Thalamic Volumes in Patients With First-Episode Schizophrenia
Abstract
OBJECTIVE: The thalamus, a highly evolved sensory and motor gateway to the cortex, has been implicated in the pathophysiology of several illnesses, including schizophrenia. Several studies have suggested thalamic volume differences in patients with schizophrenia, although only a few studies have examined thalamic structure in new-onset patients. METHOD: The authors used magnetic resonance imaging to measure thalamic volumes in previously untreated patients with first-episode schizophrenia (N=16) relative to those of healthy comparison subjects (N=25). The age range of the patients and comparison subjects was 15 to 45 years of age. Thalamic volumes in the right and left hemispheres were segmented and analyzed, both separately and as total thalamic volume, by a rater blind to clinical data. The thalamus was further segmented into regions that roughly reflected individual thalamic nuclei. Analysis of covariance was used to control for intracranial volume. RESULTS: Right, left, and total thalamic volumes of the patients with schizophrenia were significantly smaller than those of the comparison subjects. Significantly smaller volumes were found in the left central medial subdivision of the patients as well as a smaller volume in the right central medial subdivision that approached significance. These regions primarily comprised the dorsomedial nucleus, a thalamic nucleus thought to be an important component of aberrant circuitry in schizophrenia. Significant volume differences were also seen in the left anterior, right anterior, and right posterior medial subdivisions. CONCLUSIONS: These findings suggest significant thalamic volumetric differences between patients with newly diagnosed schizophrenia and healthy comparison subjects. Future analysis of individual thalamic nuclei may reveal important, specific relationships between thalamic abnormalities and schizophrenia.
Schizophrenia is a complex disease, the pathogenesis of which remains to be elucidated. Alterations in cortical-subcortical circuitry and the components of these pathways may contribute to positive and negative symptoms and executive function deficits and may result from abnormal brain development (1–6). Examination of the early course of the disease may reveal important characteristics of schizophrenic brains that may underlie disease evolution and progression.
Several studies have demonstrated morphological and functional changes in the thalamus of patients with schizophrenia. The thalamus is the vital relay station to the cortex and is important for consciousness, perception, and the integration of thought processes (6). An aberrant thalamus could contribute to the perceptual and motor dysfunction of schizophrenia (7). It has been postulated that abnormal prefrontal-thalamic-cerebellar circuitry may contribute to the cognitive impairment characteristic of the disease (5, 8). Cell loss and reduced tissue volume of the thalamus have been described in several studies of schizophrenic brains (9–13). Furthermore, imaging studies have consistently revealed smaller thalamic volumes in patients with schizophrenia as well as altered thalamic perfusion and metabolism (8, 14–22).
The most recent emphasis on the neurodevelopmental model of schizophrenia (1–6) points to the importance of first-episode schizophrenia research. Studies of first-episode patients may reveal the biological basis of psychotic disorders without the potential confounds of prior treatment and illness chronicity. Prospective studies of this population can clarify the impact of illness course and treatment on neurobiology (23). Because a specific thalamic role in schizophrenia remains unknown, and most structural and functional thalamic imaging studies have examined chronic, treated patients, we sought to measure thalamic volume in treatment-naive patients with first-episode schizophrenia. Furthermore, since the thalamus is composed of several nuclei, we conducted the first study to our knowledge that has measured subdivisions of the thalamus in this patient population. Our goal was to better understand the role of individual thalamic nuclei, particularly the dorsomedial nucleus and pulvinar, which have connections to brain regions previously implicated in the pathophysiology of schizophrenia (6). We hypothesized that the regions mainly comprising the dorsomedial nucleus and pulvinar in patients with first-episode schizophrenia would differ from those of healthy comparison subjects.
Method
Subjects were 16 patients with schizophrenia and 25 healthy comparison subjects. The patients (15 with schizophrenia and one with schizoaffective disorder, depressed type) were diagnosed according to DSM-IV criteria at consensus conference meetings of senior diagnostic/clinical researchers approximately 1 month after entry into the study. All available clinical information and data gathered by using the Structured Clinical Interview for DSM-III-R Axis I disorders (SCID) (24) were incorporated. All diagnoses were ascertained as part of a longitudinal, prospective study and included a careful diagnostic rereview to ensure diagnostic stability (23). None of the subjects currently met criteria for DSM-IV psychoactive substance abuse or dependence; two subjects had a prior history of substance abuse and were dropped from the study. None of the patients had histories of significant head injury (with loss of consciousness of more than 10 minutes), neurological or medical illness, prior neuroleptic treatment, or mental retardation (IQ lower than 75). Illness duration was computed from the date of onset of prodromal symptoms to entry into the study. Clinical ratings were determined by using the Brief Psychiatric Rating Scale (BPRS) (25).
