Altered Transcript Expression of NMDA Receptor-Associated Postsynaptic Proteins in the Thalamus of Subjects With Schizophrenia
Abstract
OBJECTIVE: NMDA receptor dysfunction has been implicated in the pathophysiology of schizophrenia. The NMDA receptor is a multimeric ligand-gated ion channel, and the obligate NR1 subunit is expressed as one of eight isoforms due to the alternative splicing of exons 5, 21, and 22. Alternative splicing of NR1 subunits modulates receptor function by influencing the association of NR1 with other NMDA receptor subunits and myriad intracellular molecules, such as the postsynaptic density family of proteins that target NMDA receptors to the synaptic membrane and couple it to numerous signal transduction enzymes. Recently, the authors reported that the NMDA receptor subunits NR1 and NR2C are abnormally expressed in the thalamus in schizophrenia. They hypothesized that this reduction is associated with specific NR1 isoforms and that NMDA receptor-related postsynaptic density proteins are abnormally expressed. METHOD: Using in situ hybridization, the authors examined expression of the transcripts encoding NR1 isoforms containing exons 5, 21, or 22, and the NMDA receptor-related postsynaptic density proteins NF-L, PSD93, PSD95, and SAP102. RESULTS: Reduced NR1 subunit transcript expression was restricted to exon 22-containing isoforms. Increased expression of the NMDA receptor-associated postsynaptic density proteins NF-L, PSD95, and SAP102 was also detected in the thalamus of subjects with schizophrenia. CONCLUSIONS: These data support the hypothesis of glutamatergic abnormalities in schizophrenia and suggest that glutamatergic dysfunction may occur not only at the level of receptor expression but also within intracellular pathways associated with glutamate receptor-associated signal transduction.
While prefrontal and temporal cortical dysfunction are associated with schizophrenia, recent attention has focused on the role of the thalamus in this illness (1, 2). The thalamus plays a critical role in the processing and integration of sensory information relevant to emotional and cognitive function. Abnormal thalamic activity, or a disturbance of corticothalamic circuitry, may contribute to sensory and cognitive deficits that schizophrenia patients often experience (3). In fact, multiple thalamic abnormalities have been reported in schizophrenia. Neuroimaging studies have consistently reported decreased thalamic volume (4–7) and altered metabolism (8–10) in patients with schizophrenia. Postmortem morphometric studies and magnetic resonance imaging investigations have found volume loss in specific thalamic nuclei, including the dorsomedial (6, 11–13), central medial, posteromedial (6), and anterior (6, 14) nuclei as well as the pulvinar (11). It is of interest that thalamic cell loss is most often found in the dorsomedial and anterior nuclei (which are reciprocally connected to limbic brain regions frequently implicated in schizophrenia) but not in nuclei with primarily nonlimbic projections (13).
While there is evidence of structural and functional thalamic changes in patients with schizophrenia, few studies have examined associated neurochemical abnormalities (15–18). Thalamic efferents, as well as corticothalamic and sensory afferents, predominately use glutamate as a neurotransmitter, which activates ionotropic (NMDA, AMPA, kainate) and metabotropic receptors expressed throughout thalamic nuclei. Several lines of investigation have implicated glutamatergic dysfunction in the pathophysiology of schizophrenia (19, 20). The glutamatergic hypothesis of schizophrenia rests largely on pharmacological observations, since NMDA receptor blockade with dissociative anesthetics like phencyclidine produces psychosis and cognitive deficits in normal subjects and exacerbates these symptoms in patients with schizophrenia (21). Moreover, enhancing NMDA receptor-mediated neurotransmission either with D-cycloserine (an agonist of the glycine/D-serine co-agonist site of the NMDA receptor) or ampakines (which stimulate AMPA receptors and indirectly enhance NMDA receptor function) improves negative symptoms in some patients (20, 22). Finally, postmortem studies have identified abnormalities of glutamate receptor expression and subunit composition in multiple limbic brain regions implicated in schizophrenia, including the thalamus (15), prefrontal cortex (19, 23, 24), and temporal lobe structures (25–28). Together, these findings suggest that a perturbation of NMDA receptor-mediated glutamate neurotransmission in the thalamus and other limbic structures may contribute to the pathophysiology of schizophrenia.
The NMDA receptor is a multimeric ligand-gated ion channel formed by subunits encoded by seven genes: NR1, NR2A-D, and NR3A-B(29, 30). NR1 is an obligate subunit expressed as one of eight isoforms, due to the alternative splicing of exons 5, 21, and 22 (29). Exon 5 encodes an N-terminal cassette that influences receptor desensitization and sensitivity to protons, zinc ions, and polyamines (31). Exons 21 and 22 encode two C-terminal cassettes (C1 and C2). These C-terminal cassettes modulate NMDA receptor function by influencing the association of NMDA receptor subunits with each other (32) and with other intracellular molecules, including protein kinase C (33), calmodulin (34), and postsynaptic density-associated proteins like PSD95 (35, 36). These intracellular molecules modulate NMDA receptor activity and participate in signal transduction pathways. Consequently, alternative splicing of NR1 has a substantial impact on NMDA receptor structure, function, and related intracellular signaling.
