Central Serotonin Transporter Availability Measured With [123I]β-CIT SPECT in Relation to Serotonin Transporter Genotype
Abstract
OBJECTIVE: A functional polymorphism has been described in the promoter region of the gene (SLC6A4) coding for the serotonin transporter protein (SERT). This polymorphism has two common alleles, designated as long and short. Each allele has been linked with a number of human clinical phenotypes, including neuropsychiatric diseases associated with dysregulation of serotonin transmission. In vitro studies of nonneural cells have suggested that the long allele may have higher transcriptional activity than the short allele. However, the relevance of these findings for SERT levels in the brain remains unclear. METHOD: The authors assessed genotypes at the SLC6A4 promoter polymorphism in 96 healthy European American subjects who underwent single photon emission computed tomography scanning with [123I]2β-carbomethoxy-3β-(4-iodophenyl) tropane ([123I]β-CIT) for measurement of central SERT availability. A ratio of specific to nondisplaceable brain uptake (i.e., V3′′=[brainstem-diencephalon – occipital]/occipital), a measure proportional to the binding potential (Bmax/KD), was derived. RESULTS: The results showed that the main effect of genotype was significant. Post hoc Tukey pairwise comparisons revealed that the short-short homozygotes had significantly greater SERT availability than the long-short heterozygotes. There was a nonsignificant tendency for the long-long homozygotes to have greater SERT availability than the heterozygotes, but no difference was observed between the long-long homozygotes and the short-short homozygotes. The effect of age was significant in the analysis of covariance model. CONCLUSIONS: These results do not suggest higher central SERT levels in association with the long allele in European American subjects but point to a more complex relationship between SLC6A4 genotype and protein availability.
A functional polymorphism has been described in the 5′ flanking regulatory (promoter) region of the gene (SLC6A4) coding for the serotonin transporter protein (SERT). This polymorphism (5-HTTLPR) has two common alleles that have been designated as long (16 repeats) and short (14 repeats), according to their relative size (1).
The 5-HTTLPR polymorphism has been associated with a number of clinical phenotypes and diseases (for a review, see reference 2). The following are selected from numerous previous reports. Healthy subjects who carry the short allele have been reported to have higher scores on measures of anxiety-related personality traits (1), although this has not been observed uniformly (e.g., reference 3). Conversely, the long allele has been associated with depression and suicide (4), and among patients with geriatric depression, long-long homozygotes have been observed to have more severe depressive symptoms and higher platelet factor 4 and ß-thromboglobulin levels than those with short carriers (5). The long allele also has been reported to be in linkage disequilibrium with obsessive-compulsive disorder (6). Short carriers have been reported to have a higher risk of bipolar disorder (7, 8) and antidepressant-induced mania in bipolar disorder (9). Susceptibility to alcoholism has yielded contradictory associations with 5-HTTLPR, including no effect (10, 11), a greater risk for long-long homozygotes (12), and a greater risk for short carriers (13). Among alcoholic patients, short carriers also may have a higher risk of suicide attempts (14). Finally, two studies have suggested that the short allele is a risk factor for late-onset Alzheimer’s disease (15, 16). The clinical associations of the 5-HTTLPR polymorphism are far from established, as reports for most psychiatric phenotypes have been inconsistent, a circumstance that may reflect the genetic complexity of these phenotypes and, consequently, the limited power to detect associations with them.
Emerging reports have also considered intermediate phenotypes, or “endophenotypes,” which (since they are thought to reflect phenomena closer to the direct effect of the SLC6A4 variant than a complex behavioral phenotype) have the potential to increase power for observing a phenotypic effect. For example, Moreno et al. (17) reported that the SLC6A4 genotype is related to mood response during tryptophan depletion, and Hariri et al. (18) showed that the SLC6A4 genotype is related to amygdalar response to emotion based on functional magnetic resonance imaging (MRI) measures.
