Association of Enhanced Limbic Response to Threat With Decreased Cortical Facial Recognition Memory Response in Schizophrenia
Abstract
Objective
Recognition memory of faces is impaired in patients with schizophrenia, as is the neural processing of threat-related signals, but how these deficits interact to produce symptoms is unclear. The authors used an affective face recognition paradigm to examine possible interactions between cognitive and affective neural systems in schizophrenia.
Method
Blood-oxygen-level-dependent response was examined by means of functional magnetic resonance imaging (3 Tesla) in healthy comparison subjects (N=21) and in patients with schizophrenia (N=12) or schizoaffective disorder, depressed type (N=4), during a two-choice recognition task that used images of human faces. Each target face, previously displayed with a threatening or nonthreatening affect, was displayed with neutral affect. Responses to successful recognition and responses to the effect of previously threatening versus nonthreatening affect were evaluated, and correlations with symptom severity (total Brief Psychiatric Rating Scale score) were examined. Functional connectivity analyses examined the relationship between activation in the amygdala and cortical regions involved in recognition memory.
Results
Patients performed the task more slowly than healthy comparison subjects. Comparison subjects recruited the expected cortical regions to a greater degree than patients, and patients with more severe symptoms demonstrated proportionally less recruitment. Increased symptoms were also correlated with augmented amygdala and orbitofrontal cortex response to threatening faces. Comparison subjects exhibited a negative correlation between activity in the amygdala and cortical regions involved in cognition, while patients showed weakening of this relationship.
Conclusion
Increased symptoms were related to an enhanced threat response in limbic regions and a diminished recognition memory response in cortical regions, supporting a link between these two brain systems that are often examined in isolation. This finding suggests that abnormal processing of threat-related signals in the environment may exacerbate cognitive impairment in schizophrenia.
Patients with schizophrenia show impairments in cognition (1, 2) and emotion processing (3, 4) that affect functional outcomes. Despite some discussion of relationships between these deficits (5), few studies have investigated the interaction between cognitive and emotional processing demands in schizophrenia. Studies in healthy people suggest that cognitive functioning and emotional processing are intimately related. For example, one study (6) reported that responses to threatening stimuli impede performance on cognitive tasks. Functional magnetic resonance imaging (fMRI) studies have identified a potential neural basis for this interaction, demonstrating that increased responses to threat in ventral limbic regions inhibit activation in cortical regions involved in cognition (7, 8).
Recognition memory is prominently affected in schizophrenia (1, 9) and is related to dysregulation of multiple brain regions (10–13). In a series of experiments in healthy individuals, Buckner and colleagues demonstrated that medial and lateral parietal regions are the most reliably recruited brain regions by recognition memory tasks (14, 15). These regions are functionally connected with the hippocampus (16), and abnormalities within this network correlate with symptoms of dementia (17). Notwithstanding the traditional focus on fronto-temporal abnormalities in schizophrenia, parietal regions merit increasing scrutiny, since parietal abnormalities in the "default network" in schizophrenia have been found (18). In contrast, emotional paradigms in schizophrenia consistently demonstrate altered recruitment of limbic regions, including the amygdala and orbitofrontal cortex (19–21).
In the present study, we examined the interaction between emotion processing and recognition memory systems in schizophrenia using an affective face recognition memory paradigm. Subjects made a simple, binary choice ("old" versus "new") regarding previously viewed (target) or novel (foil) faces, all shown with a neutral expression. However, the target faces were initially displayed with either intense threatening affect (angry or fearful) or nonthreatening affect (happy or sad). Thus, the task had the advantage of integrating both cognitive (old versus new) and affective (threat versus nonthreat) components. In healthy subjects, this task recruits frontoparietal regions involved in recognition memory and also produces an amygdala and orbitofrontal cortex response to faces initially seen with a threatening expression (22).
