasks has been an important step in identifying specific cognitive processes and brain regions that may be disturbed in schizophrenia, but their complexity makes it difficult to disentangle working memory from other cognitive processes, thereby making it difficult to determine links between working memory deficits and prefrontal dysfunction in schizophrenia. The use of such complex tasks also makes it difficult to choose appropriate control tasks for use in “subtractive” image analysis methodology, further complicating inferences that can be drawn regarding working memory deficits and brain activity (25).
A second issue concerns the interpretation of activation differences in the face of group differences in task performance. It has been argued that, since patients with schizophrenia generally perform poorly on many cognitive tasks (26), hypofrontality or other abnormal task-evoked brain activity reflects a generalized secondary phenomenon or deficit, such as inattention or lack of engagement, and that such activation differences are merely “artifacts” of these nonspecific factors (27, 28) (compare with reference 29).
The primary aim of the present study was to build on previous findings and begin addressing these limitations by exploiting the advantages of functional magnetic resonance imaging (fMRI) and parametrically manipulating working memory load. By varying working memory load in a graded fashion, it becomes possible to selectively identify the brain circuitry supporting working memory in healthy subjects in a “dose-response” fashion. Thus, we can evaluate dysfunction within this circuitry in patients with schizophrenia by assessing neural activity at multiple levels of task demand and behavioral performance. Parametric manipulation has been shown to evoke graded changes in neural activity associated with working memory (15, 16, 30) and, more recently, has been shown to differentiate patients with schizophrenia from healthy subjects during both working memory (31) and retrieval from long-term memory (32).
We acquired fMRI data while patients with schizophrenia and healthy comparison subjects performed several levels of the “n-back” task, which we (15, 16) and others (30) have shown both systematically manipulates behavioral performance associated with verbal working memory and activates the cortical circuitry underlying verbal working memory, including the dorsolateral prefrontal cortex. We predicted that if working memory and dorsolateral prefrontal cortex dysfunction are functionally linked, patients and healthy comparison subjects would differ most in dorsolateral prefrontal cortex activation when they also differed in task performance. In contrast, we predicted that brain regions supporting other aspects of task performance that are not critical to working memory function (e.g., stimulus encoding or response execution) would not differentiate the groups, providing a critical “internal activation standard” (33). Finally, we predicted that dorsolateral prefrontal cortex dysfunction would correlate selectively with cognitive disorganization and that regions not showing activation deficits related to working memory would not show such a relationship.
Method
Participants
Participants were 17 clinically stable outpatients diagnosed with schizophrenia and 16 demographically matched healthy comparison subjects recruited from the community (Table 1). Eleven of the 17 patients and 10 of the 16 comparison subjects were men; six patients and six comparison subjects were women. To reduce the heterogeneity of the patient group, only patients who had been maintained on fixed doses of conventional injectable neuroleptics for at least 2 months before the study were included, and most participated within 5 days before the end of their injection cycle. Medical record review and structured clinical interview (with the Structured Clinical Interview for DSM-IV [SCID] [35]), conducted by a Ph.D.-level clinical psychologist (W.M.P.) and/or a trained research assistant, confirmed DSM-IV diagnoses of schizophrenia; absence of psychiatric symptoms in comparison subjects was also confirmed by the SCID.
The patients’ clinical state was assessed by using the Positive and Negative Syndrome Scale (34) on the day of testing. Ratings were completed either by a Ph.D.-level clinical psychologist (W.M.P.) or a trained researcher who regularly participated in training and reliability sessions. Symptoms were grouped into three “syndromes” according to the three factors suggested by Liddle (36)—reality distortion, poverty, and disorganization (Table 1). Intraclass correlations (37) established for interrater reliability during reliability sessions were 0.95, 0.95, and 0.94 for reality distortion, poverty, and disorganization, respectively.
All participants were right-handed, and none had a history of brain trauma, seizure disorder, ECT, mental retardation, or substance abuse or dependence within the past 6 months. All participants were paid for their participation. Before experimental sessions, patients were acclimated in an MRI simulator for at least 15 minutes, and all participants practiced the tasks until it was evident that they understood the instructions. Practice also served to reduce the influence of task-strategy development on brain activity. After complete description of the study, written informed consent was obtained from all participating subjects.