As a possible basis for some genetic-phenotypic associations of the 5-HTTLPR polymorphism, in vitro studies of nonneural cells (1, 19) have suggested that the long allele may have higher transcriptional activity than the short allele. However, thus far, postmortem binding studies have not supported an association between the long allele and increased SERT binding in human brain tissue in mixed patient-comparison groups. Studies to date have reported the following:
1. | Lower SERT binding in the midbrain in long-short heterozygotes than in either long-long or short-short homozygotes among cocaine-dependent and healthy comparison subjects (20). | ||||
2. | Greater SERT binding in the prefrontal cortex in short-short homozygotes than in long carriers among suicide victims and healthy comparison subjects (4). | ||||
3. | No relationship between 5-HTTLPR genotype and SERT binding in the prefrontal cortex in individuals with (N=29) and without (N=71) a history of major depression (21). |
With the advent of functional brain imaging, it is possible to study the effects of genetic polymorphisms on living subjects (18, 22). As an in vivo probe for SERT, several investigators have chosen the single photon emission computed tomography (SPECT) radioligand [123I]2β-carbomethoxy-3β-(4-iodophenyl)tropane ([123I]β-CIT, also referred to as [123I]RTI-55). [123I]β-CIT is a potent cocaine analog with a high affinity in vitro for both SERT and the dopamine transporter (23). Previous studies in humans and nonhuman primates have shown that [123I]β-CIT accumulates in two distinct brain regions: the striatum and the brainstem-diencephalon. Pharmacological characterization of regional [123I]β-CIT uptake in baboons (24) has indicated that striatal activity is associated almost exclusively with the dopamine transporter (i.e., displaceable by GBR-12909 but not citalopram), while binding in the brainstem-diencephalon appears to be specific for SERT (i.e., displaceable by citalopram but not GBR-12909). Thus, regional uptake of [123I]β-CIT in the brainstem-diencephalon appears to provide a pharmacologically relevant measure of SERT in humans.
Two recent neuroimaging investigations with SPECT with [123I]β-CIT have examined the effects of the SLC6A4 5-HTTLPR polymorphism on SERT availability in humans. Heinz et al. (25) studied 14 abstinent alcoholics and eight healthy comparison subjects and found that among healthy subjects, SERT availability was higher for long-long homozygotes than for short carriers, whereas among alcohol-dependent subjects, SERT availability was lower for long-long homozygotes than for short carriers. Jacobsen et al. (26) studied 27 healthy comparison subjects and reported no effect of the SLC6A4 genotype on SERT availability.
The discrepant results in these two studies may be attributable to a number of factors, including the small group size, different outcome measures and image analysis techniques, and diagnostic and population heterogeneity. Therefore, we sought to examine a larger diagnostically and racially homogeneous group. In the present study, the effect of the SLC6A4 5-HTTLPR genotype on SERT protein availability was measured in 96 healthy European American subjects.
Method
Subjects
The study population consisted of 96 healthy European American volunteers (54 male and 42 female) who ranged in age from 18 to 88 years (mean=50, SD=19). The subjects underwent a clinical examination by a research psychiatrist to exclude any neurological or psychiatric disease, and alcohol or substance abuse. Screening procedures included a physical and neurological examination, an ECG, serum chemistries, thyroid function studies, a CBC, a urinalysis, and a urine toxicology screen. Female subjects of childbearing potential were required to have a negative pregnancy test (a serum test at screening, a urine test immediately before tracer injection). The subjects ≥55 years of age (N=31) also were required to have no significant evidence of cognitive impairment, as indicated by a Folstein Mini-Mental State Examination (27) score ≥27. The subjects ≥68 (N=25) were required to have a normal brain MRI scan. No subject was taking medication known to affect the brain serotonin (5-HT) system. After complete description of the study procedures to the subjects, written informed consent was obtained. The present study group is a subset of those (N=126) in whom we previously reported an age-related decline in dopamine transporters (28) and SERTs (29) using the same [123I]β-CIT SPECT scans. It also contains 12 subjects’ scans in common with our earlier [123I]β-CIT SERT genetic report (26). All scans, including these common scans, were analyzed by a different rater from the previous report using somewhat different methods.
DNA Analysis
The SLC6A4 promoter variable number of tandem repeats polymorphism was genotyped as described previously (10). Alleles were designated according to their relative size: short (14 repeats) and long (16 repeats).
SPECT Imaging
All subjects received 0.6 g potassium iodide (a saturated solution of potassium iodide) in the 24 hours before the SPECT scan. They then received an injection of [123I]β-CIT (mean=6.0 mCi, SD=0.7; specific activity >5,000 Ci/mmol) on day 1, followed 22.9 hours later (±1.9 hours) by a 24-minute scan with a PRISM 3000 (N=72) or 3000XP (N=24) SPECT camera (Picker, Cleveland) equipped with a low-energy, high-resolution fan-beam collimator (128×128 matrix, 120° angular range, 3° angular step, 40 steps, 36 seconds per step, 15.5 cm radius of rotation). In this configuration, the PRISM 3000 acquires images at a reconstructed full-width at half maximum resolution of 12.3 mm, as determined by a 123I point source in water. Comparability of the two cameras has previously been confirmed by imaging 26 subjects on both cameras from a single [123I]β-CIT injection (29). Previous studies (30, 31) have demonstrated that [123I]β-CIT reaches equilibrium binding in the brain after 18 to 24 hours, yielding a simple unitless ratio of regional radioactivities (V3′′=specific/nondisplaceable binding=[brainstem-diencephalon – occipital]/occipital), which is proportional to SERT number (i.e., Bmax), assuming that both the affinity (KD) and the nondisplaceable binding (V2) do not vary significantly within the population. Before scanning, four or five fiducial markers filled with 5 μCi of Na99mTcO4 were attached to the skin along the canthomeatal plane to identify it during image analysis.
