The Relationship of Clozapine and Haloperidol Treatment Response to Prefrontal, Hippocampal, and Caudate Brain Volumes
Abstract
OBJECTIVE: The study was designed to assess the predictive relationship between brain structure volume and positive and negative symptom response to clozapine and haloperidol. METHOD: Partially responsive outpatients with schizophrenia who participated in a 10-week, parallel-group, double-blind comparison of clozapine and haloperidol and who had an available magnetic resonance imaging scan were included in the current study. Prefrontal gray and white matter, hippocampal, and caudate volumes were manually measured. The Scale for the Assessment of Negative Symptoms (SANS) and the Brief Psychiatric Rating Scale (BPRS) were used to assess symptom changes. The Simpson-Angus Rating Scale was used to assess extrapyramidal symptoms. RESULTS: Twenty-two patients randomly assigned to clozapine and 23 patients assigned to haloperidol met study entry criteria. There were significant interactions between treatment and right prefrontal gray matter volume for BPRS total score and SANS total score. There were no significant treatment-by-brain structure interactions for BPRS positive symptom items. Right prefrontal gray matter volume was also related to differential treatment effects for the BPRS subscales of anxiety/depression and hostility and the Simpson-Angus Rating Scale akathisia item. CONCLUSIONS: These results suggest that there is a differential interaction among clozapine and haloperidol, brain structure, and treatment response. Partially responsive patients with larger brain volumes may be more likely to experience the benefits of clozapine treatment, but they may be more vulnerable to side effects and experience a subsequent worsening of their symptoms when treated with haloperidol.
Thirty to 60% of patients refractory to conventional antipsychotics respond to clozapine (1). The pharmacological mechanism(s) through which clozapine exerts its superior efficacy are unknown. Speculation has focused on the unique binding properties of clozapine, including its ability to antagonize the D4 dopamine receptor, its greater affinity for the 5-HT2A serotonin receptor versus the D2 dopamine receptor, and its greater affinity for the D1 versus the D2 dopamine receptor (1). Alternatively, the neural substrate of the pharmacological actions of clozapine may differ in important respects from those of conventional antipsychotics (2).
Structural and functional imaging techniques have been used to examine the relationship between neuroanatomy and clozapine response. In a computed tomography (CT) study of 34 treatment-resistant patients, clozapine response, as defined by percent change in Brief Psychiatric Rating Scale (BPRS) total score, was related to a smaller prefrontal sulcal prominence (3). In a second CT study of 42 treatment-resistant patients, those who responded to clozapine according to Clinical Global Impression ratings had smaller frontal and temporal sulci (4). A magnetic resonance imaging (MRI) study with 18 patients did not observe any significant relationships between morphological variables and clozapine response (5). In contrast, Lauriello et al. (6) found that clozapine response was significantly related to larger anterior superior temporal lobe CSF volume. In this MRI study of 21 patients, there were no significant relationships between clozapine response and prefrontal or frontal measures. In SPECT functional neuroimaging studies, increased perfusion in the thalamus and right prefrontal cortex has been associated with clozapine response (7, 8).
In the present study, we used structural MRI to examine whether morphological brain measures could predict clozapine response in a group of partially responsive outpatients with schizophrenia. We selected brain regions that have been previously implicated in the underlying pathophysiology of positive symptoms (i.e., the hippocampus) and negative symptoms (i.e., the prefrontal cortex and caudate nucleus) (9). On the basis of previous findings, we hypothesized that hippocampal volume would be related to positive symptom change and that prefrontal cortex and caudate volumes would be related to negative symptom change (9, 10).