Tasks
Participants performed a sequential-letter memory task—the n-back task (15, 16). This task parametrically varied working memory load—0, 1, and 2 items for patients and 0, 1, 2, and 3 items for comparison subjects. Patients were not subjected to the 3-back task to minimize scanner time and reduce potential movement artifact. In the 0-back condition, the target was any letter that matched a prespecified letter (e.g., “X”). In the 1-back condition, the target was any letter that was identical to the one immediately preceding it (i.e., one trial back). In the 2-back and 3-back conditions, the target was any letter that was identical to the one present two and three trials back, respectively. Stimulus encoding and response demands are constant across conditions; only the requirements to maintain and update increasingly greater amounts of information at higher loads differ.
Pseudorandom sequences of single consonants were presented centrally on a visual display mounted in the scanner (500-msec duration, 3500-msec interstimulus interval). Subjects responded with a dominant-hand button press to each stimulus, pressing one button to targets (33% of trials) and another to nontargets. In each trial block, a number of stimuli were nontarget repeats that were included as foils (e.g., 1-back repeats in the 2-back task). Conditions were run in blocks of 72 seconds (18 stimuli) during which scans were acquired, with six blocks for each load condition. Order of task conditions was randomized across subjects.
Image Acquisition
Scanning took place in a conventional 1.5-T GE Signa whole-body scanner, and a standard radiofrequency head coil was used. Functional images were acquired in the axial plane by using a two-interleave T2*-weighted spiral-scan pulse sequence (TR=2000 msec, TE=35 msec, flip angle=80°, field of view=24 cm) (38) and were composed of isotropic voxels (3.75 mm3) acquired at 26 contiguous locations parallel to the anterior commissure-posterior commissure line. Scan acquisition was time-locked to each stimulus onset, and the duration of each scan spanned the entire 4-second duration of each trial. The first three trials of each block were discarded to allow for “loading” of working memory at the outset of the task. Structural images were obtained with a standard T1-weighted pulse sequence before functional scanning.
Task Performance Analysis
Reaction time and signal detection measures (39)—d′ (sensitivity) and beta (response criterion)—characterized the pattern of behavioral responding, which is not adequately reflected in overall accuracy. To test the a priori hypothesis that patients would perform worse than comparison subjects only at higher load levels, planned one-tailed, between-group, pairwise comparisons were made at each working memory level.
Image Analysis
Following reconstruction, images were normalized to a common mean and movement-corrected by using six-parameter automated image registration (40). Each subject’s structural images were then coregistered to a common reference by using 12-parameter automated image registration and smoothed by using a three-dimensional Gaussian filter (8-mm full width at half maximum) to accommodate between-subject differences in brain anatomy. Imaging data were analyzed with voxel-wise, mixed-model, two-group by three-load analyses of variance (ANOVAs) (subject=random factor). Follow-up contrasts of signal intensity in statistically identified regions were made by using between-group tests of linear and quadratic trend. A significance level of p<0.005 and an 8-voxel three-dimensional contiguity (41) were used as thresholds for statistical maps. Anatomical localization of suprathreshold activity was determined by overlaying activation maps onto the reference structural image and transforming them into standard reporting coordinates (42) by using AFNI software (43).
A potential criticism of fMRI studies in schizophrenia is that increased movement among patients creates artifacts that impair detection of cortical activation. This seems unlikely in the present study because significant differences in activation were limited to specific cortical regions and a specific task condition. Nevertheless, we evaluated this possibility by analyzing each of the six incremental estimated movement measures using group-by-load ANOVAs. A main effect of group emerged for the roll measure (F=6.96, df=1, 31, p<0.02). Consequently, we conducted two additional pixel-wise group-by-load ANOVAs on the fMRI data: one excluded two patients who contributed most to group differences in movement; the second retained all subjects but excluded images during and immediately before and after any movement parameter exceeded two standard deviations above the mean of any measure for comparison subjects. No significant group differences remained in the movement measures, and image analyses for both movement mitigation approaches yielded a pattern of statistical results that was identical to those already described.
Results
Task Performance
Neither d′ nor beta (Table 2) significantly differentiated the groups at the 0-back and 1-back loads, but both measures were significantly lower in patients than comparison subjects at the 2-back load (t≥1.88, df=31, p<0.04). Thus, patients were less able than comparison subjects to detect targets accurately and were more willing to respond to stimuli as targets at the highest load. Also, the two groups exhibited an equivalent number of responses overall in that they showed an equal proportion of “no responses” at all load levels. Finally, reaction times were significantly longer for patients than comparison subjects at the 0-back level (t=2.25, df=31, p<0.02), but the groups did not differ at the 1-back or 2-back levels.