The images were reconstructed from photopeak counts (mean=159 keV, SD=16) by using standard filtered backprojection methods (Butterworth, power=10, cutoff=1.16 cm–1) and displayed as a 128×128×64 matrix with a voxel size of 2.07×2.07×3.56 mm (15.25 mm3). Subsequent image analysis was performed by an operator who was unaware of subject demographics. SPECT data were reoriented to correct for deviations from the canthomeatal plane, as identified by the fiducial markers. Eight contiguous transaxial slices with the highest uptakes in the brainstem-diencephalon and the striatum, respectively, were identified from a reconstructed midsagittal image and digitally summed to yield two transaxial slices, each 28.5 mm thick. Attenuation correction was performed by using a Chang zero-order method (attenuation coefficient μ=0.15 cm–1) within an ellipse drawn around the skull. Standard region-of-interest templates (previously published [29]) for the brainstem-diencephalon (432 voxels or 6.6 ml) and the occipital cortex (7912 voxels or 120.6 ml) were positioned on their respective summed slices, with the occipital region of interest placed at the striatal level. No attempt was made to correct for scatter or partial-volume effects.
Statistical Analysis
Chi-square tests were used to compare observed genotype frequencies to those predicted by the Hardy-Weinberg equilibrium and also to compare sex distributions among genotypic groups. Subject age was compared across groups by using analysis of variance (ANOVA). V3′′ for the brainstem-diencephalon was computed without conversion of SPECT cpm to absolute units of radioactivity as [(cpm/voxel)brainstem-diencephalon – (cpm/voxel)occipital]/(cpm/voxel)occipital. Values of V3′′ for the brainstem-diencephalon were then compared across genotypic groups by using analysis of covariance (ANCOVA) with control for age, which has been strongly correlated with brainstem-diencephalon V3′′ in previous studies with [123I]β-CIT (29). For significant main effects by ANOVA or ANCOVA, Tukey post hoc pairwise comparisons were performed. All statistical analyses used the SPSS (SPSS, Inc., Chicago) or SYSTAT (SYSTAT, Inc., Evanston, Ill.) software packages and employed two-tailed tests of significance.
Results
Genotypic Composition of the Group
Frequencies of SLC6A4 5-HTTLPR genotypes for the 96 subjects are displayed in Table 1. Of these, 36 were classified as 5-HTTLPR long-long homozygotes, 18 as short-short homozygotes, and 42 as long-short heterozygotes. Overall frequencies of the long and short alleles, 59% and 41%, respectively, are similar to those observed by Lesch et al. (1) (57% and 43%, respectively) in a predominantly Caucasian population, as well as those observed in homogeneous healthy European American groups by Gelernter et al. (10) (60% and 40%, respectively). The distribution of the three common genotypes did not differ significantly from Hardy-Weinberg equilibrium expectations (Table 1).
Unexpectedly, the three genotypic groups differed significantly with respect to age (F=8.22, df=2, 93, p=0.0005, ANOVA), as the long-long homozygotes (mean=59.2 years, SD=19.3) were significantly older than both the short-short homozygotes (mean=40.7 years, SD=14.4) (p=0.002, Tukey pairwise comparison) and the long-short heterozygotes (mean=46.2 years, SD=17.7) (p=0.005). The genotypic groups did not differ significantly with regard to sex (male:female; for long-long, 17:19; for long-short, 27:15; for short-short, 10:8) (χ2=2.30, df=2, p=0.32).
Effect of Genotype on SERT Availability
The values of V3′′ for the brainstem-diencephalon are displayed in Figure 1. When the contribution of age was controlled, the main effect of genotype was significant (F=4.48, df=2, 92, p=0.01, ANCOVA). Post hoc Tukey pairwise comparisons revealed that the short-short homozygotes had significantly greater SERT availability than the long-short heterozygotes (p=0.02). In addition, there was a nonsignificant tendency for the long-long homozygotes to have greater SERT availability than the long-short heterozygotes (p=0.09), but there was no difference between the long-long homozygotes and the short-short homozygotes (p=0.70). The effect of age was significant in the ANCOVA model for the brainstem-diencephalon (t=–3.61, df=95, p<0.001). The interaction of age by genotype was also significant (F=5.19, df=2, 93, p=0.007), with the short-short homozygotes showing a greater decline in V3′′ with age than either of the other two groups, possibly because of the small number of elderly short-short homozygotes (only one subject >60 years). As in our previous report (29), the addition of gender (with or without the interaction of age and gender) did not significantly improve the prediction of V3′′ after control for the contribution of age.