The healthy comparison subjects were recruited through advertisements in the same local neighborhoods and communities in which the patients resided. These subjects were free from any current or past axis I disorder (including substance abuse or dependence) as determined by the nonpatient version of the SCID. None had any prior exposure to any psychotropic medication within 6 months of the baseline assessment; a history of neurologic disorders or any other chronic medical problems with potential to influence neurological function; or mental retardation (IQ lower than 75). None reported a history of schizophrenia or major mood disorder in a first-degree relative. The comparison subjects were matched, as a group, with the schizophrenia patients for age, gender, race, and parental socioeconomic status (from the Hollingshead Four-Factor Index [26]). After an initial screening evaluation by telephone, these subjects were interviewed by a psychiatrist (M.S.K.) or clinical psychologist with the SCID (nonpatient version).
After complete description of the study to the subjects, written informed consent was obtained. The study was approved by the Biomedical Institutional Board of the University of Pittsburgh.
MRI Studies
All volumetric magnetic resonance imaging (MRI) scans were conducted at the University of Pittsburgh Medical Center (1.5-T Signa Whole Body Scanner, GE Medical Systems, Milwaukee). Image quality and clarity as well as patient head position were determined with a sagittal scout series. Total brain volume was measured with a three-dimensional spoiled gradient recalled acquisition in the steady state pulse sequence that obtained 124 1.5-mm thick contiguous coronal images (TE=5 msec, TR=25 msec, acquisition matrix=256 × 192, field of view=24 cm, flip angle=40°). In order to facilitate image orientation, coronal slices were obtained perpendicular to the anterior commissure-posterior commissure line. Axial proton density and T2-weighted images were obtained for thalamic measurement and to exclude structural abnormalities on MRI scans. Image software (version 1.56) developed by the National Institutes of Health was used to measure brain anatomy (27). This technique yields valid and reliable neuroanatomic measurements of the regions of interest with a semiautomated segmentation approach (28). None of the MRI scans in this data set showed motion or magnetic field inhomogeneity artifacts.
Neuroanatomic Measures
We measured the right and left hemispheres of the thalamus, their subdivisions, and total intracranial volume, which was used as a covariate. We also measured the volume of the dorsolateral prefrontal cortex in view of the implications of this structure in the pathophysiology of schizophrenia (3, 28) and its reciprocal connections with the thalamic nuclei (6). Neuroanatomic boundaries were determined by reference to standard neuroanatomical atlases (29, 30), and detailed definitions were adapted from previously published psychiatric neuroimaging studies of the thalamus (8, 15, 16, 31–33).
Thalamic Volume
Separate measurements were obtained for the left and right hemispheres of the thalamus by using a manual tracing technique. The mamillary body was used as the anterior boundary. The internal capsule was considered the lateral boundary, the third ventricle the medial boundary, and the inferior border of the third ventricle the inferior boundary. The posterior boundary was defined by where the hemispheres of the thalamus merged under the crux fornix. The superior boundary was the main body of the lateral ventricle (32). Measurements were made by a single well-trained and reliable rater (A.R.G.) who was blind to the study hypothesis, subject identification, and clinical data.
Thalamic Subdivisions
The thalamus was first divided into two distinct medial and lateral regions (axial view in Figure 1). The point of reference for the line that bisected the thalamus into medial and lateral regions was the emergence of the fourth ventricle as it appeared as a vertical line through the pons. A line drawn parallel to the lateral border of the midbrain, interhemispheric fissure, and cerebral aqueduct represented our line of vertical bisection of each thalamus (coronal view in Figure 1). This line was carried on through all thalamic slices to create a plane of bisection parallel to the interhemispheric fissure. We chose this approach because no significant differences have been seen between patients with schizophrenia and comparison subjects with regard to brainstem structures (34). Using the spoiled gradient recalled acquisition protocol, we measured contiguous coronal slices of the thalamus, dividing the regions into anterior, central, and posterior divisions. The anterior and central divisions each contained 40% of the total number of slices; the posterior division contained 20% of the total number of slices (axial and sagittal views in Figure 1). These divisions are based upon neuroanatomical atlases of the human brain as well as a previously published article examining the thalamus in schizophrenia (16, 29, 30). The divisions roughly reflect the individual nuclei that are located in these thalamic regions (axial view in Figure 1). Each individual subdivision was measured as a percentage of the total number of slices. Measurements were made by a single, well-trained, and reliable rater (A.R.G.) who was blind to the study hypothesis, subject identification, and clinical data.