We hypothesized that the symptoms of schizophrenia might reflect an imbalance between emotional and cognitive responses, such that as symptoms become more severe, limbic responses predominate at the expense of cortical recruitment by cognitive demands. Specifically, we examined brain responses using fMRI to test three predictions. First, we expected that greater symptom severity would be correlated with diminished recruitment of parietal regions known to be involved in recognition memory (14, 23, 24). Second, as suggested by previous investigations (21, 25), we expected that response to threat in limbic regions would be correlated with symptom severity. Third, we used a functional connectivity analysis to test for disruption of the relationship between parietal regions involved in recognition memory and limbic regions involved in threat response.
Method
Subjects
The study sample consisted of 16 patients (men: 60%) with schizophrenia (N=12) or schizoaffective disorder, depressed type (N=4), and 21 healthy comparison subjects (men: 48%). The patient and comparison groups were demographically balanced (see the data supplement accompanying the online version of this article). After a complete description of the study, subjects provided written, informed consent. Standardized assessment followed previously reported procedures (see the online data supplement). No subject met criteria for a current depressive episode. Fourteen patients were receiving treatment with antipsychotics (first generation [N=1]; second generation [N=12]; first and second generation combined [N=1]), and two patients were unmedicated. The average daily chlorpromazine equivalent dose was 290 mg (SD=254).
Task
The face recognition experiment was preceded by an emotion identification task (26–28) in which subjects viewed 30 faces displaying happy, sad, angry, fearful, or neutral affect and were asked to label the emotion displayed (Figure 1). Stimuli validation is described in detail elsewhere (29). In the face recognition experiment, 30 faces from the emotion identification phase (targets) as well as 30 novel faces (foils) were presented. Subjects made a simple, "old" versus "new" judgment using a two-button response keypad. All faces in the recognition experiment were displayed with neutral expressions, whereas target faces had been shown in the identification experiment with emotional expressions. However, target faces with a neutral affect were displayed with the same expression in both experiments, making neutral trials difficult to compare with other target faces, and therefore these trials were excluded from all analyses. Previous studies conducted in our laboratory (26, 27) have indicated the utility of dividing emotions by threat-relatedness, as suggested by Gray (30) and other investigators (31, 32). Target faces originally displayed with an angry or fearful affect were modeled together as "threat," and faces originally displayed with a happy or sad affect were modeled together as "nonthreat." There were six trials per emotion in the emotion identification experiment, yielding 12 threat and 12 nonthreat trials.

aPanel A illustrates the encoding task. Subjects initially performed an emotion identification task in which they identified the facial affect displayed. Five emotional labels were available, including two of nonthreatening affect (happy and sad) and two of threatening affect (angry and fearful) as well as neutral affect. Subjects were not instructed to remember the faces displayed. Panel B illustrates the face recognition task. Following the affect identification task, subjects were asked to make a forced-choice facial recognition judgment. Thirty faces from the affect identification task (targets) and 30 novel faces (foils) were displayed for 2 seconds each. Subjects made a simple "old" versus "new" judgment as to whether the face had been previously displayed in the affect identification task. Faces were separated by a variable interval (0–12 seconds) in which a crosshair fixation point was displayed on a complex background (degraded face). The task duration was 4 minutes, 16 seconds. Emotion identification and face recognition experiments were separated by a 10-minute diffusion tensor imaging acquisition.
Performance Analysis
Percent correct and median response time were calculated by group (patients versus healthy comparison subjects), prior facial affect (threat versus nonthreat), and face recognition response (correct versus incorrect). Differences in accuracy and response time among the conditions were evaluated with a 2×2×2 (group-by-affect-by-response) repeated-measures analysis of variance (ANOVA) implemented in STATA (StataCorp., LP, College Station, Tex.). Response bias was evaluated by calculating "Br" (bias measure) (33), which provided an independent measure of the overall tendency of subjects to make "old" or "new" responses regardless of accuracy: Br=False Alarms/[1–(Hit–False Alarms)]–0.5. Positive values corresponded to a liberal familiarity bias (i.e., more likely to judge a new item as old), whereas negative values corresponded to a conservative novelty bias (i.e., more likely to judge an old item as new). Association with symptoms was evaluated by correlating each patient's total Brief Psychiatric Rating Scale (BPRS) score with the accuracy and response time for all trials, as well as targets and foils, separately. Exploratory analyses of the influence of paranoia (as measured by the "suspiciousness" item in BPRS) and medication dosage (chlorpromazine equivalents) were conducted in a similar fashion (34). All performance analyses were evaluated at a p-value significance level of 0.05 (uncorrected).