Discussion
We examined the effect of the SLC6A4 5-HTTLPR genotype on SERT availability in the largest and most homogeneous subject group reported to date: a group of 96 healthy European Americans. When the contribution of age was controlled, the main effect of genotype was significant, with the short-short homozygotes demonstrating significantly greater SERT availability than the long-short heterozygotes, and the long-long homozygotes showing a nonsignificant tendency for greater SERT availability than the heterozygotes. No difference was observed between the long-long homozygotes and the short-short homozygotes.
The significant difference in age distributions of the three genotypic groups (long-long > long-short and short-short) is difficult to explain. The long allele could conceivably possess a survival or sampling advantage in older healthy subject populations, given the association of the short allele with several conditions (depression or suicide [4, 14], bipolar disorder [7, 8], and late-onset Alzheimer’s disease [15, 16]) that might eliminate older individuals from the healthy subject pool. However, any advantage of the long allele would likely be insufficient to account for these large age differences, and another report found no difference in genotypic frequencies between young and older healthy subject groups (16). A random sampling effect seems more likely. In any case, the age differences among genotypic subgroups dictate caution in interpreting the present results. Similarly, the significant interaction of genotype by age in the ANCOVA results (with short-short homozygotes showing a steeper age-related decline than the other two groups) must be taken with caution, given the skewed age distribution of the short-short subjects (only one >60 years).
Comparison With Other Neuroimaging Studies
These results differ from those of two previous neuroimaging investigations (also using [123I]β-CIT SPECT) of the SLC6A4 genotype and protein availability in smaller groups. Heinz et al. (25) found that among eight healthy comparison subjects, SERT availability was greater in long-long homozygotes than in short carriers, whereas among 14 abstinent alcoholic subjects, SERT availability was lower for long-long homozygotes than for short carriers. Jacobsen et al. (26) reported no effect of the SLC6A4 genotype on SERT availability in 27 healthy comparison subjects.
The divergent results among these three studies are potentially attributable to several differences in methods. Heinz et al. (25) employed the outcome measure BP′, a ratio of the specific tracer uptake to the free plasma [123I]β-CIT concentration at equilibrium, whereas Jacobsen et al. (26) and the present study both used V3′′, a ratio of specific to nondisplaceable binding at equilibrium. V3′′ has the theoretical disadvantage of assuming equivalent nondisplaceable tracer uptake between subjects (or at least study groups) (30, 31) but may be more reproducible since it does not depend on plasma measurements. Our results are unlikely to be confounded by a systematic difference in nondisplaceable [123I]β-CIT uptake among long-long homozygotes, short-short homozygotes, and long-short heterozygotes. Heinz et al. (25) and Jacobsen et al. (26) both used MRI coregistration for region-of-interest placement, permitting the use of the cerebellum as a reference region, whereas the present study used the occipital cortex as a reference region. Although a previous primate study (24) demonstrated no displaceable component of cortical [123I]β-CIT uptake, human postmortem studies have found low but measurable levels of SERT in the occipital cortex (32, 33), including by [123I]β-CIT binding studies (34). However, this difference is again unlikely to yield systematic differences among genotypic groups. Only Jacobsen et al. (26) performed measured attenuation correction, whereas the present study assumed uniform attenuation within an ellipse drawn around the skull, and Heinz et al. (25) performed no attenuation correction (personal communication). However, the increased accuracy in [123I]β-CIT quantitation by measured—compared to uniform—attenuation correction appears to be small (35) and, in any case, would not introduce systematic biases among genotypic groups.
Other important differences among these three studies include varying the diagnostic and racial composition of the subject groups. Whereas the present study and that of Jacobsen et al. (26) included only healthy subjects, the subjects of Heinz et al. (25) comprised 14 abstinent alcoholics and eight comparison subjects. SLC6A4 5-HTTLPR allele frequency is known to vary across population groups (10, 36), although no effect on SERT availability has been determined for race or the interaction of race and genotype. Since we lacked sufficient racial subsamples to undertake a systematic investigation of racial effects, we opted to restrict our analysis to our largest population subgroup of European Americans. The other two studies (25, 26) both included small numbers of subjects of non-European ancestry, although neither study possessed the statistical power to address the effect of race on SERT availability rigorously. Future studies using larger groups of different racial groups will be necessary to address this question.
All three studies are limited by [123I]β-CIT’s dual affinity for SERT and the dopamine transporter. Although displacement binding studies in primates have demonstrated that [123I]β-CIT uptake in the brainstem-diencephalon is primarily associated with SERT (24) (i.e., displaceable by citalopram but not GBR-12909), a small component of this uptake is likely attributable to the dopamine transporter. Ex vivo autoradiography studies of three monkeys from our group (unpublished data) suggest that [123I]β-CIT uptake in the brainstem-diencephalon is distributed (starting from the region with the highest uptake) in 1) the superior colliculus, 2) the dorsal and median raphe nuclei, 3) the substantia nigra, and 4) several hypothalamic and thalamic nuclei. Although this distribution closely mirrors that of SERT binding in homogenate binding and autoradiographic studies in human and nonhuman primates (32, 33, 37), the substantia nigra also contains significant levels of dopamine transporter. This relatively small dopamine transporter component of brainstem uptake may account for the less than complete displacement of [123I]β-CIT binding in the human brainstem-diencephalon by citalopram (38). Therefore, replication of the present findings using a more selective positron emission tomography or SPECT tracer would be valuable.