Dorsolateral Prefrontal Cortical Volume
The dorsolateral prefrontal cortex was measured in the coronal plane by a method similar to that used by Seidman et al. (35). The most posterior part of the genu was located and used as the first slice in measuring the dorsolateral prefrontal cortex. The borders of the dorsolateral prefrontal cortex were first manually outlined, and the gray matter was then segmented. The superior boundary was the superior frontal sulcus, and the inferior boundary was the posterior lateral fissure and the horizontal ramus of the anterior lateral fissure. The lateral border was the edge of the cerebral cortex, and the medial border was created by connecting the deepest points on the superior frontal sulcus and the lateral fissure. Ten successive anterior slices were measured. The dorsolateral prefrontal cortex was segmented, and the gray matter and total volume were measured.
Intracranial Volume
Intracranial volume included the following volumes: total brain, dura, ventricular and extraventricular CSF, brainstem, and cerebellum; this was measured by manually outlining the outer limit of the tracing along the inner border of the inner table of skull. This measurement was made from the coronal slices. The intracranial volume was used as a covariate in comparison to volumetric measures of the aforementioned structures across diagnostic groups.
Statistical Analysis
The thalamic volumes and their subdivisions were compared between subjects by using analysis of covariance, with the intracranial volume as a covariate. The relations between thalamic volumes and subject characteristics (age, symptom severity) were examined by using Pearson correlation coefficients. Interrater (K.H., A.R.G.) reliabilities (intraclass correlations; N=10) for all measurements and intrarater reliabilities for measurements by the same rater (A.R.G.), conducted approximately 1 month apart on 10 scans, were all greater than 0.90.
Results
The two groups did not significantly differ with regard to age, gender, or handedness (Table 1). The right hemisphere, left hemisphere, and total thalamic volumes of the patient group were significantly smaller than those of the comparison subjects, after we controlled for intracranial volume (Table 2). Intracranial volume did not significantly differ between the groups. When only right-handed subjects (12 patients and 23 comparison subjects) were examined, there were continuing differences between the groups (left hemisphere: F=9.34, df=1, 33, p=0.004; right hemisphere: F=3.86, df=1, 33, p=0.06; total thalamus: F=7.14, df=1, 33, p=0.01). There were no differences observed when we controlled for handedness.
No significant gender effect was seen for left-hemisphere thalamic volume (F=1.75, df=1, 36, p=0.19), but female subjects had smaller right-hemisphere thalamic volumes (F=4.18, df=1, 36, p=0.04). No gender-by-diagnosis interactions were seen for either structure. Right hemisphere, left hemisphere, and total thalamic volumes, after we controlled for intracranial volume, did not correlate with age in either the patients (partial correlation coefficients<0.25, df=13, p>0.50) or the comparison subjects (partial correlation coefficients<0.25, df=22, p>0.50). In the patient group, thalamic volumes were not correlated with Global Assessment of Functioning scores (r<0.15, df=14, p>0.60) or total BPRS scores (r<0.15, df=14, p>0.60). When the patient with schizoaffective disorder was excluded, thalamic volume differences remained significant (left hemisphere: F=8.53, df=1, 37, p=0.006; right hemisphere: F=5.84, df=1, 37, p=0.02; total: F=8.08, df=1, 37, p=0.007). The number of coronal slices used to quantify the thalamus ranged from 13 to 21 slices, with an average of 17.5 slices. There were no significant differences between groups in the number of slices.
Thalamic Subdivisions
Measurements of thalamic subdivisions were conducted in 16 patients and 25 comparison subjects (Table 3). The 18% difference in volume for the left central medial subdivision was significant, and the 17% difference in volume for the right central medial subdivision approached significance. There was a highly significant difference in right posterior medial subdivision volume (43% smaller in the patient group). Significant volume differences were also found in the right and left anterior medial subdivisions (13% and 14%, respectively). There were no significant volume differences in the lateral subdivisions.
Dorsolateral Prefrontal Cortical Volume
The dorsolateral prefrontal cortex volumes (gray, white, total) did not differ significantly between patients and comparison subjects. However, the left dorsolateral prefrontal cortex gray matter volume was smaller in the patients than in the comparison subjects (mean=9.94 cm3 [SD=1.35] versus 10.56 cm3 [SD=1.13], respectively) (F=2.86, df=1, 38, p<0.10). No significant correlations were seen between the thalamic and thalamic subdivision volumes on the one hand and the gray, white, and total dorsolateral prefrontal cortex volumes on the other (all partial correlations [after partialing out intracranial volume] <0.50, df=13, p>0.20).