Glutamate neurotransmission involves not only glutamate receptors but also intracellular molecules enriched in postsynaptic density proteins. PSD95, the prototype of this family, and related proteins contain domains that bind the C-termini of NMDA receptor subunits, cytoskeletal proteins, and signal transduction enzymes (Figure 1). Through protein-protein interactions, PSD95 promotes clustering and anchoring of the NMDA receptor at the postsynaptic density (35), modulates NMDA receptor sensitivity to glutamate (37), and, perhaps most importantly, assembles signaling complexes that coordinate NMDA receptor-related intracellular processes (38). An alteration of the expression of PSD95-like molecules, or of the interaction between NMDA receptor subunits and these proteins, can impede NMDA receptor–mediated intracellular signaling. To date, two studies have examined the expression of PSD95 in schizophrenia and reported increased mRNA expression in the occipital cortex (24) and decreased expression in Brodmann’s area 9 of the prefrontal cortex (39) but no change in area A6 of the prefrontal cortex (24) or hippocampus (39).
We have reported alterations of glutamatergic molecules in the thalamus in schizophrenia (15–17), including decreased expression of NR1 subunit transcripts and decreased binding to the polyamine and glycine sites of the NMDA receptor, with the most prominent changes occurring in nuclei reciprocally linked to limbic regions (15). In the present study, we hypothesized that this reduction of NR1 subunits is specifically associated with particular isoforms and used in situ hybridization to determine transcript expression for exon 5-, 21-, and 22-containing NR1 isoforms in the thalamus. We also determined whether the expression of the PSD95 family of molecules is altered in the thalamus in schizophrenia by using in situ hybridization to measure the transcript expression of a set of NMDA receptor-associated postsynaptic density proteins in these same subjects.
Method
Thirteen subjects with schizophrenia and eight nonpsychiatrically ill individuals from the Mount Sinai Medical Center Brain Bank were studied (Table 1). Next of kin consent was obtained for each subject. There were no significant differences between the diagnostic groups in age (t=1.59, df=19, p=0.12), postmortem interval (t=1.18, df=19, p=0.25), or sex distribution (χ2=1.68, df=1, p=0.19). Each schizophrenia and comparison brain underwent neuropathological examination, and Alzheimer’s disease was specifically ruled out in each case. Brains were prepared by slicing one hemisphere into 1-cm coronal slabs, which were snap frozen. Blocks containing thalamus were cryostat-sectioned (20 μm thick), thaw-mounted onto poly-L-lysine-subbed microscope slides, dried, and stored at –80°C. Two slides per subject were prepared for in situ hybridization for each probe.
Antisense oligodeoxyribonucleotides were synthesized for NR1 exons 5 (corresponding bases 1022-1066), 21 (3152-3196), and 22 (3258-3302) to use as in situ hybridization probes. Regions of minimal homology to other genes were identified and verified through a search of Genbank and EMBL databases, using the National Center for Biotechnology Information BLAST network service. Antisense oligonucleotides were purified by high-performance liquid chromatography, diluted to a concentration of 1 μg/μL and stored at –20°C. Oligonucleotides were terminally labeled with 50 μCi of [35S]dATP (New England Nuclear, Boston) with 1.5-μl terminal deoxynucleotidyl transferase (Boehringer-Mannheim, Mannheim, Germany) and used for in situ hybridization as we have previously described in an earlier report examining the distribution of these exons in macaque thalamus (40).
We performed in situ hybridization with riboprobes to determine the thalamic expression of transcripts encoding the NMDA receptor-related postsynaptic density proteins NF-L, PSD93, PSD95, SAP102, and Yotiao. We amplified unique regions of NF-L (Genbank accession number: X05608, nucleotide coding region: 456-950), PSD93 (NM_001364, 587-1082), PSD95 (U83192, 1254-1744), SAP102 (U49089, 204-713), and Yotiao (5032230, 3652-4207) from a human brain cDNA library (EdgeBiosystems, Gaithersburg, Md.) by using polymerase chain reaction (PCR). Amplified cDNA segments were extracted (QIAquick Gel Extraction Kit, Qiagen, Valencia, Calif.), subcloned (Zero Blunt TOPO PCR cloning kit, Invitrogen, Carlsbad, Calif.), and confirmed by nucleotide sequencing (Thermo Sequenase Radiolabeled Termination Cycle Sequencing Kit, USB, Cleveland). Linearized subclones were used to synthesize [35S]UTP-labeled riboprobes, as we have previously described (40).
To ensure the specificity of in situ hybridization signals, multiple control studies were performed. For the NR1 splice variants, adjacent slides were incubated with either labeled probe for exon 5, 21, or 22, or labeled probe in the presence of 100-fold excess of unlabeled oligonucleotide. For the postsynaptic density proteins, adjacent sections were incubated with sense strand probes and processed as previously described (40). One set of these slides was exposed to Kodak film for 7–60 days, and a second set was dipped in Kodak NTB-2 emulsion and stored at 4°C for 21–90 days prior to developing (41).
Film-based images were analyzed for mRNA expression on a nucleus-by-nucleus basis. As we have previously described (15), we identified six discrete thalamic nuclei in each section: anterior, dorsomedial, central medial, ventral anterior, reticular, and pooled ventral tier nuclei. The nuclei were identified based on cellular and white matter patterns, defined by cresyl violet and gold chloride staining of sections from each subject (15). Tissue background values from adjacent white matter were subtracted from grayscale values for each nucleus and converted to optical density. Data from the NMDA receptor isoform study are expressed as optical density values (Table 2). Postsynaptic density protein data are expressed as mRNA concentrations. The amount of radioactivity bound (in nCi/g) was determined by using [14C] microscale standards (Amersham Biosciences, Piscataway, N.J.) (42), which were exposed on the same film as the slides for each study. The number of labeled uridine nucleotides contained in each riboprobe and the specific activity of the [35S]UTP were then used to convert bound radioactivity to concentration of mRNA per nucleus, expressed as fmol/g. For all studies, values for each nucleus from two sections per subject were averaged and used for statistical analysis, which was performed for each probe by two-way analysis of variance, with nucleus and diagnosis as independent variables, and optical density values (exon studies) or mRNA concentrations (postsynaptic density protein studies) as the dependent variables. The Kolmogorov-Smirnov test was used to ensure normality of all data. Analysis of covariance was performed with age and postmortem interval as covariates, and Pearson product-moment correlations were used to determine relationships between continuously distributed variables. For all tests, alpha=0.05.