Comparison With Previous Studies
The present results are somewhat paradoxical in suggesting the lowest SERT availability for the long-short heterozygotes, with relatively higher values for either homozygote condition (in the case of the long-long homozygotes, by a nonsignificant tendency). However, they are remarkably similar to the postmortem results of Little et al. (20) in 17 human cocaine users and 21 healthy comparison subjects, in which lower SERT binding (by [125I]β-CIT in the dorsal and median raphe and the substantia nigra) was observed in long-short heterozygotes than in long-long homozygotes or short-short homozygotes. They also bear similarity to the postmortem findings of Du et al. (4) in eight suicide victims and 15 healthy comparison subjects. They reported greater SERT binding (by [3H]paroxetine in the prefrontal cortex) in short-short homozygotes than in long carriers. Comparison of the present results with those obtained in mixed healthy comparison-patient groups is clearly limited by the possibility that disease states may alter the relationship between the SLC6A4 genotype and SERT binding.
Thus, the emerging brain binding and imaging studies of the SERT genotype-phenotype relationship are somewhat at odds with the previous in vitro data from nonneural cells. In their original landmark report, Lesch et al. (1) used cultured human lymphoblastoid cell lines and observed reductions in SERT mRNA concentrations, SERT expression, and 5-HT reuptake in lymphoblasts carrying the short allele compared to long-long homozygotes. Heils et al. (19) also performed functional studies of the native 5-HTTLPR in a human placental choriocarcinoma cell line (JAR) and found that transcriptional activity (both basal and induced by cAMP or protein kinase C) was increased by the long allele compared to the short allele. Greenberg et al. (39) investigated the expression and function of 5-HTTLPR in human platelets and found no difference in SERT expression but more rapid initial 5-HT uptake in long-long homozygotes than in short carriers. In the postmortem brain study of Little et al. (20), the authors also measured SERT mRNA levels and observed the same pattern as Lesch et al. (1), with lower SERT mRNA levels in the short carriers than in the long-long homozygotes. Overall, the available postmortem/imaging and in vitro studies are in substantial agreement with regard to the comparison between long-long homozygotes and long-short heterozygotes but diverge strikingly with regard to the short-short homozygotic condition.
These differences between in vitro results in nonneural cells and brain binding and imaging studies are not readily explained. However, the 5-HTTLPR polymorphism may play a more complex role in neural tissue than in lymphoblasts or placental choriocarcinoma cells, and SERT binding sites also may differ in these tissues. Alternatively, SERT protein levels in the brain may not strictly reflect transcriptional activity, and putative associations between the 5-HTTLPR polymorphism and clinical phenotypes and diseases may result from a mechanism other than adult basal levels (e.g., SERT levels during development, under conditions of stress). The differences could also reflect interactions with other loci, i.e., epistatic effects. Finally, associations between this polymorphism and protein levels (as well as disease) may simply be population dependent.
In conclusion, these results do not suggest higher central SERT levels in association with the long allele in European American subjects but point to a more complex relationship between SLC6A4 genotype and protein availability. SLC6A4 5-HTTLPR short-short homozygotes (and possibly long-long homozygotes) may have greater SERT availability than heterozygotes. These results may enhance the understanding of previously observed associations between the SLC6A4 genotype and neuropsychiatric diseases and phenotypes.
![]() |
Received Sept. 17, 2002; revisions received Feb. 21 and July 15, 2003; accepted July 21, 2003. From the Departments of Psychiatry, Neurobiology, and Diagnostic Radiology, Yale University School of Medicine; and West Haven VA Medical Center, West Haven, Conn. Address reprint requests to Dr. van Dyck, Alzheimer’s Disease Research Unit, Department of Psychiatry, Yale University School of Medicine, One Church St., Suite 600, New Haven, CT 06510; christopher. [email protected] (e-mail). Supported by the American Federation for Aging Research (grant to Dr. van Dyck), a National Alliance for Research on Schizophrenia and Depression Independent Investigator Award (to Dr. Malison), gifts from Rose and Philip Hoffer, funds from the Department of Veterans Affairs (the Research Enhancement Award in Depression), and grants from NIMH (MH-48243, MH-58620, and MH-01387) and from the National Institute on Drug Abuse (DA-12849 and DA-12690). The authors thank Heide Klumpp, Michelle Early, Eileen Smith, Gary Wisniewski, Louis Amici, and Ann Marie Lacobelle for technical assistance and Larry Muenz, Ph.D., for statistical advice.