Image Analysis
Functional MRI data were preprocessed and analyzed using FMRI Expert Analysis Tool, Version 5.9 (Functional MRI of the Brain Software Library, Oxford University, U.K.), with standard fMRI settings (see the online data supplement) (22). To study both recognition memory and prior facial affect, single-subject analyses were carried out separately using two general linear models. The first model examined recognition memory and considered the following four trial types as regressors of interest: correctly identifying a target face ("hit"), incorrectly identifying a target face as new ("miss"), correctly identifying a foil face ("correct rejection"), and incorrectly identifying a foil face as old ("false alarm"). Analysis of this model focused on the standard recognition memory contrast of hit versus correct rejection. The second model examined prior facial affect and included each original target emotion (happy, sad, anger, fear) separately as regressors of interest. The main contrast of interest in this model was threat (anger, fear) versus nonthreat (happy, sad). In this analysis, correct and incorrect trials were modeled together. Both general linear models included neutral trials, nonresponses, temporal derivatives of each variable, and six rigid-body movement parameters as covariates of no interest.
Within-group and between-group mixed-effects analyses were performed to conduct one- and two-sample t tests on subject-level whole-brain contrasts. For each contrast, a hypothesis-driven analysis within a priori regions of interest was followed by a whole-brain exploratory analysis. To examine individual differences in clinical severity, blood-oxygen-level-dependent response in the hit versus correct rejection and the threat versus nonthreat contrasts were correlated in separate voxelwise analyses, with the total BPRS score as an additional covariate of interest. Peak r values are reported for each significant correlation with total BPRS scores (but see Vul et al. [35]). In an exploratory analysis, we examined the influence of paranoia by adding the BPRS suspiciousness item (34) as an additional covariate. In order to contain type I error, correlations were evaluated only within a priori regions of interest. Individual items beyond paranoia were not evaluated. Finally, we investigated the effect of medication dosage by adding each patient's antipsychotic dose (chlorpromazine equivalent dose) as a covariate to each correlation analysis.
We corrected for multiple comparisons using AFNI's AlphaSim Monte Carlo simulations (developed by R.W. Cox, National Institutes of Health, Bethesda, Md.) at a z threshold of 2.33 and a probability of spatial extent at a p value <0.05. For display purposes, all figures were smoothed using a 6×6×6-mm kernel and thresholded at a p value <0.05 (uncorrected) using Mango Software (developed by J.L. Lancaster and J. Martinez, University of Texas Health Science Center, San Antonio, Tex.). Identified clusters were anatomically labeled using the Talairach Daemon database (36). Coordinates are reported in Montreal Neurological Institute coordinate space. Peak voxel percent signal change was plotted against the total BPRS score for each subject for the display of correlation scatter plots.
Regions of Interest
We identified parietal regions previously implicated in other studies of recognition memory (14, 15, 23, 24) as our a priori region of interest for the hit versus correct rejection analysis. This region of interest was constructed using a contiguous mask of regions from the Talairach Daemon Atlas (36). The large cortical mask (25,098 2×2×2 mm voxels) included the lateral parietal cortex (inferior parietal lobule, angular gyrus, and supramarginal gyrus) and medial parietal cortex (precuneus and posterior cingulate). For the threat versus nonthreat contrast, we identified the bilateral amygdala and orbitofrontal cortex as a priori regions of interest (22), anatomically defined using the Harvard-Oxford Subcortical Atlas, thresholded at 0. For both contrasts of interest, we followed the a priori analysis with an exploratory whole-brain analysis to identify significant effects outside the a priori regions of interest. As noted, correlation analyses were conducted for a priori regions of interest only.