Results
NMDA Receptor NR1 Isoform Expression
NMDA receptor NR1 isoform transcripts containing exons 5, 21, and 22 were expressed in all thalamic nuclei (Figure 2 and Table 2). Exon 22-containing transcripts were the most abundant, with relatively lower levels of exon 5 and 21 transcripts detected. Expression of exon 22-containing isoforms was significantly decreased in the thalamus of the schizophrenia subjects (F=4.34, df=1, 114, p<0.04). The expression of NR1 isoforms containing exons 5 and 21 were not significantly different between groups. There were no significant diagnosis-by-nucleus interactions for any of the three isoforms. Correlation analysis revealed that the expression levels of each splice variant correlated with neither age (r=–0.19 to 0.20, p=0.38–0.57) nor postmortem interval (r=–0.14 to 0.29, p=0.20–0.88).
mRNA Expression of Postsynaptic Density Proteins
Transcripts encoding NF-L, PSD95, SAP102, and PSD93 were expressed in all nuclei studied (Figure 3), but negligible levels of Yotiao mRNA were found (data not shown). NF-L transcripts were abundantly expressed throughout the thalamus. PSD95 transcripts were also abundant and were enriched in the dorsal and anterior nuclei. There were moderate levels of SAP102 transcripts but low levels of PSD93. NF-L mRNA expression was 23% higher in the thalamus in schizophrenia subjects (Figure 4). There was a 21% increase in PSD95 transcripts in schizophrenia subjects and a 32% increase in SAP102 transcripts (Figure 4). Expression of PSD93 mRNA was not significantly different between groups. We did not detect significant diagnosis-by-nucleus interactions for any of the postsynaptic density protein transcripts. The inclusion of age and postmortem interval as covariates in our analyses did not alter these results for any molecule. Correlation analysis showed that postsynaptic density protein transcripts did not correlate significantly with either age (r=–0.38 to 0.18, p=0.09–0.97) or postmortem interval (r=–0.29 to 0.28, p=0.19–0.82).
In Situ Hybridization Control Experiments
For each of the NR1 splice variant studies, no specific hybridization was observed in thalamic tissue incubated with labeled oligonucleotides for each exon in the presence of excess unlabeled probe (Figure 2). Similarly, in the postsynaptic density protein experiments, no signal was observed in tissue hybridized with sense strand riboprobes (Figure 3). Emulsion-dipped slides that had been incubated with antisense strand probes for each postsynaptic density protein demonstrated the cellular localization of these transcripts in the thalamus (Figure 3). NF-L transcripts were primarily concentrated in large glutamatergic relay neurons (Figure 3). PSD95 transcripts were present in both relay cells and small GABAergic interneurons (Figure 3). SAP102 transcripts were expressed in numerous cells in the thalamus, while PSD93 mRNA expression was quite sparse (Figure 3).
Discussion
We recently reported alterations in the expression of NMDA receptor subunits NR1 and NR2C and other molecules involved in glutamatergic neurotransmission in the thalamus of subjects with schizophrenia (15–17). In the present study, using the same subjects that were used in these earlier studies, we have characterized the thalamic expression of NR1 subunit isoforms in schizophrenia and found that this reduction is restricted to exon 22-containing isoforms. Moreover, we have examined the expression of postsynaptic density proteins that associate with NMDA receptor subunits and found a significant increase in the transcript expression of the NR1-associated protein NF-L and NR2-associated proteins PSD95 and SAP102.
The NMDA NR1 subunit is obligate, and numerous studies have indicated that alternative splicing of the N- and C-terminal cassettes produces functionally distinct NMDA channels (31). Our results are consistent with previous reports of NR1 splice variant expression patterns in the rodent, macaque, and human brain (40, 43–45). Exon 5-containing isoforms are generally rare but are expressed at low to moderate levels in the rodent (45) and macaque thalamus (40). Moderate to high levels of expression of exon 22-containing isoforms have been reported in the cortex, hippocampus, striatum, and thalamus of rat (45) and macaque (40). Our results coincide with this work, since we found modest levels of exon 5-containing isoforms and relatively high levels of exon 22-containing isoforms in the human thalamus, consistent with the relative expression of these exons in macaque (40).
Altered NR1 subunit isoforms may reflect changes in NMDA receptor subunit composition, which in turn may have significant functional consequences, since alternative splicing of exons 5, 21, and 22 can modulate NMDA receptor localization and function (33, 34, 46). Most studies have focused on the functional significance of the exon 5-encoded N1 cassette, the exon 21-encoded C1 cassette, or the C2′ cassette, which is a unique C-terminal tail that is produced when the exon 22-encoded C2 cassette is excluded. Omission of exon 5 modifies NMDA receptor desensitization and its sensitivity to protons, zinc ions, and polyamines (31). Alternative splicing of the C1 cassette reduces protein kinase C potentiation of NMDA receptor activity (46, 47) and also influences the receptor’s intracellular distribution (48) and cell surface expression (49). NR1 isoforms that contain both C1 and C2 cassettes are expressed at low levels on the cell membrane in vitro, whereas isoforms that contain only the C2′ domain are highly expressed (49). It is of interest that the C2′ cassette contains a PDZ-binding sequence that is thought to be involved in trafficking the NMDA receptor to the synaptic membrane (50, 51), suggesting that NR1 isoforms differentially interact with postsynaptic density proteins and other cytoskeletal components to target the NMDA receptor to the postsynaptic density proteins.