Imaging Data
Voxel-wise group-by-load ANOVAs (Table 3) revealed significant load main effects in a network of regions previously shown to be engaged by verbally mediated working memory (Figure 1). All load main effects reflected monotonically increasing or decreasing activity; only increases are discussed here. The main effect of group revealed that activity in the subgenual region of the anterior cingulate gyrus was greater in patients than comparison subjects, but not as a function of load. More importantly, the predicted group-by-load interaction emerged in the dorsolateral prefrontal cortex (Figure 1). Compared with healthy subjects, patients with schizophrenia showed a negligible increase in signal intensity in the right dorsolateral prefrontal cortex at the 2-back compared with the 0-back and 1-back loads, but both groups showed equivalent increases from the 0-back to 1-back loads. Analysis of signal intensity within the right dorsolateral prefrontal cortex cluster yielded a significant interaction of group with both the linear (t=3.67, df=31, p<0.001) and quadratic (t=2.57, df=31, p<0.02) trends over load.
A second potential criticism is that poorer task performance by patients confounds interpretation of activation differences (44, 45); that is, patients may not activate the dorsolateral prefrontal cortex because they are not performing the task rather than because of any intrinsic dorsolateral prefrontal cortex dysfunction. The finding that the two groups did not differ in the number of no-response trials argues against this possibility.
Relationship Between Dorsolateral Prefrontal Cortex Activity and Task Performance
Correlations for each group separately between prefrontal cortex activity and measures of task performance, collapsed across working memory load levels, yielded a significant positive relationship with beta for the comparison subjects (r=0.53, df=15, p<0.04), but not the patients (r=0.12, df=16, p>0.60). This finding suggests that a more stringent response criterion was associated with increased prefrontal cortex signal intensity in the healthy subjects but not in the patients.
To demonstrate a link between dorsolateral prefrontal cortex dysfunction and target-detection sensitivity (d′), participants were grouped according to whether or not they exhibited increased right dorsolateral prefrontal cortex activity in the 2-back versus the 0-back condition. The change in d′ across these levels was then assessed by using a one-tailed t test. No comparison subject showed less activity in the 2-back than in the 0-back condition; five of the 17 patients did. Patients with less activity at the 2-back than the 0-back load showed significantly greater d′ decrement (–1.78) than patients who showed activity increases from the 0-back to the 2-back load (–0.99) (t=1.73, df=15, p=0.05).
The fMRI data were also analyzed after matching the groups on behavioral performance (d′)—0-back, 1-back, and 3-back levels for comparison subjects and 0-back, 1-back, and 2-back levels for patients. Under these conditions, no brain regions showed a significant group-by-load interaction at p<0.005 or at a more liberal threshold of p<0.01, providing additional evidence that group differences in task performance were associated with the observed group differences in dorsolateral prefrontal cortex activity.
Relationship Between Dorsolateral Prefrontal Cortex Activity and Symptoms
Correlation analyses conducted between the three symptom syndromes and fMRI signal change from 0-back to 2-back load levels in the right dorsolateral prefrontal cortex yielded a significant correlation only for disorganization (r=–0.74, df=16, p<0.0007) (Figure 2): increased disorganization was associated with decreased signal intensity change. This relationship was specific to the dorsolateral prefrontal cortex and disorganization; correlations between each of the three symptom syndromes and signal intensity change in supplementary motor and Broca’s areas were not significant.
Discussion
Medicated patients with schizophrenia, compared with healthy subjects, failed to exhibit task-related activity increases in the dorsolateral prefrontal cortex at the highest level of working memory load, consistent with the hypothesis of working-memory-related dorsolateral prefrontal cortex dysfunction in schizophrenia. Additionally, the reduced dorsolateral prefrontal cortex activation was associated with both poorer task performance and cognitive disorganization symptoms and emerged only at a level of working memory load that distinguished patients from healthy subjects on task performance.
These results complement previous findings of dorsolateral prefrontal cortex dysfunction using the n-back task (4, 31, 41). However, the present study employed a parametric approach with larger samples and found significant differences between groups in brain activity only when behavioral performance distinguished between the groups. The difference in dorsolateral prefrontal cortex activity persisted after we controlled for differences that might arise from movement artifact and was not sustained after groups were matched on task performance, providing additional support for a relationship between dorsolateral prefrontal cortex activity and working memory deficits.