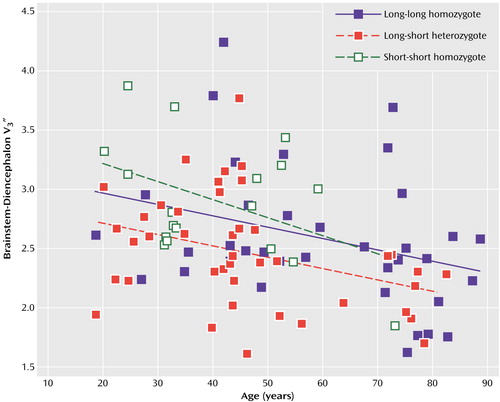
Figure 1. Relation of Serotonin Transporter Protein (SERT) Availability (V3′′) in the Brainstem-Diencephalon to Age in 96 Healthy European American Subjects Grouped by Genotype for the 5-HTTLPR Polymorphism of the SERT Gene (SLC6A4)a
aAvailability of SERT was measured with [123I]2β-carbomethoxy-3β-(4-iodophenyl)tropane and single photon emission computed tomography. The main effect of genotype was significant with control for age (F=4.48, df=2, 92, p=0.01, ANCOVA). Post hoc Tukey pairwise comparisons revealed that the short-short homozygotes (N=18) had significantly greater SERT availability than the long-short heterozygotes (N=42) (p=0.02). In addition, there was a nonsignificant tendency for the long-long homozygotes (N=36) to have greater SERT availability than the long-short heterozygotes (p=0.09), but there was no difference between the long-long homozygotes and the short-short homozygotes (p=0.70).
1. Lesch KP, Bengel D, Heils A, Sabol SZ, Greenberg B, Petri S, Benjamin J, Muller CR, Hamer DH, Murphy DL: Association of anxiety-related traits with a polymorphism in the serotonin transporter gene regulatory region. Science 1996; 274:1527–1531Crossref, Medline, Google Scholar
2. Lesch KP, Mössner R: Genetically driven variation in serotonin uptake: is there a link to affective spectrum, neurodevelopmental, and neurodegenerative disorders? Biol Psychiatry 1998; 44:179–192Crossref, Medline, Google Scholar
3. Gelernter J, Kranzler H, Coccaro EF, Siever LJ, New AS: Serotonin transporter protein gene polymorphism and personality measures in African American and European subjects. Am J Psychiatry 1998; 155:1332–1338Link, Google Scholar
4. Du L, Faludi G, Palkovits M, Demeter E, Bakish D, Lapierre YD, Sotonyi P, Hrdina PD: Frequency of long allele in serotonin transporter gene is increased in depressed suicide victims. Biol Psychiatry 1999; 46:196–201Crossref, Medline, Google Scholar
5. Whyte EM, Pollock BG, Wagner WR, Mulsant BH, Ferrell RE, Mazumdar S, Reynolds CF III: Influence of serotonin-transporter-linked promoter region polymorphism on platelet activation in geriatric depression. Am J Psychiatry 2001; 158:2074–2076Link, Google Scholar
6. McDougle CJ, Epperson CN, Price LH, Gelernter J: Evidence for linkage disequilibrium between serotonin transporter protein gene (SLC6A4) and obsessive compulsive disorder. Mol Psychiatry 1998; 3:270–274Crossref, Medline, Google Scholar
7. Collier DA, Stober G, Li T, Heils A, Catalano M, Di Bella D, Arranz MJ, Murray RM, Vallada HP, Bengel D, Muller CR, Roberts GW, Smeraldi E, Kirov G, Sham P, Lesch KP: A novel functional polymorphism within the promoter of the serotonin transporter gene: possible role in susceptibility to affective disorders. Mol Psychiatry 1996; 1:453–460Medline, Google Scholar
8. Bellivier F, Henry C, Szoke A, Schurhoff F, Nosten-Bertand M, Feingold J, Launay JM, Leboyer M, Laplanche JL: Serotonin transporter gene polymorphisms in patients with unipolar or bipolar depression. Neurosci Lett 1998; 255:143–146Crossref, Medline, Google Scholar
9. Mundo E, Walker M, Cate T, Macciardi F, Kennedy JL: The role of serotonin transporter protein gene in antidepressant-induced mania in bipolar disorder. Arch Gen Psychiatry 2001; 58:539–544Crossref, Medline, Google Scholar
10. Gelernter J, Kranzler H, Cubells JF: Serotonin transporter protein (SLC6A4) allele and haplotype frequencies and linkage disequilibria in African- and European-American and Japanese populations and in alcohol-dependent subjects. Hum Genet 1997; 101:243–246Crossref, Medline, Google Scholar