We observed a specific reduction of NR1 isoform transcripts containing exon 22 in the thalamus in schizophrenia. We did not detect a change in the expression of exon 5 or 21 transcripts. Because of the low expression levels of these two isoforms, however, our inability to find a further decrease of one or both in schizophrenia may be a floor effect. Given the paucity of information regarding the functional role of the exon 22-encoded C2 cassette, it is difficult to interpret what effect would result from decreased expression of exon 22-containing isoforms. When the C2 cassette is excluded, it is replaced by the C2′ cassette, so perhaps downregulating the expression of the C2 cassette results in greater numbers of isoforms with C2′, which are more likely to be transported to the cell surface (49).
PSD95 and similar NR2-associated proteins, SAP102 and PSD93, contain domains that bind numerous intracellular molecules, including the C-termini of NR2 subunits, cytoskeletal proteins, and signal transduction enzymes (38), which influence NMDA receptor function and NMDA receptor-associated intracellular processes (38, 52). We found a significant increase in the transcript expression of PSD95 and SAP102, but not PSD93, in the thalamus in subjects with schizophrenia. The total pool of postsynaptic density proteins may be increased in schizophrenia in an attempt to compensate for decreased thalamic expression of NMDA receptors. Enhanced expression of postsynaptic density molecules may lead to an enhanced association of the postsynaptic density proteins with the remaining NMDA receptor subunits, an intracellular adaptation to attempt to maintain homeostasis of NMDA receptor-related intracellular signaling in the face of decreased NMDA receptor expression or in response to a general deficit in glutamate neurotransmission.
Widespread interest in the NR2 subunit-associated PSD95 family of proteins has prompted the investigation of intracellular proteins that interact with the NR1 subunit (53, 54). Two proteins, NF-L (53) and Yotiao (54), have been identified as proteins that interact with exon 21-containing isoforms. NF-L, along with the two other neurofilament subunits, NF-heavy and NF-medium, are among the most abundant cytoskeletal elements and play an important role in the maintenance of neuronal structure (55). NF-L may also be involved in directing NMDA receptors to the postsynaptic density or linking it to the synaptic cytoskeleton (48, 53). NF-L interacts with protein phosphatase-1 (PP1), a major protein/serine/threonine phosphatase that is involved in numerous intracellular processes (56). Although the functional significance of this NF-L/PP1 interaction is not fully understood, it has been suggested that NF-L may bind PP1 and position it to dephosphorylate other postsynaptic density proteins, such as NMDA receptor subunits, or CamKII (57).
We found that NF-L mRNA expression was significantly elevated in the thalamus in subjects with schizophrenia. Although a portion of NF-L protein in the cell may interact with the NR1 subunit to participate in NMDA receptor function, the majority of NF-L associates with the other neurofilament subunits to maintain the neuronal cytoskeleton. It is not possible to determine if the elevated NF-L mRNA we found in schizophrenia subjects translates into greater amounts of NF-L protein interacting with NMDA receptors, which might serve to boost an impaired glutamatergic system, or if it is part of a more general cytoskeletal response to the illness.
Our study has several limitations. These data are from elderly subjects, so generalization of our findings to younger patients should be made with caution. A limitation of all postmortem studies in schizophrenia is the possible effect of chronic neuroleptic treatment, since these medications potentially regulate the systems under study. While antipsychotic medications have been shown to alter thalamic metabolism and immediate early gene expression (58–60), they do not appear to affect thalamic NMDA receptor expression (61). To date, no studies have directly examined the effect of neuroleptics on the expression of NMDA receptor-related postsynaptic density molecules. However, Dracheva and colleagues (24) reported altered PSD95 and NMDA receptor subunit expression in the prefrontal and occipital cortices in schizophrenia and found that these transcripts did not differ between patients who were taking antipsychotic medication within 6 weeks of death and those who were medication-free for greater than 6 weeks (24).
Numerous studies support a hypothesis of glutamatergic dysfunction in schizophrenia. While there are reports of abnormal ionotropic glutamate receptor expression in various limbic regions in schizophrenia, the pathophysiology of this illness may not be solely associated with abnormal receptor expression. Rather, it may also involve abnormalities of the intracellular signaling machinery that is linked to the NMDA receptor. The recently identified PSD95 family of molecules represents an important link between the NMDA receptor and the intracellular enzymes that mediate NMDA receptor-associated signaling. Abnormal expression of these postsynaptic density proteins, or an alteration of the interaction between NMDA receptors and these proteins, may significantly impact NMDA receptor-associated signal transduction. In this study, we show that three of these NMDA receptor-associated postsynaptic density proteins are abnormally expressed in the thalamus in schizophrenia. These data provide further evidence of glutamatergic dysfunction in schizophrenia and suggest that glutamatergic abnormalities in this illness may occur not only at the level of receptor expression but also within intracellular pathways associated with glutamate receptor-associated signal transduction.