Discussion
Consistent with the results of other early course as well as chronic schizophrenia studies, we have demonstrated significant thalamic volume differences in patients with first-episode schizophrenia relative to those of healthy comparison subjects. Furthermore, by examining treatment-naive, first-episode patients, we have eliminated the potentially confounding effects of prior treatment and illness chronicity.
Thalamic volume differences in patients with schizophrenia may reflect abnormal thalamic circuitry—a potential contributor to the cognitive dysfunction characteristic of schizophrenia. The thalamus is a recognized component of frontal, cerebellar, and limbic circuits (36–38). Lesions of thalamic nuclei have been reported to result in neuropsychological and behavioral disturbances similar to the deficits of executive function in patients with schizophrenia. Vascular and degenerative disorders affecting the thalamus are often indistinguishable from “frontal lobe”-type syndromes (36). With many cortical and subcortical connections, the thalamus is thought to filter sensory information before it reaches the cortex. Therefore, abnormal thalamic function could result in the inability to filter or “gate” sensory input to the cortex, creating the type of sensory overload often experienced by patients with schizophrenia (5, 39). Furthermore, an aberrant thalamic component to thalamocortical circuitry may contribute to or result from the frontal lobe dysfunction described in previous schizophrenia research (8). However, our inability to find a correlation between thalamic and dorsolateral prefrontal cortex volume alterations suggests that caution is warranted for such conclusions.
Thalamic volume differences in patients with schizophrenia, suggestive of aberrant thalamic circuitry, are consistent with previous structural and functional studies that have demonstrated abnormalities in other brain regions. Several postmortem studies have revealed differences in superior temporal gyrus cell and total cortical volumes as well as lower prefrontal and anterior cingulate cell densities in schizophrenic brains (40–43). Differences in cell number, density, and volume in the mediodorsal thalamic nucleus have been reported as well as lower cell volume in the globus pallidus (9, 13, 44). Imaging studies have revealed smaller thalamic volumes and less glucose metabolism and neuronal activity in patients with schizophrenia (15, 16, 20, 21). Furthermore, abnormal striatal (45, 46) as well as superior temporal gyral volumes (47) have been observed. Functional imaging studies have consistently demonstrated abnormal perfusion and metabolism in cortical and cerebellar regions of schizophrenic brains (8, 48–51). These findings support the concept of abnormal cortical-subcortical circuitry underlying the pathophysiology of schizophrenia. A greater understanding of the thalamic components of these circuits is critical.
We have previously proposed that a developmentally mediated neural dysplasia in cortical-subcortical circuitry may be associated with the pathogenesis of schizophrenia. A substantial reorganization of cortical connections, involving a programmed synaptic pruning, takes place during human development (28). It has been suggested that in schizophrenia, aberrant migration or programmed cell death in the subplate zone of the developing cortex may impair normal patterns of synaptic connections in the overlying cortex and lead to compromised circuitry (6). Recent studies have revealed abnormal thalamic projections to the prefrontal cortex and other signs of impaired thalamocortical connectivity in schizophrenia (52, 53). The primary versus secondary nature of thalamic dysfunction in schizophrenia remains unclear; however, it is understood that neuronal loss in the thalamus is commonly a retrograde degeneration ensuing from pathology in the cerebral cortex that affects the axon terminations of thalamocortical relay cells. Furthermore, retrograde degeneration secondary to cortical damage in the developing brain is typically unaccompanied by gliosis (6). It is interesting to note that the lower neuronal density described in postmortem analysis of the thalamus occurred in the absence of gliosis, which suggests a neurodevelopmental rather than neurodegenerative process (8).
While smaller thalamic volumes in patients with schizophrenia has been a consistent finding in both in vivo and postmortem studies, the majority of this research has examined chronic or treated patients (9, 14, 15, 17, 20, 54, 55). We have demonstrated that caudate volumetric measurements may differ between neuroleptic-naive and treated schizophrenia patients, perhaps secondary to the effects of neuroleptic treatment (28). In light of these potential treatment-induced changes, we sought to examine patients with untreated schizophrenia. It is possible that, similar to our caudate findings (28), volumetric measurements following 1 year of antipsychotic treatment may reveal significant treatment effects. A previous study (18) revealed no change in thalamic volumes, as compared to control subjects, in five schizophrenia patients following approximately 70 days of risperidone treatment, although higher doses of atypical neuroleptics were associated with larger thalamic volumes. It was suggested that medication-induced hypertrophy may have been responsible for the volumetric changes.