Functional Connectivity Analysis
In order to examine interactions between limbic regions involved in emotional processing and cortical regions involved in recognition memory, we conducted a functional connectivity analysis using the methods described by Fox et al. (37 [also see references 16, 17]). To remove confounding sources of correlation, we included the following three regressors in addition to motion parameters in the model: mean whole-brain signal, mean signal within the lateral ventricles, and mean signal within a white matter region of interest. We examined correlations with a left amygdala "seed" region, defined anatomically using the Harvard-Oxford Subcortical Atlas, at a threshold of 0.75. Finally, in patients, we examined the relationship of connectivity to symptom severity (total BPRS score), paranoia, and medication dosage within the a priori parietal region of interest, using the aforementioned methods.
Results
Performance
Behavioral results are presented in Table 1. Target accuracy was limited by a conservative response bias, indicating that subjects were more likely to judge old target faces as new. Patients displayed a somewhat less conservative response bias relative to healthy comparison subjects, although there was not a significant group difference. As expected, both patients and healthy comparison subjects recognized previously nonthreatening faces more accurately (F=7.95, df=1, 35, p<0.01). Although there was no accuracy difference between groups, patients performed the task more slowly than comparison subjects (F=9.04, df=1, 35, p=0.005). Furthermore, symptom burden correlated with overall response time (r=0.56, p=0.01). This BPRS score-response time correlation was present for both targets (r=0.46, p=0.03) and foils (r=0.45, p=0.04). No other group differences, main effects, interactions, or significant correlations were present.
![]() |
Hit Versus Correct Rejection Analysis
As seen in Figure 2, healthy comparison subjects activated the expected parietal regions, including the posterior cingulate and the left inferior parietal lobule. The exploratory whole-brain analysis (see Table 1 in the online data supplement) implicated other regions beyond the parietal regions of interest, including the bilateral middle frontal gyrus and anterior cingulate. As in prior experiments (14, 38), the left inferior parietal lobule demonstrated the greatest response to hit trials, an intermediate response to miss or false alarm trials, and the least response to correct rejection trials. Patients activated the same network to a lesser extent. Group comparisons within the a priori parietal region of interest demonstrated that comparison subjects activated the posterior cingulate more than patients (maximum z: 3.23; voxels: 184; coordinates: –6, –40, 26). Whole-brain analysis revealed a significant group difference in the right middle frontal gyrus (maximum z: 3.52; voxels: 147; coordinates: 46, 24, 32).

aPanel A displays how healthy comparison subjects activate the expected frontoparietal memory network, including the lateral and medial parietal regions. Panel B shows left inferior parietal lobule response during a "hit" (correctly identifying a target face), "miss" (incorrectly identifying a target face as new), "correct rejection" (correctly identifying a foil face), and "false alarm" (incorrectly identifying a foil face as old). As in other studies, the inferior parietal lobule responds robustly to hits but not correct rejections, and misses and false alarms demonstrate an intermediate response. Panel C depicts the greater response in the posterior cingulate and the right middle frontal gyrus for the hit versus correct rejection seen in healthy comparison subjects relative to the patients. Panel D illustrates the inverse relationship between symptom severity and the hit versus correct rejection response in both lateral and medial parietal cortex.
Patients with more severe symptoms demonstrated diminished hit versus correct rejection response. There were significant total BPRS correlations (Figure 2) in the lateral parietal cortex (maximum z: –3.8; voxels: 305; coordinates: 52, –66, 34; peak r=–0.84) and posterior cingulate (maximum z: –3.03; voxels: 74; coordinates: 2, –22, 40; peak r=–0.69). No correlations were found with paranoia. Patients at higher medication doses demonstrated a diminished hit versus correct rejection response in the right precuneus (maximum z: –3.8; voxels: 77; coordinates: 22, –78, 44). The correlations of total BPRS score and hit versus correct rejection response remained significant when medication dose was considered in the same model.
Threat Versus Nonthreat Analysis
As seen in Figure 3, consistent with our previous finding (22) healthy comparison subjects showed significant threat versus nonthreat responses in the left amygdala (maximum z: 3.45; voxels: 54; coordinates: –30, –6, –28), right orbitofrontal cortex (maximum z: 3.22; voxels: 137; coordinates: 44, 56, –12), and a left lateral temporal region (see Table 2 in the online data supplement). In contrast, patients did not show significant threat versus nonthreat differences in either the a priori regions of interest or the exploratory whole-brain analysis. There were no group differences between patients and comparison subjects. A follow-up analysis separately examining responses to threatening and nonthreatening stimuli versus baseline responses also did not reveal group differences. Furthermore, when the four patients with schizoaffective disorder, depressed type, were excluded from the analysis, between-group results remained unchanged.