Of particular note is the finding that significant load-related group differences were observed only in the dorsolateral prefrontal cortex and not in other regions typically engaged during verbally mediated working memory, namely, Broca’s area and the supplementary motor and posterior parietal cortices. This suggests that not all of the circuitry critical to verbal working memory is dysfunctional in patients, demonstrates some degree of specificity for dorsolateral prefrontal cortex dysfunction, and provides a critical “internal activation standard” (33). Further, the fact that the two groups did not differ on overall frequency of behavioral responding, even at the highest level of working memory load, minimizes the possibility that lack of behavioral engagement underlies the group differences in prefrontal activation. Given the entirety of findings, these results implicate a strong link between dorsolateral prefrontal cortex dysfunction and working memory deficits in conventionally medicated patients with schizophrenia. They also point to an association between cognitive disorganization and dorsolateral prefrontal cortex dysfunction.
What might be the particular aspect of working memory subserved by the dorsolateral prefrontal cortex and deficient in patients with schizophrenia? According to an influential theory (7), working memory comprises three dissociable components subserved by different but interacting cortical regions: a modality-specific buffer for storage of phonological codes (subserved largely by parietal regions), rehearsal processes to refresh these codes (subserved largely by the supplemental motor and Broca’s areas), and a set of executive or associative processes to manipulate the buffer’s contents in the service of higher cognitive functions (subserved by the dorsolateral prefrontal cortex). In view of this model, the absence of group differences in parietal and Broca’s/supplementary motor area activation suggests that pure storage and rehearsal components are not deficient in patients. Instead, more executive functions supported by the dorsolateral prefrontal cortex are implicated.
Detailed consideration of the pattern of task performance can further illuminate potential working memory component-processing deficits implied by the imaging data. Specifically, both the patient and comparison groups showed a higher proportion of false alarms on foil trials (i.e., nontarget repeats) compared with nonfoil (i.e., nonrepeat) trials and did not differ in error rates on target trials (Table 2). This suggests that patients adequately maintained trace representations of stimulus identity and points to a potential associative deficit—coding or maintaining sequential order information. The 2-back task requires maintaining the target-set n-back stimulus representations and coding and maintaining temporal position in the sequence, but the 0-back and 1-back tasks do not, since only a single letter must be kept in mind at any given time.
The groups showed equal levels of dorsolateral prefrontal cortex activity and performance at load levels that did not require temporal sequencing but differed in both when sequencing was required. This overall pattern is consistent with the hypothesis that maintenance and rehearsal are intact in schizophrenia but more associative processes (e.g., temporal coding) are dysfunctional, reflected in patients’ impaired ability to make appropriate use of the maintained information to guide behavior. This suggestion is also consistent with findings pointing to a role for the right dorsolateral prefrontal cortex in maintenance of not only the intrinsic content of stimuli but also the temporal order of their occurrence (46).
An alternative but not mutually exclusive interpretation of the observed dorsolateral prefrontal cortex deficit is that it reflects “capacity constraints.” That is, as working memory load increases and more attentional resources are required to maintain and manipulate the contents of working memory, capacity limitations are approached and expressed in impaired performance (30, 31, 45). Thus, patients may reach capacity limitations at lower load levels than healthy subjects, manifest by an inability to enhance dorsolateral prefrontal cortex activity as the limit is approached or exceeded.
Recent observations using modern neuroanatomical techniques to examine postmortem brain tissue in schizophrenia (2, 47, 48) have confirmed the presence of dorsolateral prefrontal cortex abnormalities that may contribute to the dorsolateral prefrontal cortex dysfunction described here. Among these findings are observations of reduced cortical thickness and increased neuronal density, suggestive of a loss of neuropil in dorsolateral prefrontal cortex (2), and reduced thalamic innervation of dorsolateral prefrontal cortex layer-three pyramidal cells (47). In the light of our current finding of normal activation in the inferior frontal cortex of patients with schizophrenia, it is intriguing that a recent study (48) demonstrated that the increased cell density was specific to the dorsolateral prefrontal cortex (Brodmann’s area 9) and that normal cell densities were observed in Brodmann’s area 44. It is unclear whether these and other anatomical findings reflect primary disturbances in the intrinsic circuitry of the dorsolateral prefrontal cortex, changes secondary to other factors such as altered thalamocortical connectivity, or both. However, these neuroanatomical data converge with results such as those of the present study to suggest that dorsolateral prefrontal cortex dysfunction is a reliable finding and may manifest its behavioral expression in impaired working memory function and disorganization.