![]() |
![]() |
Presented in part at the 56th annual meeting of the Society of Biological Psychiatry, New Orleans, May 3–5, 2001. Received Aug. 15, 2001; revisions received Aug. 17 and Nov. 1, 2002; accepted Dec. 3, 2002. From the Department of Psychiatry and Mental Health Research Institute, University of Michigan Medical School; and the Department of Psychiatry, Mount Sinai School of Medicine, New York. Address reprint requests to Ms. Clinton, Mental Health Research Institute and Department of Psychiatry, University of Michigan, 205 Zina Pitcher Place, Ann Arbor, MI 48109-0720; [email protected] (e-mail). Supported by NIMH grant MH-53327 and a National Alliance for Research on Schizophrenia and Depression Independent Investigator Award to Dr. Meador-Woodruff, a Veterans Merit Review Award to Dr. Haroutunian, and grants from NIMH (MH-45212) and the National Institute on Aging (AG-5138 and AG-2219) to Dr. Davis.
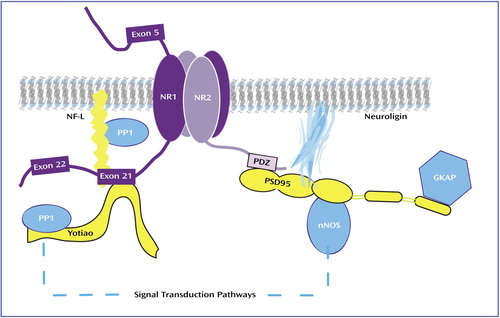
Figure 1. NMDA Receptor and Associated Postsynaptic Density Molecules Embedded in the Postsynaptic Membranea
aThe postsynaptic density proteins PSD95, SAP102, and PSD93 contain regions that bind the NR2 C-terminus and other intracellular molecules such as neuronal nitric oxide synthase (nNOS) and guanylate kinase-associated protein (GKAP). These proteins link the NMDA receptor to signal transduction pathways. The postsynaptic density proteins NF-L and Yotiao interact with exon 21-containing NR1 subunits, which link the receptor to the cytoskeleton and the signal transduction enzyme protein phosphatase-1 (PP1).
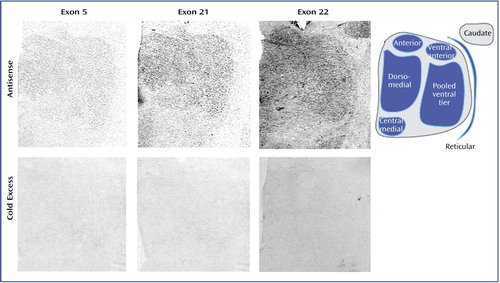
Figure 2. Transcript Expression of NMDA Receptor NR1 Isoforms Containing Exon 5, 21, or 22a
aThe antisense row shows representative sections labeled with radiolabeled oligonucleotide probes for each exon, and the cold excess row shows adjacent sections incubated with excess unlabeled oligonucleotide. The tail of the caudate nucleus is readily apparent in the case of exon 22.
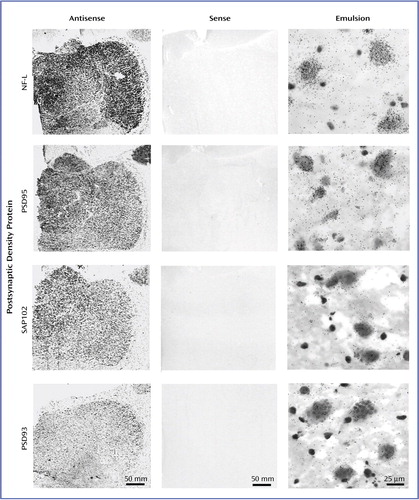
Figure 3. Transcript Expression of NMDA Receptor-Related Postsynaptic Density Proteinsa
aThe antisense and sense columns show film autoradiograms of adjacent sections treated with either antisense or sense strand riboprobes for each transcript. The emulsion column shows bright-field micrographs of emulsion-dipped slides labeled for each transcript, demonstrating cellular localization within the dorsomedial nucleus for each probe. NF-L transcripts were abundant and primarily expressed in large glutamatergic relay neurons. PSD95 transcripts were present in both relay cells and small GABAergic interneurons. SAP102 transcripts were expressed primarily in large glutamatergic cells. PSD93 transcripts were expressed at low levels.
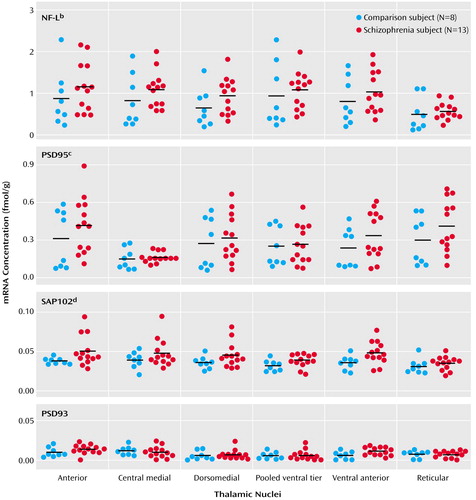
Figure 4. Thalamic Expression of NMDA Receptor-Associated Postsynaptic Density Protein Transcripts in Schizophrenia (N=13) and Comparison (N=8) Subjectsa
aHorizontal bars represent mean values.
bSignificantly higher mRNA expression in the schizophrenia subjects (F=5.14, df=1, 114, p<0.03).
cSignificantly higher mRNA expression in the schizophrenia subjects (F=3.99, df=1, 114, p<0.05).
dSignificantly higher mRNA expression in the schizophrenia subjects (F=11.09, df=1, 114, p=0.001).