Method
Patient Group
Study details have been published elsewhere (11, 12). In brief, 75 patients from an outpatient schizophrenia research clinic entered a 10-week, parallel-group, double-blind comparison of clozapine and haloperidol. Diagnosis of schizophrenia was made with the Structured Clinical Interview for DSM-III-R, direct assessment, family informants, and past medical records. Patients with a neurological disorder, an IQ below 70, or a current DSM-III-R drug abuse or dependence diagnosis were excluded from the study. Patients were required to meet retrospective and prospective criteria for partial response to conventional antipsychotics (12). Retrospective criteria included a history of residual positive or negative symptoms after at least two 6-week therapeutic trials of conventional antipsychotics and a minimum level of positive and negative symptoms at the time of study entry. The prospective evaluation of partial responsiveness consisted of a 6-week trial of open-label fluphenazine, 20 mg/day, with dose adjustments between 10 and 30 mg/day. Subjects with a 30% or greater improvement in positive or negative symptoms were excluded from the double-blind trial. Over the first 4 weeks of the double-blind trial, doses of clozapine and haloperidol were gradually increased to 400 mg/day and 20 mg/day, respectively. Clozapine and haloperidol doses could be adjusted over the next 2 weeks within fixed limits (200–600 mg/day for clozapine and 10–30 mg/day for haloperidol) in order to maximize efficacy or minimize side effects. Doses were then fixed for the remainder of the study. Patients randomly assigned to haloperidol were also prescribed double-blind benztropine (4 mg/day), and patients assigned to clozapine received placebo benztropine. All patients provided written informed consent after the study procedures had been fully explained and prior to study participation.
Clinical Measures
The BPRS (13) total score was used to assess global symptom change. The four BPRS positive symptom items (conceptual disorganization, hallucinations, unusual thought content, and suspiciousness) were used to assess positive symptom change. The Scale for the Assessment of Negative Symptoms (SANS) (14) total score was used to assess negative symptom change. The SANS total score included all items except for the inappropriate affect, poverty of content of speech, attentional, and global items. The first three items were excluded because analytic factor studies have suggested that those items are not closely related to negative symptoms (15). The global items were excluded because they are redundant with the individual items. Symptom assessments were collected at baseline and at the end of the study. The baseline symptom measure was the average of the last two ratings in the 6-week open-label fluphenazine trial. The symptom assessments were conducted by master’s- and doctoral-level clinicians. Intraclass correlation coefficients for the BPRS and SANS measures ranged from 0.76 to 0.90. The Simpson-Angus Rating Scale was used to assess extrapyramidal symptoms, and the Simpson-Angus Rating Scale akathisia item was used to assess akathisia (16).
Imaging
The MRI procedures have been previously described (17, 18). In brief, the MRI scans were performed on a Siemens 2-T Magnetom system operating at 1.5 T. The high-resolution spin-echo technique was used to evaluate the whole brain in the coronal plane in 3-mm-thick contiguous slices, with a repetition time of 600 msec, an echo time of 17 msec, and a matrix size of 256×256 pixels, with two excitations. Morphometric analyses were performed with the Loats image analysis system (19). The sample function of the image analysis system was used to determine the hippocampal and caudate brain volumes. This function enables the investigator to outline the region of interest on the MRI image and calculates the area of the demarcated region. Volumes are calculated by summing the area measurements across all appropriate images and multiplying by the slice thickness. The threshold function of the image analysis system was used to determine prefrontal gray and white matter volumes. This function enables the investigator to partition gray matter from both CSF and white matter by assigning nonoverlapping signal intensity ranges to each area (20). CSF and gray and white matter were partitioned on each MRI slice containing the prefrontal region, and the sample function was used to outline the prefrontal hemispheres generating separate gray and white matter area measurements. Volumes were calculated by summing the area measurements and multiplying by the slice thickness.