aPanel A illustrates the threat versus nonthreat response in the left amygdala and right orbitofrontal cortex of healthy comparison subjects. In patients, severity of symptoms correlates with increased response to threat in the right amygdala and the right orbitofrontal cortex.
In contrast to the effects seen for recognition memory, increased symptoms resulted in an augmented threat response. There was a significant correlation between threat versus nonthreat and the BPRS total score in the right amygdala (maximum z: 3.57; voxels: 125; coordinates: 30, 4, –14; peak r=0.87) and right orbitofrontal cortex (maximum z: 3.75; voxels: 242; coordinates: 32, 22, 2; peak r=0.79) as well as a subthreshold cluster present in the left amygdala (maximum z: 3.33; voxels: 46; coordinates: –18, –12, –16). Furthermore, paranoia was correlated with the threat versus nonthreat response in the right amygdala (maximum z: 3.68; voxels: 113; coordinates: 28, 12, –24). Medication dosage attenuated the threat versus nonthreat response in the left amygdala (maximum z: 3.35; voxels: 115; coordinates: –28, –8, –14) and left orbitofrontal cortex (maximum z: 3.24; voxels: 62; coordinates: –44, 24, 2). The correlations of the total BPRS score and threat versus nonthreat remained significant when paranoia and medication dose were both included in the same model.
Functional Connectivity Analysis
In healthy comparison subjects, amygdala activity was positively correlated with other limbic regions and negatively correlated with cortical regions, including parietal areas activated in the hit versus correct rejection analysis (Figure 4). Patients showed a similar pattern, but with stronger connectivity between the left amygdala and other ventral limbic regions, as well as a diminished negative correlation with cortical regions. A direct between-group comparison in the a priori parietal region of interest demonstrated that patients had increased connectivity (in the form of a diminished negative correlation) between the amygdala and right inferior parietal lobule (maximum z: 3.22; voxels: 97; coordinates: 50, –58, 40). In the whole-brain analysis, patients demonstrated increased connectivity in the right middle frontal gyrus as well as in multiple limbic regions, including the right orbitofrontal cortex, right insula, and midbrain (see Table 3 in the online data supplement). No symptom or medication-dose correlations were present.
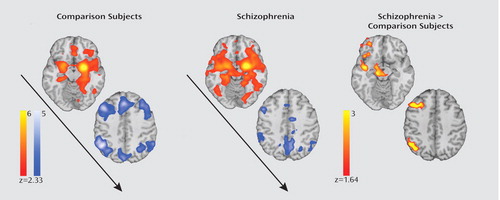
aImages on the left illustrate the positive correlation with activity in a left amygdala seed in ventral limbic regions and a negative correlation in cortical regions involved in cognition in healthy comparison subjects. Images in the middle depict the increased functional connectivity between the amygdala and other limbic regions as well as increased connectivity (reduced negative correlation) with cortical regions among the patients. The images on the right show how relative to comparison subjects, patients show significantly reduced anticorrelation between the left amygdala and the right middle frontal gyrus and right inferior parietal lobule as well as increased connectivity between the amygdala and the right orbitofrontal cortex, right insula, and midbrain.
Discussion
This study explored the sparsely investigated intersection between emotion and cognition in schizophrenia. To probe this connection, we investigated the effect of a prior exposure to an intense facial affect on neural activation during a subsequent face recognition task. There were three main findings. First, patients with schizophrenia showed impaired recruitment of regions involved in recognition memory, and the degree to which activation was reduced correlated with overall symptom severity. Second, symptom severity correlated with heightened amygdala and orbitofrontal cortex responses to previously threatening faces. Third, patients showed a weakening of the normally negatively correlated relationship between the amygdala and frontoparietal regions involved in cognition and also displayed increased amygdala connectivity with other limbic regions. These results have several implications as well as limitations.