11. Edenberg HJ, Reynolds J, Koller DL, Begleiter H, Bucholz KK, Conneally PM, Crowe R, Goate A, Hesselbrock V, Li TK, Nurnberger JIJ, Porjesz B, Reich T, Rice JP, Schuckit M, Tischfield JA, Foroud T: A family-based analysis of whether the functional promoter alleles of the serotonin transporter gene HTT affect the risk for alcohol dependence. Alcohol Clin Exp Res 1998; 22:1080–1085Crossref, Medline, Google Scholar
12. Schuckit MA, Mazzanti C, Smith TL, Ahmed U, Radel M, Iwata N, Goldman D: Selective genotyping for the role of 5-HT2A, 5-HT2C, and GABA alpha 6 receptors and the serotonin transporter in the level of response to alcohol: a pilot study. Biol Psychiatry 1999; 45:647–651Crossref, Medline, Google Scholar
13. Lichtermann D, Hraniloviæ D, Trixler M, Franke P, Jernej B, Delmo CD, Knapp M, Schwab SG, Maier W, Wildenauer DB: Support for allelic association of a polymorphic site in the promoter region of the serotonin transporter gene with risk for alcohol dependence. Am J Psychiatry 2000; 157:2045–2047Link, Google Scholar
14. Gorwood P, Batel P, Ades J, Hamon M, Boni C: Serotonin transporter gene polymorphisms, alcoholism, and suicidal behavior. Biol Psychiatry 2000; 48:259–264Crossref, Medline, Google Scholar
15. Li T, Holmes C, Sham PC, Vallada H, Birkett J, Kirov G, Lesch KP, Powell J, Lovestone S, Collier D: Allelic functional variation of serotonin transporter expression is a susceptibility factor for late onset Alzheimer’s disease. Neuroreport 1997; 8:683–686Crossref, Medline, Google Scholar
16. Oliveira JRM, Gallindo RM, Maia LGS, Brito-Marques PR, Otto PA, Passos-Bueno MR, Morais MA Jr, Zatz M: The short variant of the polymorphism within the promoter region of the serotonin transporter gene is a risk factor for late onset Alzheimer’s disease. Mol Psychiatry 1998; 3:438–441Crossref, Medline, Google Scholar
17. Moreno FA, Rowe DC, Kaiser B, Chase D, Michaels T, Gelernter J, Delgado PL: Association between a serotonin transporter promoter region polymorphism and mood response during tryptophan depletion. Mol Psychiatry 2002; 7:213–216Crossref, Medline, Google Scholar
18. Hariri AR, Mattay VS, Tessitore A, Kolachana B, Fera F, Goldman D, Egan MF, Weinberger DR: Serotonin transporter genetic variation and the response of the human amygdala. Science 2002; 297:400–403Crossref, Medline, Google Scholar
19. Heils A, Teufel A, Petri S, Stober G, Riederer P, Bengel D, Lesch KP: Allelic variation of human serotonin transporter gene expression. J Neurochem 1996; 66:2621–2624Crossref, Medline, Google Scholar
20. Little KY, McLaughlin DP, Zhang L, Livermore CS, Dalack GW, McFinton PR, DelProposto ZS, Hill E, Cassin BJ, Watson SJ, Cook EH: Cocaine, ethanol, and genotype effects on human midbrain serotonin transporter binding sites and mRNA levels. Am J Psychiatry 1998; 155:207–213Link, Google Scholar
21. Mann JJ, Huang Y, Underwood MD, Kassir SA, Oppenheim S, Kelly TM, Dwork AJ, Arango V: A serotonin transporter gene promoter polymorphism (5-HTTLPR) and prefrontal cortical binding in major depression and suicide. Arch Gen Psychiatry 2000; 57:729–738Crossref, Medline, Google Scholar
22. van Dyck CH, Gelernter J, MacAvoy MG, Avery RA, Criden M, Okereke O, Varma P, Seibyl JP, Hoffer B: The absence of an apolipoprotein E e4 allele is associated with increased parietal rCBF asymmetry in Alzheimer’s disease. Arch Neurol 1998; 55:1460–1466Crossref, Medline, Google Scholar
23. Boja JW, Mitchell WM, Patel A, Kopajtic TA, Carroll FI, Lewin AH, Abraham P, Kuhar MJ: High-affinity binding of [125I]RTI-55 to dopamine and serotonin transporters in rat brain. Synapse 1992; 12:27–36Crossref, Medline, Google Scholar
24. Laruelle M, Baldwin RM, Malison RT, Zea-Ponce Y, Zoghbi SS, Al-Tikriti MS, Sybirska EH, Zimmermann R, Wisniewski G, Neumeyer JL, Milius RA, Wang S, Smith EO, Roth RH, Charney DS, Hoffer PB, Innis RB: SPECT imaging of dopamine and serotonin transporters with [123I]β-CIT: pharmacological characterization of brain uptake in nonhuman primates. Synapse 1993; 13:295–309Crossref, Medline, Google Scholar