Anatomical Boundaries
The following brain regions were assessed: the prefrontal cortex, caudate, and the hippocampus. The anterior boundary of the prefrontal cortex was the first anterior coronal slice containing gray matter. The posterior boundary was the first slice demonstrating the genu of the corpus callosum. The anterior boundary of the caudate was the first slice that contained the caudate and the genu of the corpus callosum and the anterior horns of the lateral ventricles. The posterior boundary where the caudate turns laterally and caudally was defined by the presence of the trigone of the lateral ventricle and the splenium of the corpus callosum. The medial boundary was defined by the lateral ventricle, and the lateral boundary was primarily defined by the internal capsule. The tail of the caudate was not measured. The anterior boundary of the hippocampus was the first slice that contained the hippocampus and did not contain the amygdala. The amygdala and hippocampus co-occur in one to two coronal slices. These two structures could not be reliably distinguished on these slices and were not used in the determination of hippocampal volume. Therefore, the hippocampal volume is an approximation of the “true” volume. The posterior boundary was defined as the most posterior slice containing the hippocampus. This slice was typically at the level of the splenium of the corpus callosum and the trigone of the lateral ventricle. The lateral boundary was either the temporal horn of the lateral ventricle or the temporal lobe white matter adjacent to the lateral margin of the hippocampus. The medial boundary was defined by the crus cerebri, the adjacent cistern, and included the presubiculum, subiculum, and uncus but not the entorhinal or parahippocampus gyri. The intraclass correlation coefficients for all brain regions ranged from 0.90 to 0.99 (17). Each structure was analyzed by the same rater to reduce measurement variance. All measurements were performed blind to any identifying information.
Statistical Analysis
Statistical analyses were carried out in a hierarchical manner to control the type I error rate. To identify the distinctive brain regions through which clozapine and haloperidol exerted their effects on symptoms, we used multivariate analysis of variance (MANOVA) to fit the following model:
Symptom scores=treatment+gender+brain structure volume+ interaction between treatment and brain structure volume.
BPRS total score, BPRS positive symptom item score, and SANS total score were the dependent variables. A statistically significant (p<0.05) test of the interaction between brain structure volume and treatment was interpreted as evidence of a distinctive mechanism of action for one treatment not shared with the other. If an initial MANOVA interaction test was significant for a given brain structure, then post hoc analyses, at alpha=0.05, were conducted to determine which symptom rating score was involved in the interaction. If the BPRS total score or SANS total score brain region interaction was statistically significant, then exploratory analyses were conducted to test for structure-by-symptom subscale interactions to see whether the effect was concentrated in individual symptom clusters (i.e., BPRS anxiety/depression, hostility, activation, or positive symptom subscales; SANS affect, alogia, anhedonia, or avolition subscales). Since this procedure did not guarantee an overall alpha <0.05 for these analyses, they were regarded as exploratory hypothesis-generating analyses and were identified as such. The same MANOVA model was used to examine treatment-by-brain structure interactions for extrapyramidal symptoms. Simpson-Angus Rating Scale total score and the Simpson-Angus Rating Scale akathisia item were the dependent variables. Spearman rank correlations were used to examine within-group relationships between brain region volume and symptom change.
Results
Seventy-five patients entered the double-blind study, and 64 patients completed the study (34 patients randomly assigned to haloperidol and 30 patients assigned to clozapine) (12). Twenty-three of the 34 haloperidol-treated patients and 22 of the 30 clozapine-treated patients had available MRI scans. There were no significant differences between the patients who received a scan and those who did not on any of the baseline clinical measures.
Clinical, sociodemographic, and end-of-study drug dose data are presented in Table 1. There were no treatment group differences for any of the clinical or sociodemographic variables.
The MRI variables for the two treatment groups are presented in Table 2. The brain region measurements obtained from a group of healthy comparison subjects, who underwent the same MRI protocol, are presented in the table for the purposes of comparison. The brain regions for which there were significant volumetric differences between the two groups in the original MRI study by Breier et al. (17) are denoted. There were no significant brain volume differences between the clozapine and haloperidol treatment groups. The prefrontal measures for one patient randomly assigned to haloperidol and the caudate measures for one patient assigned to clozapine were not available because of movement artifact.
Baseline and end-of-study symptom measures are included in Table 3. In this subgroup of patients from the original double-blind study for whom we had MRI data, clozapine was significantly superior to haloperidol for the BPRS positive symptom item, SANS total score, and the SANS anhedonia subscale. As shown in Table 3, patients receiving haloperidol worsened in all negative symptom measures.