The inability to precisely measure discrete thalamic nuclei limits the interpretation of our findings. Our current measurements of thalamic subdivisions revealed significant and nearly significant volume differences in the regions primarily comprising the dorsomedial nucleus. As previously mentioned, postmortem analysis of schizophrenic brains has revealed a significant neuronal deficit in the dorsomedial nucleus. It is of interest that these changes appeared to be unaffected by treatment (9). Because of its connections to the prefrontal cortex, medial temporal cortex, and basal forebrain, all of which have been implicated in the pathophysiology of schizophrenia, the dorsomedial nucleus is thought to be an important component of aberrant circuitry (6). We also found significant volume differences in the right posterior medial subdivision, a thalamic region roughly reflecting the right medial half of the pulvinar. The pulvinar may also represent a critical thalamic nucleus in that it projects to temporal lobe regions reported to be abnormal in patients with schizophrenia and might be associated with altered visual perceptual functions (6, 42, 47).
It is important to note that our measurement of thalamic subdivisions has several limitations. We were unable to delineate and employ an intrathalamic marker as a consistent landmark for our regional subdivisions. Rather, we relied upon approximate percentage-based divisions of total thalamic area as a means of dividing the thalamus. It is possible that our approach may not account for thalamic volume loss that is inconsistent with these percentage-based divisions. Our approach assumes that all thalamic nuclei will be consistently represented by these rigid subdivisions. It is possible that some patients have thalamic nuclei that are not in proportion to the percentage-based parameters that we defined in this study. Furthermore, an enlarged third ventricle causing a mass-effect shift in thalamic volume could be misinterpreted as thalamic volume loss. Higher resolution imaging may allow for the delineation of an intrathalamic marker that may improve the reliability of our approach. Furthermore, by using more advanced imaging techniques, such as diffusion tensor imaging (56), it may be possible to delineate the white matter tracts that connect thalamic nuclei with their cortical projections and better understand the role of these circuits in schizophrenia.
![]() |
![]() |
![]() |
Received May 8, 2000; revision received Oct. 31, 2000; accepted Nov. 2, 2000. From the Department of Psychiatry, Western Psychiatric Institute and Clinic; and the Department of Psychiatry, Wayne State University School of Medicine, Detroit. Address reprint requests to Dr. Keshavan, Western Psychiatric Institute and Clinic, 3811 O’Hara St., Pittsburgh, PA 15213-2593; [email protected] (e-mail). Supported in part by NIMH grants MH-45203 and MH-45156 and a Scottish Rite grant. The authors thank Dr. David Lewis for feedback, Drs. Gretchen L. Haas, Elizabeth D. Radomsky, and Nina Schooler for clinical characterization of the study subjects, and Ilana Mankowski for morphometric measurements.
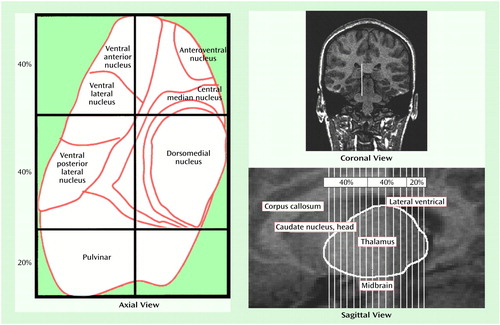
Figure 1. Axial, Coronal, and Sagittal Views of the Thalamic Regions Measured to Assess Volume Differences Between Patients With First-Episode Schizophrenia and Healthy Comparison Subjectsa
aApproximate regions of specific thalamic nuclei are depicted in the representation of the axial view, which was adapted, with permission, from Buchsbaum et al. (16). The line drawn in the coronal view—which is parallel to the lateral border of the midbrain, interhemispheric fissure, and cerebral aqueduct—represents the line of vertical bisection of each thalamus. This line was carried on through all thalamic slices to create a plane of bisection parallel to the interhemispheric fissure. The average number of coronal slices composing the anterior, central, and posterior subdivisions of the thalamus is shown in the sagittal view.