Patients With Schizophrenia Show Impaired Brain Responses to Face Recognition
Behavioral deficits in facial recognition memory in schizophrenia have been demonstrated in the past (2). In the present study, we reported on potential neural substrates of this performance deficit, since patients showed reduced recruitment of parietal and frontal regions involved in recognition memory. Further, the degree to which recruitment was impaired correlated with symptom severity. Previous literature has emphasized frontotemporal abnormalities during recognition memory in schizophrenia (10–12). Our study provides evidence that parietal regions may also contribute to these deficits. We observed similar effects in the middle frontal gyrus, a region implicated in the cognitive control of recognition memory (24). Notably, the hippocampus is not reliably activated by the standard hit versus correct rejection contrast in recognition memory studies, which might reflect robust task-induced hippocampal activation regardless of recognition performance (14). Previous studies in schizophrenia have shown impaired recruitment of the hippocampus across the task (12), which was also seen in the present study.
Threat Response in Schizophrenia is Associated With Severity of Symptoms
Previous studies have shown abnormalities in affective threat processing in schizophrenia (19–21) and suggested that these abnormalities are symptom related (25). However, all prior studies gathered data during the display of threatening affect. In the present study, we examined patients' response to neutral faces that were previously displayed with a threatening expression. This difference is important, since facial affective memory is likely to play a role in the development and maintenance of psychotic symptoms (39). We found that greater response in the amygdala to prior threat was associated with both greater symptom burden and higher levels of paranoia, suggesting an emergence of symptoms with increased sensitivity to social signals of threat. Unlike studies examining direct displays of threat, we did not find group differences in amygdala responses, although this may be the result of lack of explicit attention to affect or to the heterogeneity of symptom-mediated threat response in patients.
The finding that increased symptoms both reduced activation during recognition memory in cortical regions and augmented response to threat in limbic regions suggests a link between these two systems. However, group-level correlations cannot illuminate potential within-subject interactions between the amygdala and cortical regions involved in recognition memory. We investigated this relationship directly using a functional connectivity analysis.
Patients With Schizophrenia Demonstrate Abnormal Amygdalo-Cortical Connectivity
Substantial behavioral evidence indicates that threat-sensitivity may impair recognition memory (6). Dolcos and McCarthy (8) found that in healthy subjects, dorsal cortical regions were activated by a working memory task, while ventral limbic regions responded to threatening emotional distracters. In addition, greater limbic responses to threat were correlated with impaired memory performance. In the present study, we found that activity in emotion-responsive limbic regions was negatively correlated with activity in dorsal cortical regions implicated in cognition. Patients showed a markedly different pattern from healthy comparison subjects, with increased amygdalo-limbic and amygdalo-cortical functional connectivity, indicating a disruption of the normally anticorrelated amygdalo-cortical relationship observed in healthy comparison subjects. Notably, group differences in amygdala functional connectivity occurred within the lateral parietal cortex and middle prefrontal gyrus. These same regions demonstrated group differences and symptom correlations in the hit versus correct rejection contrast. Although firm mechanistic interpretations are premature, these results suggest that increased sensitivity of the amygdala to potentially threatening stimuli may negatively affect the function of cortical regions involved in cognition.
Limitations and Summary
Several limitations of this study should be acknowledged. First, grouping stimuli into categories of threat and nonthreat, suggested in an earlier investigation (31), may have obscured relevant differences between emotions. For example, although an angry face represents a direct threat indicated by gaze, a fearful face indicates a more ambiguous environmental threat (40). Second, the low accuracy in target recognition led our study to be underpowered for certain performance-based comparisons. Performance was limited by the general difficulty of face recognition tasks (2) and by the fact that subjects were not notified that they would be asked to later recognize the faces from the emotion identification task. We observed a conservative response bias, suggesting that low target accuracy was not the result of guessing. Although poor performance likely prevented discernment of group differences in accuracy, group differences in response time were observed. In the future, it will be important to further assess the effects of correct recognition on threat response with a design that produces more correct trials and incorporates behavioral metrics of recognition confidence. Third, in order to constrain multiple comparisons, we did not examine individual components of symptoms beyond overall severity and paranoia. Future studies should investigate the effects of paranoia on threat responsiveness with more detailed assessments in a larger sample as well as relationships with other specific symptoms. Finally, although our findings remained significant even when medication dose was added as a covariate to the models, we cannot exclude the influence of medication in our sample of patients. Interestingly, we found that increased doses of medication were correlated with diminished threat response in the amygdala and orbital frontal cortex but also diminished recognition response in the parietal cortex. Although preliminary, this finding corroborates evidence that antipsychotics may reduce psychotic symptoms but also impair cognition at high doses.