Initial MANOVA analyses were carried out to test for structure-by-treatment interactions, with the BPRS total score, BPRS positive symptom item score, and SANS total score as dependent measures (Table 4). Only the interaction between right prefrontal cortex gray matter and treatment was statistically significant. Post hoc tests found significant interactions between right prefrontal cortex gray matter and treatment for the BPRS total score (F=9.89, df=1, 39, p=0.003) (Figure 1) and SANS total score (F=7.29, df=1, 39, p=0.01) (Figure 2), but not for the BPRS positive symptom item score (F=0.73, df=1, 39, p=0.40).
Spearman rank correlations were conducted to examine within-treatment group relationships between right prefrontal cortex gray matter volume and BPRS and SANS total scores. The right prefrontal cortex gray matter volume and BPRS total score were significantly correlated in the haloperidol group (rs=0.59, p=0.005) but not in the clozapine group (rs=–0.29, p=0.21). The Spearman rank correlation of right prefrontal cortex gray matter volume and SANS total score was statistically significant in the clozapine group (rs=–0.48, p=0.03) but not in the haloperidol group (rs=0.37, p=0.10).
Further exploratory post hoc analyses were carried out to see if the interactions between right prefrontal cortex gray matter and BPRS and SANS total scores reflected effects on specific subscales (Table 5). There was evidence for significant interactions between right prefrontal cortex gray matter volume and treatment for the BPRS subscales of hostility and anxiety/depression, with tendencies for larger right prefrontal cortex volumes to be correlated with more improvement in the clozapine group but with higher symptom levels in the haloperidol group. The Spearman rank correlation of BPRS hostility subscale score with right prefrontal cortex gray matter volume was statistically significant in the haloperidol group but not in the clozapine group (Table 5). There was marginally nonsignificant interaction evidence for the SANS affect subscale. There were no significant interactions between right prefrontal cortex gray matter volume and treatment for the other three SANS subscales, although all showed a similar pattern of modest positive correlations between symptoms and right prefrontal cortex gray matter volume for the haloperidol group and negative correlations of symptoms with right prefrontal cortex gray matter volume for the clozapine group.
In order to examine the specificity of the interactions between right prefrontal cortex gray matter volume and symptom measures, we examined separately the interactions between left prefrontal cortex gray matter and treatment for BPRS total score, BPRS positive symptom items, and SANS total score. There were no significant interactions (BPRS total score: F=1.79, df=1, 39, p=0.19; BPRS positive symptom items: F=0.26, df=1, 39, p=0.61; SANS total score: F=1.82, df=1, 39, p=0.18).
The MANOVA analyses to test for treatment-by-structure interactions for Simpson-Angus Rating Scale total and akathisia item scores as dependent measures were nonsignificant. The MANOVA for the interaction between right prefrontal cortex gray matter volume and treatment was marginally nonsignificant (F=3.11, df=2, 37, p=0.057). Post hoc tests found a significant interaction between right prefrontal cortex gray matter and treatment for the Simpson-Angus Rating Scale akathisia item (F=6.15, df=1, 38, p=0.02) but not for the Simpson-Angus Rating Scale total score (F=0.78, df=1, 38, p=0.38). However, the Simpson-Angus Rating Scale akathisia item score had a limited range (0 to 3) and was not normally distributed. Therefore, to confirm these results, we compared the Spearman correlations in the haloperidol group (rs=0.58, p=0.007) and the clozapine group (rs=–0.23, p=0.33) by using the Fisher’s z-transformation test, obtaining marginal support for a difference in correlations between the two groups (z=1.904, p=0.057).