The results of the present study differ in some ways from those of several previous studies. First, at least one study (20) observed a relationship between negative symptoms and working memory. Importantly, patients in this study were unmedicated, but all of the patients in the present study and our previous behavioral studies implicating a link between working memory and disorganization were receiving maintenance doses of conventional antipsychotic medications. This discrepancy may suggest that measurement of negative symptoms in unmedicated patients provides a purer measure of negative symptoms than measurement in medicated patients and enhances the ability to detect relationships among working memory, the prefrontal cortex, and negative symptoms. Additionally, the relationship between working memory and negative symptoms observed by Carter et al. (20) was obtained by using a spatial (rather than verbal) working memory task. Clarification of the relationship between prefrontal cortex activity and symptoms clearly requires additional study.
Second, although a task analysis points to deficits in temporal coding, maintenance of sequential-order information, or both, a study that explicitly tapped these functions (49) did not prompt activation in the dorsolateral prefrontal cortex, revealing instead patient hypoactivity in Broca’s area. Among the critical task differences between these studies, however, is the constant requirement to update information over the course of a trial block in the present study, which was absent from the “span” tasks used by Stevens et al. (49) and which may account for the differences.
In summary, our findings point to a specific deficit in the right dorsolateral prefrontal cortex linked to working memory in patients with schizophrenia that is selectively associated with cognitive disorganization symptoms. Decomposition of task components suggests that dorsolateral prefrontal cortex dysfunction is associated with more executive aspects of working memory, such as coding of temporal order, rather than processes involved in maintenance of stimulus representations per se. Future studies using tasks designed to assess coding and maintenance of order information more systematically will be necessary to evaluate this hypothesis more thoroughly.
![]() |
![]() |
![]() |
Presented in part at the 38th annual meeting of the Society for Psychophysiological Research, Denver, Oct. 23–27, 1998; the Fourth International Conference on Functional Mapping of the Human Brain, Montreal, June 7–12, 1998; and the 54th annual meeting of the Society of Biological Psychiatry, Washington, D.C., May 12–16, 1999. Received Aug. 25, 2000; revision received Jan. 17, 2001; accepted Feb. 1, 2001. From the Departments of Clinical and Health Psychology and Psychiatry and McKnight Brain Institute, University of Florida; the Department of Psychiatry and the MR Research Center, University of Pittsburgh, Pittsburgh; the Department of Biomedical Engineering, University of Michigan, Ann Arbor; and the Department of Psychology, Princeton University, Princeton, N.J. Address reprint requests to Dr. Perlstein, Department of Clinical and Health Psychology, Health Science Center, P.O. Box 100165, University of Florida, Gainesville, FL 32610; [email protected] (e-mail). Supported by grants from the National Alliance for Research in Schizophrenia and Depression and the Scottish Rite Foundation for Schizophrenia Research (Dr. Perlstein) and by an NIMH Postdoctoral Fellowship in Clinical Research Training in Psychiatry at the University of Pittsburgh School of Medicine. The authors thank Fred W. Sabb and Brittany Lourea for their assistance in patient recruitment and data collection and management.
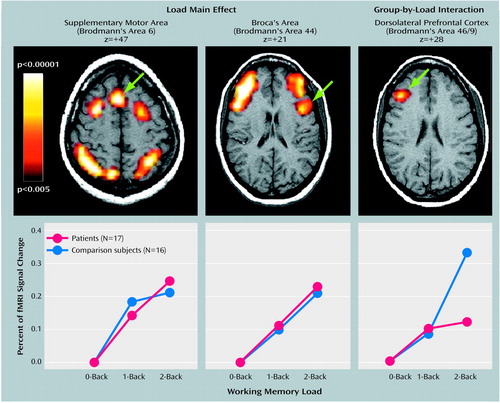
Figure 1. Brain Regions of Patients With Schizophrenia and Healthy Comparison Subjects in Which Activity Increased Monotonically as a Function of Working Memory Load, Group-by-Load Interaction in Dorsolateral Prefrontal Cortex, and Change in Signal Intensitya
aThe z values in the upper panel indicate the superior-inferior locations of the slices shown (according to the atlas of Talairach and Tournoux [42]) (left=right, right=left). The signal intensity values shown in the lower panel reflect the signal intensity averaged across all voxels in the statistically defined cluster. Working memory was assessed with a sequential-letter task. In the 0-back condition, the target was any letter that matched a prespecified letter. In the 1-back condition, the target was any letter that was identical to the one immediately preceding it. In the 2-back condition, the target was any letter identical to the one two trials back.