1. Jones EG: Cortical development and thalamic pathology in schizophrenia. Schizophr Bull 1997; 23:483-501Crossref, Medline, Google Scholar
2. Andreasen NC, Paradiso S, O’Leary DS: “Cognitive dysmetria” as an integrative theory of schizophrenia: a dysfunction in cortical-subcortical-cerebellar circuitry? Schizophr Bull 1998; 24:203-218Crossref, Medline, Google Scholar
3. Andreasen NC, O’Leary DS, Cizadlo T, Arndt S, Rezai K, Boles Ponto LL, Watkins GL, Hichwa RD: Schizophrenia and cognitive dysmetria: a positron-emission tomography study of dysfunctional prefrontal-thalamic-cerebellar circuitry. Proc Natl Acad Sci USA 1996; 93:9985-9990Crossref, Medline, Google Scholar
4. Andreasen NC, Arndt S, Swayze V II, Cizadlo T, Flaum M, O’Leary D, Ehrhardt JC, Yuh WTC: Thalamic abnormalities in schizophrenia visualized through magnetic resonance image averaging. Science 1994; 266:294-298Crossref, Medline, Google Scholar
5. Buchsbaum MS, Someya T, Teng CY, Abel L, Chin S, Najafi A, Haier RJ, Wu J, Bunney WE Jr: PET and MRI of the thalamus in never-medicated patients with schizophrenia. Am J Psychiatry 1996; 153:191-199Link, Google Scholar
6. Gilbert AR, Rosenberg DR, Harenski K, Spencer S, Sweeney JA, Keshavan MS: Thalamic volumes in patients with first-episode schizophrenia. Am J Psychiatry 2001; 158:618-624Link, Google Scholar
7. Gur RE, Maany V, Mozley PD, Swanson C, Bilker W, Gur RC: Subcortical MRI volumes in neuroleptic-naive and treated patients with schizophrenia. Am J Psychiatry 1998; 155:1711-1717Link, Google Scholar
8. Tamminga CA, Thaker GK, Buchanan R, Kirkpatrick B, Alphs LD, Chase TN, Carpenter WT: Limbic system abnormalities identified in schizophrenia using positron emission tomography with fluorodeoxyglucose and neocortical alterations with deficit syndrome. Arch Gen Psychiatry 1992; 49:522-530Crossref, Medline, Google Scholar
9. Hazlett EA, Buchsbaum MS, Byne W, Wei T-C, Spiegel-Cohen J, Geneve C, Kinderlehrer R, Haznedar MM, Shihabuddin L, Siever LJ: Three-dimensional analysis with MRI and PET of the size, shape, and function of the thalamus in the schizophrenia spectrum. Am J Psychiatry 1999; 156:1190-1199Abstract, Google Scholar
10. Silbersweig DA, Stern E, Frith C, Cahill C, Holmes A, Grootoonk S, Seaward J, McKenna P, Chua SE, Schnorr L, et al: A functional neuroanatomy of hallucinations in schizophrenia. Nature 1995; 378:176-179Crossref, Medline, Google Scholar
11. Byne W, Buchsbaum MS, Kemether E, Hazlett EA, Shinwari A, Mitropoulou V, Siever LJ: Magnetic resonance imaging of the thalamic mediodorsal nucleus and pulvinar in schizophrenia and schizotypal personality disorder. Arch Gen Psychiatry 2001; 58:133-140Crossref, Medline, Google Scholar
12. Pakkenberg B: Pronounced reduction of total neuron number in mediodorsal thalamic nucleus and nucleus accumbens in schizophrenics. Arch Gen Psychiatry 1990; 47:1023-1028Crossref, Medline, Google Scholar
13. Popken GJ, Bunney WE Jr, Potkin SG, Jones EG: Subnucleus-specific loss of neurons in medial thalamus of schizophrenics. Proc Natl Acad Sci USA 2000; 97:9276-9280Crossref, Medline, Google Scholar
14. Danos P, Baumann B, Bernstein HG, Franz M, Stauch R, Northoff G, Krell D, Falkai P, Bogerts B: Schizophrenia and anteroventral thalamic nucleus: selective decrease of parvalbumin-immunoreactive thalamocortical projection neurons. Psychiatry Res 1998; 82:1-10Crossref, Medline, Google Scholar
15. Ibrahim HM, Hogg AJ Jr, Healy DJ, Haroutunian V, Davis KL, Meador-Woodruff JH: Ionotropic glutamate receptor binding and subunit mRNA expression in thalamic nuclei in schizophrenia. Am J Psychiatry 2000; 157:1811-1823Link, Google Scholar
16. Smith RE, Haroutunian V, Davis KL, Meador-Woodruff JH: Expression of excitatory amino acid transporter transcripts in the thalamus of subjects with schizophrenia. Am J Psychiatry 2001; 158:1393-1399Link, Google Scholar
17. Smith RE, Haroutunian V, Davis KL, Meador-Woodruff JH: Vesicular glutamate transporter transcript expression in the thalamus in schizophrenia. Neuroreport 2001; 12:2885-2887Crossref, Medline, Google Scholar
18. Popken GJ, Leggio MG, Bunney WE, Jones EG: Expression of mRNAs related to the GABAergic and glutamatergic neurotransmitter systems in the human thalamus: normal and schizophrenic. Thalamus & Related Systems 2002; 1:349-369Google Scholar
19. Meador-Woodruff JH, Healy DJ: Glutamate receptor expression in schizophrenic brain. Brain Res Brain Res Rev 2000; 31:288-294Crossref, Medline, Google Scholar