Notwithstanding these limitations, this study demonstrates that patients with schizophrenia have symptom-related abnormalities in both cognitive and affective components of face recognition memory. The functional connectivity analysis indicates these deficits may be related: an abnormal response to the emotional elements of the task may impair performance of the task's cognitive demands. These results link patients' deficits in recognition memory to parietal abnormalities, extending previous results relating cognitive deficits to fronto-temporal abnormalities. Furthermore, our data also suggest that some degree of cognitive impairment may be related to abnormalities in threat processing. Thus, development of new treatments for cognitive impairment in schizophrenia should consider affective dysregulation as well.
1 : The Consortium on the Genetics of Schizophrenia: neurocognitive endophenotypes. Schizophr Bull 2007; 33:49–68 Crossref, Medline, Google Scholar
2 : Face recognition memory deficits and visual object memory performance in patients with schizophrenia and their relatives. Am J Psychiatry 2005; 162:1963–1966 Link, Google Scholar
3 : Emotion recognition deficit in schizophrenia: association with symptomatology and cognition. Biol Psychiatry 2000; 48:127–136 Crossref, Medline, Google Scholar
4 : Impairment in the specificity of emotion processing in schizophrenia. Am J Psychiatry 2006; 163:442–447 Link, Google Scholar
5 : The relationships among cognition, motivation, and emotion in schizophrenia: how much and how little we know. Schizophr Bull 2005; 31:875–881 Crossref, Medline, Google Scholar
6 : Emotional modulation of cognitive control: approach-withdrawal states double-dissociate spatial from verbal two-back task performance. J Exp Psychol Gen 2001; 130:436–452 Crossref, Medline, Google Scholar
7 : Dissociation in human prefrontal cortex of affective influences on working memory-related activity. Proc Natl Acad Sci U S A 2002; 99:1736–1741 Crossref, Medline, Google Scholar
8 : Brain systems mediating cognitive interference by emotional distraction. J Neurosci 2006; 26:2072–2079 Crossref, Medline, Google Scholar
9 : Neuropsychological deficits in neuroleptic naive patients with first-episode schizophrenia. Arch Gen Psychiatry 1994; 51:124–131 Crossref, Medline, Google Scholar
10 : Frontotemporal cerebral blood flow change during executive and declarative memory tasks in schizophrenia: a positron emission tomography study. Neuropsychology 1998; 12:399–413 Crossref, Medline, Google Scholar
11 : Alterations of fronto-temporal connectivity during word encoding in schizophrenia. Psychiatry Res 2007; 154:221–232 Crossref, Medline, Google Scholar
12 : Event-related fMRI of frontotemporal activity during word encoding and recognition in schizophrenia. Am J Psychiatry 2004; 161:1004–1015 Link, Google Scholar
13 : Complexity of prefrontal cortical dysfunction in schizophrenia: more than up or down. Am J Psychiatry 2003; 160:2209–2215 Link, Google Scholar
14 : Parietal lobe contributions to episodic memory retrieval. Trends Cogn Sci 2005; 9:445–453 Crossref, Medline, Google Scholar
15 : Functional-anatomic correlates of memory retrieval that suggest nontraditional processing roles for multiple distinct regions within posterior parietal cortex. J Neurosci 2004; 24:10084–10092 Crossref, Medline, Google Scholar
16 : Coherent spontaneous activity identifies a hippocampal-parietal memory network. J Neurophysiol 2006; 96:3517–3531 Crossref, Medline, Google Scholar
17 : Disruption of large-scale brain systems in advanced aging. Neuron 2007; 56:924–935 Crossref, Medline, Google Scholar