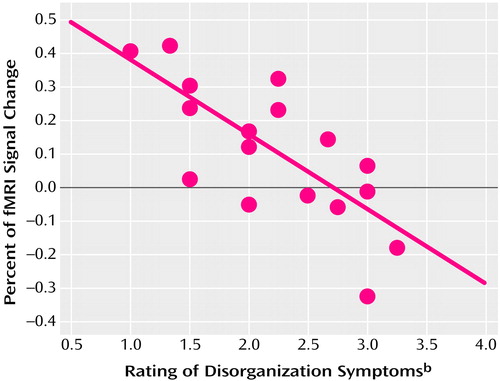
Figure 2. Relationship Between Disorganization Symptoms and Change in Signal Intensity in Right Dorsolateral Prefrontal Cortex as a Function of Working Memory Load for 17 Patients With Schizophreniaa
aWorking memory was assessed with a sequential-letter task. In the 0-back condition, the target was any letter that matched a prespecified letter. In the 1-back condition, the target was any letter that was identical to the one immediately preceding it. In the 2-back condition, the target was any letter identical to the one two trials back. The signal change shown here is the percent change of the 2-back from the 0-back condition.
bIncludes conceptual disorganization, mannerisms and posturing, difficulty abstracting, and poor attention from the Positive and Negative Syndrome Scale (34).
1. Blanchard JJ, Neale JM: The neuropsychological signature of schizophrenia: generalized or differential deficit? Am J Psychiatry 1994; 151:40-48Link, Google Scholar
2. Selemon LD, Goldman-Rakic PS: The reduced neuropil hypothesis: a circuit based model of schizophrenia. Biol Psychiatry 1999; 45:17-25Crossref, Medline, Google Scholar
3. Weinberger DR, Berman K, Zec R: Physiological dysfunction of dorsolateral prefrontal cortex in schizophrenia, I: regional cerebral blood flow evidence. Arch Gen Psychiatry 1986; 43:114-124Crossref, Medline, Google Scholar
4. Carter CS, Perlstein W, Ganguli R, Brar J, Mintun M, Cohen JD: Functional hypofrontality and working memory dysfunction in schizophrenia. Am J Psychiatry 1998; 155:1285-1287Google Scholar
5. Cohen JD, Servan-Schreiber D: Context, cortex, and dopamine: a connectionist approach to behavior and biology in schizophrenia. Psychol Rev 1992; 99:45-77Crossref, Medline, Google Scholar
6. Goldman-Rakic PS: Circuitry of primate prefrontal cortex and regulation of behavior by representational memory, in Handbook of Physiology: The Nervous System. Edited by Plum F. Bethesda, Md, American Physiological Society, 1987, pp 373-414Google Scholar
7. Baddeley AD: Working Memory. Oxford, UK, Oxford University Press, 1986Google Scholar
8. Barch DM, Berenbaum H: Language production and thought disorder in schizophrenia. J Abnorm Psychol 1996; 105:81-88Crossref, Medline, Google Scholar
9. Cohen JD, Barch DM, Servan-Schreiber D: Context processing deficits in schizophrenia: converging evidence from three theoretically motivated cognitive tasks. J Abnorm Psychol 1999; 108:120-133Crossref, Medline, Google Scholar
10. Servan-Schreiber D, Cohen JD, Stengard S: Schizophrenic deficits in the processing of context: an empirical test of a theoretical model. Arch Gen Psychiatry 1996; 53:1105-1112Google Scholar
11. Perlstein WM, Carter CS, Barch DM, Baird JW: The Stroop task and attention deficits in schizophrenia: a critical evaluation of card and single-trial Stroop methodologies. Neuropsychology 1998; 12:414-425Crossref, Medline, Google Scholar
12. Barch DM, Carter CS: Selective attention in schizophrenia: relationship to verbal working memory. Schizophr Res 1998; 33:53-61Crossref, Medline, Google Scholar
13. Barch DM, Carter CS, Perlstein WM, Baird JD, Cohen JD: Increased Stroop facilitation is not due to enhanced spreading activation in schizophrenia. Schizophr Res 1999; 39:51-64Crossref, Medline, Google Scholar
14. Barch DM, Carter CS, Hachten PC, Usher M, Cohen JD: The benefits of distractibility: mechanisms underlying increased Stroop effects in schizophrenia. Schizophr Bull 1999; 25:749-762Crossref, Medline, Google Scholar