20. Goff DC, Coyle JT: The emerging role of glutamate in the pathophysiology and treatment of schizophrenia. Am J Psychiatry 2001; 158:1367-1377Link, Google Scholar
21. Lahti A, Holcomb H, Medoff D, Tamminga C: Ketamine activates psychosis and alters limbic blood flow in schizophrenia. Neuroreport 1995; 6:869-872Crossref, Medline, Google Scholar
22. Javitt DC, Zylberman I, Zukin SR, Heresco-Levy U, Lindenmayer JP: Amelioration of negative symptoms in schizophrenia by glycine. Am J Psychiatry 1994; 151:1234-1236Link, Google Scholar
23. Akbarian S, Sucher NJ, Bradley D, Tafazzoli A, Trinh D, Hetrick WP, Potkin SG, Sandman CA, Bunney WE Jr, Jones EG: Selective alterations in gene expression for NMDA receptor subunits in prefrontal cortex of schizophrenics. J Neurosci 1996; 16:19-30Crossref, Medline, Google Scholar
24. Dracheva S, Marras SAE, Elhakem SL, Kramer FR, Davis KL, Haroutunian V: N-Methyl-d-aspartic acid receptor expression in the dorsolateral prefrontal cortex of elderly patients with schizophrenia. Am J Psychiatry 2001; 158:1400-1410; correction, 158:2107Link, Google Scholar
25. Dean B, Scarr E, Bradbury R, Copolov D: Decreased hippocampal (CA3) NMDA receptors in schizophrenia. Synapse 1999; 32:67-69Crossref, Medline, Google Scholar
26. Grimwood S, Slater P, Deakin JF, Hutson PH: NR2B-containing NMDA receptors are up-regulated in temporal cortex in schizophrenia. Neuroreport 1999; 10:461-465Crossref, Medline, Google Scholar
27. Le Corre S, Harper CG, Lopez P, Ward P, Catts S: Increased levels of expression of an NMDARI splice variant in the superior temporal gyrus in schizophrenia. Neuroreport 2000; 11:983-986Crossref, Medline, Google Scholar
28. Gao X-M, Sakai K, Roberts RC, Conley RR, Dean B, Tamminga CA: Ionotropic glutamate receptors and expression of N-methyl-d-aspartate receptor subunits in subregions of human hippocampus: effects of schizophrenia. Am J Psychiatry 2000; 157:1141-1149Link, Google Scholar
29. Hollmann M, Heinemann S: Cloned glutamate receptors. Annu Rev Neurosci 1994; 17:31-108Crossref, Medline, Google Scholar
30. Das S, Sasaki YF, Rothe T, Premkumar LS, Takasu M, Crandall JE, Dikkes P, Conner DA, Rayudu PV, Cheung W, Chen HS, Lipton SA, Nakanishi N: Increased NMDA current and spine density in mice lacking the NMDA receptor subunit NR3A. Nature 1998; 393:377-381Crossref, Medline, Google Scholar
31. Zukin R, Bennett M: Alternatively spliced isoforms of the NMDARI receptor subunit. Trends Neurosci 1995; 18:306-313Crossref, Medline, Google Scholar
32. Sheng M, Cummings J, Roldan L, Jan Y, Jan L: Changing subunit composition of heteromeric NMDA receptors during development of rat cortex. Nature 1994; 368:144-147Crossref, Medline, Google Scholar
33. Tingley W, Roche K, Thompson A, Huganir R: Regulation of NMDA receptor phosphorylation by alternative splicing of the C-terminal domain. Nature 1993; 364:70-73Crossref, Medline, Google Scholar
34. Ehlers M, Zhang S, Bernhadt J, Huganir R: Inactivation of NMDA receptors by direct interaction of calmodulin with the NR1 subunit. Cell 1996; 84:745-755Crossref, Medline, Google Scholar
35. Brenman J, Christopherson K, Craven S, McGee A, Bredt D: Cloning and characterization of postsynaptic density 93, a nitric oxide synthase interacting protein. J Neurosci 1996; 16:7407-7415Crossref, Medline, Google Scholar
36. Lau L, Mammen A, Ehlers M, Kindler S, Chung W, Garner C, Huganir R: Interaction of the N-methyl-d-aspartate receptor complex with a novel synapse-associated protein, SAP102. J Biol Chem 1996; 271:21622-21628Crossref, Medline, Google Scholar
37. Rutter A, Stephenson F: Coexpression of postsynaptic density-95 protein with NMDA receptors results in enhanced receptor expression together with a decreased sensitivity to L-glutamate. J Neurochem 2000; 75:2501-2510Crossref, Medline, Google Scholar
38. Sheng M, Pak D: Ligand-gated ion channel interactions with cytoskeletal and signaling proteins. Annu Rev Physiol 2000; 62:755-778Crossref, Medline, Google Scholar
39. Ohnuma T, Kato H, Arai H, Faull R, McKenna P, Emson P: Gene expression of PSD95 in prefrontal cortex and hippocampus in schizophrenia. Neuroreport 2000; 11:3133-3137Crossref, Medline, Google Scholar
40. Clinton SM, Meador-Woodruff JH: Nucleus-specific expression of NMDA receptor-associated postsynaptic density proteins in primate thalamus. Thalamus & Related Systems 2002; 1:303-316Google Scholar