1. Feinberg I: Schizophrenia: caused by a fault in programmed synaptic elimination during adolescence? J Psychiatr Res 1983; 17:319–334Google Scholar
2. Fish B: Neurological antecedents of schizophrenia in children: evidence for an inherited, congenital neurointegrative defect. Arch Gen Psychiatry 1977; 34:1297–1313Google Scholar
3. Weinberger DR: Implications of normal brain development for the pathogenesis of schizophrenia. Arch Gen Psychiatry 1987; 44:660–669Crossref, Medline, Google Scholar
4. Murray RM, Lewis SW: Is schizophrenia a neurodevelopmental disorder? Br Med J 1987; 295:681–682Google Scholar
5. Andreasen NC: A unitary model of schizophrenia: Bleuler’s “fragmented phrene” as schizencephaly. Arch Gen Psychiatry 1999; 56:781–787Crossref, Medline, Google Scholar
6. Jones EG: Cortical development and thalamic pathology. Schizophr Bull 1997; 23:483–501Crossref, Medline, Google Scholar
7. Scheibel AB: The thalamus and neuropsychiatric illness. J Neuropsychiatry Clin Neurosci 1997; 9:342–353Crossref, Medline, Google Scholar
8. Andreasen NC: The role of the thalamus in schizophrenia. Can J Psychiatry 1997; 42:27–33Crossref, Medline, Google Scholar
9. Pakkenberg B: Pronounced reduction of total neuron number in mediodorsal thalamic nucleus and nucleus accumbens in schizophrenia. Arch Gen Psychiatry 1990; 47:1023–1028Google Scholar
10. Pakkenberg B: The volume of the mediodorsal thalamic nucleus in treated and untreated schizophrenics. Schizophr Res 1992; 7:95–100Crossref, Medline, Google Scholar
11. Fungfeld E: Pathological-anatomische untersuchungen bei Dementia praecox mit besonderer beruckischtigung des Thalamus opticus. Z Gesamte Neurol Psychiatrie 1925; 95:411–463Crossref, Google Scholar
12. Baumer H: Veranderungen des Thalamus bei Schizophrenie. J Hirnforsch 1954; 1:157–172Google Scholar
13. Treff WM, Hempel KJ: Die zelidichte bei Schizophrenen und klinisch gesunden. J Hirnforsch 1958; 4:314–369Medline, Google Scholar
14. Andreasen NC, Ehrhardt JC, Swayze VW II, Alliger RJ, Yuh WT, Cohen G, Ziebell S: Magnetic resonance imaging of the brain in schizophrenia: the pathophysiologic significance of structural abnormalities. Arch Gen Psychiatry 1990; 47:35–44Crossref, Medline, Google Scholar
15. Andreasen NC, Arndt S, Swayze V II, Cizadlo T, Flaum M, O’Leary D, Ehrhardt JC, Yuh WTC: Thalamic abnormalities in schizophrenia visualized through magnetic resonance image averaging. Science 1994; 266:294–298Crossref, Medline, Google Scholar
16. Buchsbaum MS, Someya T, Teng CY, Abel L, Najafti A, Haier RJ, Wu J, Bunney WE Jr: PET and MRI of the thalamus in never-medicated patients with schizophrenia. Am J Psychiatry 1996; 153:191–199Link, Google Scholar
17. Frazier JA, Giedd JN, Hamburger SD, Albus KE, Kaysen D, Vaituzis AC, Rajapakse JC, Lenane MC, McKenna K, Jacobsen LK, Gordon CT, Breier A, Rapoport JL: Brain anatomic magnetic resonance imaging in childhood-onset schizophrenia. Arch Gen Psychiatry 1996; 53:617–624Crossref, Medline, Google Scholar
18. Gur RE, Maany V, Mozley PD, Swanson C, Bilker W, Gur RC: Subcortical MRI volumes in neuroleptic-naive and treated patients with schizophrenia. Am J Psychiatry 1998; 155:1711–1717Google Scholar
19. Rodriguez VM, Andree RM, Castejon MJ, Zamora ML, Alvaro PC, Delgado JL, Vila FJ: Fronto-striato-thalamic perfusion and clozapine response in treatment-refractory schizophrenic patients: a 99m Tc-HMPAQ study. Psychiatry Res 1997; 76:51–61Crossref, Medline, Google Scholar
20. Holcomb HH, Cascella NG, Thaker GK, Medoff DR, Dannals RF, Tamminga CA: Functional sites of neuroleptic drug action in the human brain: PET/FDG studies with and without haloperidol. Am J Psychiatry 1996; 153:41–49Link, Google Scholar