15. Braver TS, Cohen JD, Nystrom LE, Jonides J, Smith EE, Noll DC: A parametric study of prefrontal cortex involvement in human working memory. Neuroimage 1997; 5:49-62Crossref, Medline, Google Scholar
16. Cohen JD, Perlstein WM, Braver TS, Nystrom LE, Noll DC, Jonides J, Smith EE: Temporal dynamics of brain activation during a working memory task. Nature 1997; 386:604-608Crossref, Medline, Google Scholar
17. Courtney SM, Ungerleider LG, Kell K, Haxby JV: Transient and sustained activity in a distributed neural system for human working memory. Nature 1997; 386:608-611Crossref, Medline, Google Scholar
18. Fuster JM: The Prefrontal Cortex: Anatomy, Physiology and Neuropsychology of the Frontal Lobe, 3rd ed. New York, Raven Press, 1997Google Scholar
19. Van der Does AW, Van den Bosch RJ: What determines Wisconsin Card Sorting performance in schizophrenia? Clin Psychol Rev 1992; 12:567-583Crossref, Google Scholar
20. Carter CS, Robertson LC, Nordahl TE, Kraft L, Chaderjian M, Oshora-Celaya L: Spatial working memory deficits and their relationship to negative symptoms in unmedicated schizophrenic patients. Biol Psychiatry 1996; 40:930-932Crossref, Medline, Google Scholar
21. Park S, Holzman PS: Schizophrenics show spatial working memory deficits. Arch Gen Psychiatry 1992; 49:975-982Crossref, Medline, Google Scholar
22. Ingvar DH, Franzen G: Abnormalities of cerebral blood flow distribution in patients with chronic schizophrenia. Acta Psychiatr Scand 1974; 50:425-462Crossref, Medline, Google Scholar
23. Andreasen NC, Rezai K, Alliger R, Swayze VW II, Flaum M, Kirchner P, Cohen G, O’Leary DS: Hypofrontality in neuroleptic-naive patients and in patients with chronic schizophrenia: assessment with Xenon 133 single-photon emission computed tomography and the Tower of London. Arch Gen Psychiatry 1992; 49:943-958Crossref, Medline, Google Scholar
24. Taylor SF: Cerebral blood flow activation and functional lesions in schizophrenia. Schizophr Res 1996; 19:129-140Crossref, Medline, Google Scholar
25. Friston KJ, Price CJ, Fletcher P, Moore C, Frackowiak RSJ, Dolan RJ: The trouble with cognitive subtraction. Neuroimage 1996; 4:97-104Crossref, Medline, Google Scholar
26. Chapman LJ, Chapman JP: Strategies for resolving the heterogeneity of schizophrenics and their relatives using cognitive measures. J Abnorm Psychol 1989; 98:357-366Crossref, Medline, Google Scholar
27. Ebmeier KP, Lawrie SM, Blackwood DHR, Johnstone EC, Goodwin GM: Hypofrontality revisited: a high resolution single photon emission computed tomography study in schizophrenia. J Neurol Neurosurg Psychiatry 1995; 58:452-456Crossref, Medline, Google Scholar
28. Gur RC, Gur RE: Hypofrontality in schizophrenia: RIP. Lancet 1995; 345:1383-1384Google Scholar
29. Weinberger DR, Berman KF: Prefrontal function in schizophrenia: confounds and controversies. Phil Trans R Soc Lond B 1996; 351:1495-1503Google Scholar
30. Callicott JH, Mattay VS, Bertolino A, Finn K, Coppola R, Frank JA, Goldberg TE, Weinberger DR: Physiological characteristics of capacity constraint in working memory as revealed by functional MRI. Cereb Cortex 1999; 9:20-26Crossref, Medline, Google Scholar
31. Callicott JH, Bertolino A, Mattay VS, Langheim FJP, Duyn J, Coppola R, Goldberg TE, Weinberger DR: Physiological dysfunction of the dorsolateral prefrontal cortex in schizophrenia revisited. Cereb Cortex 2000; 10:1078-1092Google Scholar
32. Fletcher PC, McKenna PJ, Frith CD, Grasby PM, Friston KJ, Dolan RJ: Brain activations in schizophrenia during a graded memory task studied with functional neuroimaging. Arch Gen Psychiatry 1998; 55:1001-1008Google Scholar