41. Meador-Woodruff JH, Mansour A, Bunzow JR, Van Tol HH, Watson SJ Jr, Civelli O: Distribution of D2 dopamine receptor mRNA in rat brain. Proc Natl Acad Sci USA 1989; 86:7625-7628Crossref, Medline, Google Scholar
42. Miller JA: The calibration of 35S or 32P with 14C-labeled brain paste or 14C-plastic standards for quantitative autoradiography using LKB Ultrofilm or Amersham Hyperfilm. Neurosci Lett 1991; 121:211-214Crossref, Medline, Google Scholar
43. Standaert DG, Testa CM, Young AB, Penney JB Jr: Organization of N-methyl-d-aspartate glutamate receptor gene expression in the basal ganglia of the rat. J Comp Neurol 1994; 343:1-16Crossref, Medline, Google Scholar
44. Meoni P, Bunnemann BH, Trist DG, Bowery NG: N-terminal splice variants of the NMDAR1 glutamate receptor subunit: differential expression in human and monkey brain. Neurosci Lett 1998; 249:45-48Crossref, Medline, Google Scholar
45. Laurie DJ, Seeburg PH: Regional and developmental heterogeneity in splicing of the rat brain NMDAR1 mRNA. J Neurosci 1994; 14(5, part 2):3180-3194Google Scholar
46. Logan S, Rivera F, Leonard J: Protein kinase C modulation of recombinant NMDA receptor currents: roles for the C-terminal C1 exon and calcium ions. J Neurosci 1999; 19:974-986Crossref, Medline, Google Scholar
47. Zheng X, Zhang L, Wang AP, Bennett MV, Zukin RS: Protein kinase C potentiation of N-methyl-d-aspartate receptor activity is not mediated by phosphorylation of N-methyl-d-aspartate receptor subunits. Proc Natl Acad Sci USA 1999; 96:15262-15267Crossref, Medline, Google Scholar
48. Ehlers MD, Tingley WG, Huganir RL: Regulated subcellular distribution of the NR1 subunit of the NMDA receptor. Science 1995; 269:1734-1737Crossref, Medline, Google Scholar
49. Okabe S, Miwa A, Okado H: Alternative splicing of the C-terminal domain regulates cell surface expression of the NMDA receptor NR1 subunit. J Neurosci 1999; 19:7781-7792Crossref, Medline, Google Scholar
50. Standley S, Roche KW, McCallum J, Sans N, Wenthold RJ: PDZ domain suppression of an ER retention signal in NMDA receptor NR1 splice variants. Neuron 2000; 28:887-898Crossref, Medline, Google Scholar
51. Scott DB, Blanpied TA, Swanson GT, Zhang C, Ehlers MD: An NMDA receptor ER retention signal regulated by phosphorylation and alternative splicing. J Neurosci 2001; 21:3063-3072Crossref, Medline, Google Scholar
52. Migaud M, Charlesworth P, Dempster M, Webster LC, Watabe AM, Makhinson M, He Y, Ramsay MF, Morris RG, Morrison JH, O’Dell TJ, Grant SG: Enhanced long-term potentiation and impaired learning in mice with mutant postsynaptic density-95 protein. Nature 1998; 396:433-439Crossref, Medline, Google Scholar
53. Ehlers M, Fung E, O’Brien R, Huganir R: Splice variant-specific interaction of the NMDA receptor subunit NR1 with neuronal intermediate filaments. J Neurosci 1998; 18:720-730Crossref, Medline, Google Scholar
54. Lin J, Wyszynski M, Madhavan R, Sealock R, Kim J, Sheng M: Yotiao, a novel protein of neuromuscular junction and brain that interacts with specific splice variants of NMDA receptor subunit NR1. J Neurosci 1998; 18:2017-2027Crossref, Medline, Google Scholar
55. Xu Z, Dong DL, Cleveland DW: Neuronal intermediate filaments: new progress on an old subject. Curr Opin Neurobiol 1994; 4:655-661Crossref, Medline, Google Scholar
56. Shenolikar S: Protein serine/threonine phosphatases—new avenues for cell regulation. Annu Rev Cell Biol 1994; 10:55-86Crossref, Medline, Google Scholar
57. Terry-Lorenzo R, Inoue M, Connor J, Haystead T, Armbruster B, Gupta R, Oliver C, Shenolikar S: Neurofilament-L is a protein phosphatase-1-binding protein associated with neuronal plasma membrane and post-synaptic density. J Biol Chem 2000; 275:2439-2446Crossref, Medline, Google Scholar
58. Cohen BM, Wan W: The thalamus as a site of action of antipsychotic drugs. Am J Psychiatry 1996; 153:104-106Link, Google Scholar
59. Holcomb HH, Cascella NG, Thaker GK, Medoff DR, Dannals RF, Tamminga CA: Functional sites of neuroleptic drug action in the human brain: PET/FDG studies with and without haloperidol. Am J Psychiatry 1996; 153:41-49Link, Google Scholar
60. Deutch A, Ongur D, Duman R: Antipsychotic drugs induce Fos protein in the thalamic paraventricular nucleus: a novel antipsychotic drug action. Neuroscience 1995; 66:337-346Crossref, Medline, Google Scholar
61. Ulas J, Nguyen L, Cotman C: Chronic haloperidol treatment enhances binding to NMDA receptors in rat cortex. Neuroreport 1993; 4:1049-1051Crossref, Medline, Google Scholar