25. Heinz A, Jones D, Mazzanti C, Goldman D, Ragan P, Hommer D, Linnoila M, Weinberger DR: A relationship between serotonin transporter genotype and in vivo protein expression and alcohol neurotoxicity. Biol Psychiatry 2000; 47:643–649Crossref, Medline, Google Scholar
26. Jacobsen LK, Staley JK, Zoghbi SS, Seibyl JP, Kosten TR, Innis RB, Gelernter J: Prediction of dopamine transporter binding availability by genotype: a preliminary report. Am J Psychiatry 2000; 157:1700–1703Link, Google Scholar
27. Folstein MF, Folstein SE, McHugh PR: “Mini-Mental State”: a practical method for grading the cognitive state of patients for the clinician. J Psychiatr Res 1975; 12:189–198Crossref, Medline, Google Scholar
28. van Dyck CH, Seibyl JP, Malison RT, Laruelle M, Zoghbi SS, Baldwin RM, Innis RB: Age-related decline in dopamine transporters: analysis of striatal subregions, nonlinear effects, and hemispheric asymmetries. Am J Geriatr Psychiatry 2002; 10:36–43Crossref, Medline, Google Scholar
29. van Dyck CH, Malison RT, Seibyl JP, Laruelle M, Klumpp H, Zoghbi SS, Baldwin RM, Innis RB: Age-related decline in central serotonin transporter availability with [123I]β-CIT SPECT. Neurobiol Aging 2000; 21:497–501Crossref, Medline, Google Scholar
30. Laruelle M, Wallace E, Seibyl JP, Baldwin RM, Zea-Ponce Y, Zoghbi SS, Neumeyer JL, Charney DS, Hoffer PB, Innis RB: Graphical, kinetic, and equilibrium analyses of in vivo [123I]β-CIT binding to dopamine transporters in healthy human subjects. J Cereb Blood Flow Metab 1994; 14:982–994Crossref, Medline, Google Scholar
31. van Dyck CH, Seibyl JP, Malison RT, Wallace E, Zoghbi SS, Zea-Ponce Y, Baldwin RM, Charney DS, Hoffer PB, Innis RB: Age-related decline in dopamine transporter binding in human striatum with [123I]β-CIT SPECT. J Nucl Med 1995; 36:1175–1181Medline, Google Scholar
32. Cortes R, Soranoi E, Pazos A, Probst A, Palacios J: Autoradiography of antidepressant binding sites in the human brain: localization using [3H]imipramine and [3H]paroxetine. Neuroscience 1988; 27:473–496Crossref, Medline, Google Scholar
33. Laruelle M, Vanisberg M-A, Maloteaux J-M: Regional and subcellular localization in human brain of [3H]paroxetine binding, a marker of serotonin uptake sites. Biol Psychiatry 1988; 24:299–309Crossref, Medline, Google Scholar
34. Staley JK, Basile M, Flynn DD, Mash DC: Visualizing dopamine and serotonin transporters in the human brain with the potent cocaine analogue [125I]RTI-55: in vitro binding and autoradiographic characterization. J Neurochem 1994; 62:549–555Crossref, Medline, Google Scholar
35. Rajeevan N, Zubal IG, Ramsby SQ, Zoghbi SS, Seibyl J, Innis RB: Significance of nonuniform attenuation correction in quantitative brain SPECT imaging. J Nucl Med 1998; 39:1719–1726Medline, Google Scholar
36. Kunugi H, Hattori M, Kato T, Tatsumi M, Sakai T, Sasaki T, Hirose T, Nanko S: Serotonin transporter gene polymorphisms: ethnic difference and possible association with bipolar affective disorder. Mol Psychiatry 1997; 2:457–462Crossref, Medline, Google Scholar
37. Bäckström I, Bergström M, Marcusson J: High affinity [3H]paroxetine binding to serotonin uptake sites in human brain tissue. Brain Res 1989; 486:261–268Crossref, Medline, Google Scholar
38. Pirker W, Asenbaum S, Kasper S, Walter H, Angelberger P, Koch G, Pozzera A, Deecke L, Podreka I, Brücke T: β-CIT SPECT demonstrates blockade of 5-HT-uptake sites by citalopram in the human brain in vivo. J Neural Transm Gen Sect 1995; 100:247–256Crossref, Medline, Google Scholar
39. Greenberg BD, Tolliver TJ, Huang SJ, Li Q, Bengel D, Murphy DL: Genetic variation in the serotonin transporter promoter region affects serotonin uptake in human blood platelets. Am J Med Genet 1999; 88:83–87Crossref, Medline, Google Scholar