33. Weinberger DR, Mattay V, Callicott J, Kotrla K, Santha A, van Gelderen P, Duyn J, Moonen C, Frank J: fMRI applications in schizophrenia research. Neuroimage 1996; 4(3, part 3):S118-S126Google Scholar
34. Kay SR, Fiszbein A, Opler LA: The Positive and Negative Syndrome Scale (PANSS) for schizophrenia. Schizophr Bull 1987; 13:261-276Crossref, Medline, Google Scholar
35. First MB, Spitzer RL, Gibbon M, Williams JBW: Structured Clinical Interview for DSM-IV Axis I Disorders (SCID). New York, New York State Psychiatric Institute, Biometrics Research, 1995Google Scholar
36. Liddle PF: Syndromes of chronic schizophrenia: a re-examination of the positive-negative dichotomy. Br J Psychiatry 1987; 151:145-151Crossref, Medline, Google Scholar
37. Shrout PE, Fleiss JL: Intraclass correlations: uses in assessing rater reliability. Psychol Bull 1979; 86:420-428Crossref, Medline, Google Scholar
38. Noll DC, Cohen JD, Meyer CH, Schneider W: Spiral K-space MR imaging of cortical activity. J Magn Reson Imaging 1995; 5:49-56Crossref, Medline, Google Scholar
39. Green DM, Swets JA: Signal Detection Theory and Psychophysics. New York, Krieger, 1966Google Scholar
40. Woods RP, Cherry SR, Maziotta JC: Rapid automation algorithm for aligning and reslicing PET images. J Comput Assist Tomogr 1992; 16:620-633Crossref, Medline, Google Scholar
41. Forman SD, Cohen JD, Fitzgerald M, Eddy WF, Mintun MA, Noll DC: Improved assessment of significant activation in functional magnetic resonance imaging (fMRI): use of a cluster-size threshold. Magn Reson Med 1995; 33:636-647Crossref, Medline, Google Scholar
42. Talairach J, Tournoux P: Co-Planar Stereotaxic Atlas of the Human Brain: Three-Dimensional Proportional System. Stuttgart, Germany, Georg Thieme, 1988Google Scholar
43. Cox RW: AFNI: software for analysis and visualization of functional magnetic resonance neuroimages. Comput Biomed Res 1996; 29:162-173Crossref, Medline, Google Scholar
44. Callicott JH, Ramsey NF, Tallent K, Bertolino A, Knable MB, Coppola R, Goldberg T, van Gelderen P, Mattay VS, Frank JA, Moonen CTW, Weinberger DR: Functional magnetic resonance imaging brain mapping in psychiatry: methodological issues illustrated in a study of working memory in schizophrenia. Neuropsychopharmacology 1998; 18:186-195Crossref, Medline, Google Scholar
45. Manoach DS, Press DZ, Thangaraj V, Searl MM, Goff DC, Halpern E, Saper CB, Warach S: Schizophrenic subjects activate dorsolateral prefrontal cortex during a working memory task, as measure by fMRI. Biol Psychiatry 1999; 45:1128-1137Google Scholar
46. Smith EE, Jonides J: Neuroimaging analyses of human working memory. Proc Natl Acad Sci USA 1998; 95:12061-12068Google Scholar
47. Akil M, Pierri JN, Whitehead RE, Edgar CL, Mohila C, Sampson AR, Lewis DA: Lamina-specific alterations in the dopamine innervation of the prefrontal cortex in schizophrenic subjects. Am J Psychiatry 1999; 156:1580-1589Google Scholar
48. Selemon LD, Mrzljak J, Kleinman JE, Herman MM, Goldman-Rakic PS: Selective neuropil deficit in prefrontal area 9, not Broca’s area 44, in a new cohort of schizophrenic brains. Abstracts of the Society for Neuroscience 1999; 25:574Google Scholar
49. Stevens AA, Goldman-Rakic PS, Gore JC, Fulbright RK, Wexler BE: Cortical dysfunction in schizophrenia during auditory word and tone working memory demonstrated by functional magnetic resonance imaging. Arch Gen Psychiatry 1998; 55:1097-1103Google Scholar