18 : Hyperactivity and hyperconnectivity of the default network in schizophrenia and in first-degree relatives of persons with schizophrenia. Proc Natl Acad Sci U S A 2009; 106:1279–1284 Crossref, Medline, Google Scholar
19 : Increased medial temporal lobe activation during the passive viewing of emotional and neutral facial expressions in schizophrenia. Schizophr Res 2006; 82:153–162 Crossref, Medline, Google Scholar
20 : Evidence that altered amygdala activity in schizophrenia is related to clinical state and not genetic risk. Am J Psychiatry 2009; 166:216–225 Link, Google Scholar
21 : Limbic activation associated with misidentification of fearful faces and flat affect in schizophrenia. Arch Gen Psychiatry 2007; 64:1356–1366 Crossref, Medline, Google Scholar
22 : Frontolimbic responses to emotional face memory: the neural correlates of first impressions. Hum Brain Mapp 2009; 30:3748–3758 Crossref, Medline, Google Scholar
23 : Neural correlates of episodic retrieval success. Neuroimage 2000; 12:276–286 Crossref, Medline, Google Scholar
24 : Functional-anatomic correlates of sustained and transient processing components engaged during controlled retrieval. J Neurosci 2003; 23:8460–8470 Crossref, Medline, Google Scholar
25 : A functional anatomic study of emotion in schizophrenia. Schizophr Res 2002; 58:159–172 Crossref, Medline, Google Scholar
26 : Neural circuitry for accurate identification of facial emotions. Brain Res 2008; 1194:37–44 Crossref, Medline, Google Scholar
27 : Brain activation during facial emotion processing. Neuroimage 2002; 16(3 pt 1):651–662 Crossref, Google Scholar
28 : An fMRI study of facial emotion processing in patients with schizophrenia. Am J Psychiatry 2002; 159:1992–1999 Link, Google Scholar
29 : A method for obtaining 3-dimensional facial expressions and its standardization for use in neurocognitive studies. J Neurosci Methods 2002; 115:137–143 Crossref, Medline, Google Scholar
30 : Brain systems that mediate both emotion and cognition. Cogn Emotion 1990; 4:269–288 Crossref, Google Scholar
31 : Amygdala activation during masked presentation of emotional faces predicts conscious detection of threat-related faces. Brain Cogn 2006; 61:243–248 Crossref, Medline, Google Scholar
32 : Modulating emotional responses: effects of a neocortical network on the limbic system. Neuroreport 2000; 11:43–48 Crossref, Medline, Google Scholar
33 : Pragmatics of measuring recognition memory: applications to dementia and amnesia. J Exp Psychol Gen 1988; 117:34–50 Crossref, Medline, Google Scholar
34 : An investigation of attributional style in first-episode psychosis. Br J Clin Psychol 1999; 38:181–194 Crossref, Medline, Google Scholar
35 : Puzzlingly high correlations in fMRI studies of emotion, personality, and social cognition. Perspect Psychol Sci 2009; 4:274–290 Crossref, Medline, Google Scholar
36 : Automated Talairach atlas labels for functional brain mapping. Hum Brain Mapp 2000; 10:120–131 Crossref, Medline, Google Scholar
37 : The human brain is intrinsically organized into dynamic, anticorrelated functional networks. Proceedings of the National Academy of Sciences of the United States of America 2005; 102:9673–9678 Crossref, Medline, Google Scholar
38 : Distinct cortical anatomy linked to subregions of the medial temporal lobe revealed by intrinsic functional connectivity. J Neurophysiol 2008; 100:129–139 Crossref, Medline, Google Scholar
39 : Social predictors of psychotic experiences: specificity and psychological mechanisms. Schizophr Bull 2008; 34:1012–1020 Crossref, Medline, Google Scholar
40 : Anxiety predicts a differential neural response to attended and unattended facial signals of anger and fear. Neuroimage 2009; 44:1144–1151 Crossref, Medline, Google Scholar