21. Heimberg C, Komoroski RA, Lawson WB, Cardwell D, Karson CN: Regional proton magnetic resonance spectroscopy in schizophrenia and exploration of drug effect. Psychiatry Res 1998; 83:105–115Crossref, Medline, Google Scholar
22. Gunther W: MRI-SPECT and PET-EEG findings on brain dysfunction in schizophrenia. Prog Neuropsychopharmacol Biol Psychiatry 1992; 16:445–462Crossref, Medline, Google Scholar
23. Keshavan MS, Schooler NR: First-episode studies in schizophrenia: criteria and characterization. Schizophr Bull 1992; 18:491–513Crossref, Medline, Google Scholar
24. Spitzer RL, Williams JBW: Structured Clinical Interview for DSM-III-R (SCID). New York, New York State Psychiatric Institute, Biometrics Research, 1985Google Scholar
25. Overall JE, Gorham DR: The Brief Psychiatric Rating Scale. Psychol Rep 1962; 10:799–812Crossref, Google Scholar
26. Hollingshead AB: Four-Factor Index of Social Status. New Haven, Conn, Yale University, Department of Sociology, 1975Google Scholar
27. Rasband W: NIH Image Manual. Bethesda, Md, National Institutes of Health, 1993Google Scholar
28. Keshavan MS, Anderson S, Pettegrew JW: Is schizophrenia due to excessive synaptic pruning in the prefrontal cortex? the Feinberg hypothesis revisited. J Psychiatr Res 1994; 28:239–265Crossref, Medline, Google Scholar
29. Daniels DL, Haughton VM, Naidich TP: Cranial and Spinal Magnetic Resonance Imaging: An Atlas and Guide. New York, Raven Press, 1987Google Scholar
30. Talairach J, Tournoux P: Co-Planar Stereotaxic Atlas of the Human Brain. New York, Thieme Medical, 1988Google Scholar
31. Gilbert AR, Moore GJ, Keshavan MD, Paulson LD, Narula V, MacMaster FP, Stewart CM, Rosenberg DR: Decrease in thalamic volumes of pediatric obsessive compulsive disorder patients taking paroxetine. Arch Gen Psychiatry 2000; 57:449–456Crossref, Medline, Google Scholar
32. Portas CM, Goldstein JM, Shenton ME, Hokama HH, Wible CG, Fischer I, Kikinis R, Donnino R, Jolesz FA, McCarley RW: Volumetric evaluation of the thalamus in schizophrenic male patients using magnetic resonance imaging. Biol Psychiatry 1998; 43:649–659Crossref, Medline, Google Scholar
33. Staal WG, Pol HEH, Schnack H, van der Schot AC, Kahn RS: Partial volume decrease of the thalamus in relatives of patients with schizophrenia. Am J Psychiatry 1998; 155:1784–1786Google Scholar
34. Aylward EH, Reiss A, Barta PE, Tien A, Han W, Lee J, Pearlson GD: Magnetic resonance imaging measurement of posterior fossa structures in schizophrenia. Am J Psychiatry 1994; 151:1448–1452Google Scholar
35. Seidman LJ, Yurgelun-Todd D, Kremen WS, Woods BS, Goldstein JM, Faraone SV, Tsuang MT: Relationship of prefrontal and temporal lobe MRI measures to neuropsychological performance in chronic schizophrenia. Biol Psychiatry 1994; 35:235–246Crossref, Medline, Google Scholar
36. Cummings JL: Frontal-subcortical circuits and human behavior. Arch Neurol 1993; 50:873–880Crossref, Medline, Google Scholar
37. Alexander GE, Crutcher MD, DeLong MR: Basal ganglia-thalamo-cortical circuits: parallel substrates for motor, occulomotor, prefrontal and limbic functions, in Progress in Brain Research, vol 85. Edited by Uylins HBM, Van Eden CG, Corner MA, Feenstra MGP. Amsterdam, Elsevier, 1989, pp 119–146Google Scholar
38. Jones EG: The Thalamus. New York, Plenum, 1985Google Scholar
39. Braff DL, Swerdlow NR: Neuroanatomy of schizophrenia. Schizophr Bull 1997; 23:509–512Crossref, Medline, Google Scholar
40. Pakkenberg B: Total nerve cell number in neocortex in chronic schizophrenics and controls estimated using optical disectors. Biol Psychiatry 1993; 34:768–772Crossref, Medline, Google Scholar
41. Benes FM, McSparren J, San Giovanni JP, Vincent SL: Deficits in small interneurons in cingulate cortex of schizophrenics and schizoaffective patients. Arch Gen Psychiatry 1991; 48:996–1001Google Scholar
42. Akbarian S, Bunney WE, Potkin SG, Wigal SB, Hagman JO, Sandman CA, Jones EG: Altered distribution of nicotinamide-adenine-dinucleotide phosphate-diaphorase cells in frontal cortex of schizophrenics implies disturbances of cortical development. Arch Gen Psychiatry 1993; 50:169–177Crossref, Medline, Google Scholar
43. Falkai P, Bogerts B, Schneider T, Greve B, Pfeiffer U, Pilz K, Gonsiorzcyk C, Majtenyi C, Ovary I: Disturbed planum temporale asymmetry in schizophrenia: a quantitative post-mortem study. Schizophr Res 1995; 14:161–176Crossref, Medline, Google Scholar
44. Bogerts B, Falkai P, Haupts M, Greve B, Ernst S, Tapernon-Franz U, Heinzmann U: Post-mortem volume measurements of limbic system and basal ganglia structures in chronic schizophrenics: initial results from a new brain collection. Schizophr Res 1990; 3:295–301Crossref, Medline, Google Scholar
45. Flaum M, Swayze VW II, O’Leary DS, Yuh WTC, Ehrhardt JC, Arndt SV, Andreasen NC: Effects of diagnosis, laterality, and gender on brain morphology in schizophrenia. Am J Psychiatry 1995; 152:704–714Link, Google Scholar
46. Keshavan MS, Rosenberg D, Sweeney JA, Pettegrew JW: Decreased caudate volume in neuroleptic-naive psychotic patients. Am J Psychiatry 1998; 155:774–778Abstract, Google Scholar
47. Keshavan MS, Haas GL, Kahn CE, Aguilar E, Dick EL, Schooler NR, Sweeney JA, Pettegrew JW: Superior temporal gyrus and the course of early schizophrenia: progressive, static, or reversible? J Psychiatr Res 1998; 32:161–167Google Scholar
48. Shihabuddin L, Buchsbaum MS, Hazlett EA, Haznedar MM, Harvey PD, Newman A, Schnur DB, Spiegel-Cohen J, Wei T, Machac J, Knesaurek K, Vallabhajosula S, Biren MA, Ciaravolo TM, Luu-Hsia C: Dorsal striatal size, shape, and metabolic rate in never-medicated and previously medicated schizophrenics performing a verbal learning task. Arch Gen Psychiatry 1998; 55:235–243Crossref, Medline, Google Scholar
49. Berman KF, Illowsky BP, Weinberger DR: Physiological dysfunction of dorsolateral prefrontal cortex in schizophrenia, III: further evidence for regional and behavioral specificity. Arch Gen Psychiatry 1988; 45:616–622Crossref, Medline, Google Scholar
50. Weinberger DR, Berman KF, Zec RF: Physiological dysfunction of dorsolateral prefrontal cortex in schizophrenia: regional cerebral blood flow (rCBF) evidence. Arch Gen Psychiatry 1986; 43:114–124Crossref, Medline, Google Scholar
51. Gur RE, Mozley PD, Resnick SM, Mozley LH, Shtasel DL, Gallacher F, Arnold SE, Karp JS, Alavi A, Reivich M: Resting cerebral glucose metabolism in first-episode and previously treated patients with first episode schizophrenia relates to clinical features. Arch Gen Psychiatry 1995; 52:657–667Crossref, Medline, Google Scholar
52. Lewis DA, Pierri JN, Volk DW, Melchitsky DS, Woo TU: Altered GABA neurotransmission and prefrontal cortical dysfunction in schizophrenia. Biol Psychiatry 1999; 46:616–626Crossref, Medline, Google Scholar
53. Pierri JN, Chaudry AS, Woo T-UW, Lewis DA: Alterations in chandelier neuron axon terminals in the prefrontal cortex of schizophrenic subjects. Am J Psychiatry 1999; 156:1709–1719Google Scholar
54. Jeste DV, McAdams LA, Palmer BW, Braff D, Jernigan TL, Paulsen JS, Stout JC, Symonds LL, Bailey A, Heaton RK: Relationship of neuropsychological and MRI measures to age of onset of schizophrenia. Acta Psychiatr Scand 1998; 98:156–164Crossref, Medline, Google Scholar
55. Corey-Bloom J, Jernigan T, Archibald S, Harris MJ, Jeste DV: Quantitative magnetic resonance imaging of the brain in late-life schizophrenia. Am J Psychiatry 1995; 152:447–449Link, Google Scholar
56. Lim KO, Hedehus M, Moseley M, deCrespigny A, Sullivan EV, Pfefferbaum A: Compromised white matter tract integrity in schizophrenia inferred from diffusion tensor imaging. Arch Gen Psychiatry 1999; 56:367–374Crossref, Medline, Google Scholar