Discussion
The major finding of the study was the observation of significant interaction between treatment and right prefrontal gray matter volume for SANS total score and BPRS total score. In general, in clozapine-treated patients, larger right prefrontal gray matter volume was associated with better treatment response, whereas in haloperidol-treated patients, larger right prefrontal gray matter volume was associated with poorer treatment response. The observation of a significant relationship between right prefrontal cortex volume and SANS total score supports our a priori hypothesis for this region and symptoms. In the clozapine-treated patients, the correlation between change in SANS total score and right prefrontal gray matter volume was significant, i.e., patients with larger brain volumes exhibited a better negative symptom response. There were no significant relationships with any of the SANS subscales. The study failed to support our a priori hypothesis of a predictive relationship between caudate volume and negative symptom response. In contrast to our study hypothesis, there was no relationship between hippocampal volume and positive symptom response. In the haloperidol-treated patients, there was a significant inverse correlation between change in BPRS total score and right prefrontal gray matter volume, i.e., patients with larger brain volumes exhibited a poorer global response. There was also a significant inverse correlation between change in BPRS hostility subscale score and right prefrontal gray matter volume. We had not specified a priori hypotheses for either of these measures.
An important aspect of the study is that the examination of the predictive relationship between morphological brain measures and clozapine treatment response occurred in the context of a double-blind clinical trial. The double-blind study design limits selection bias and, more important, the presence of a comparison treatment arm provides a framework for the interpretation of the observed brain structure and clozapine treatment response relationships.
The relationship between right prefrontal gray matter volume and clozapine response is consistent with some but not all previous studies. In the CT structural imaging studies, sulcal measurements were used to examine the integrity of the prefrontal cortex (3, 4). Presumably, there is an inverse relationship between sulcal and cortical gray matter measures (i.e., patients with smaller prefrontal sulci have larger prefrontal gray matter volumes). In the Friedman et al. study (3), there was an inverse relationship between prefrontal sulcal prominence and negative symptom response, as measured by the BPRS, which would be consistent with the current study results. There are also two negative MRI structural imaging studies (5, 6). In the first study, only global morphological measures are reported, with no specific frontal lobe measures (5). In the second study, prefrontal volumes were specifically measured, but only prefrontal total volumes were reported (6). There were not any separate analyses for prefrontal gray and white matter.
In functional imaging studies, clozapine responders showed higher perfusion in the right prefrontal cortex, thalamus, and left basal ganglia (7, 8). The higher perfusion could be related to larger volumes of these structures. However, neither of these studies examined whether the magnitude of perfusion was related specifically to either positive or negative symptom response. Finally, the plausibility of the observed relationship between right prefrontal gray matter volume and negative symptom response receives indirect support from the Wolkin et al. study (21), which observed that negative symptom severity was inversely related to glucose metabolism in the right prefrontal cortex.
Clozapine has been repeatedly observed to reduce caudate volumes in patients previously treated with conventional antipsychotics (22). In a recent study, positive and general symptom response to clozapine was related to reduction in the left caudate volume after clozapine initiation (23). In the present study, the MRI was obtained before the onset of clozapine treatment. Therefore, the only conclusion that we can make is that baseline caudate volume does not seem to predict clozapine response.
In the current study, there were significant group differences for the SANS total score and anhedonia subscale. In light of the lack of effect of clozapine for primary negative symptoms (12, 24, 25), how can the observation of a significant predictive relationship between right prefrontal cortex gray matter volume and negative symptoms be interpreted? One possible explanation is that clozapine may not be exerting a direct beneficial effect on negative symptoms but rather that the modest negative symptom benefit of clozapine represents a reduction in side effects and that the worsening with haloperidol represents an exacerbation of side effects. Patients with larger right prefrontal cortex gray matter volumes may be better able to take advantage of the unique pharmacological profile of clozapine and experience a greater decrease in side effects, whereas patients with larger right prefrontal cortex gray matter volumes may be more susceptible to the side effects caused by haloperidol. There are several lines of evidence that support this interpretation. First, the group differences in negative symptom effect were not due to a pronounced benefit of clozapine for these symptoms. There was only a 7.3% improvement in SANS total score in the clozapine-treated patients, whereas there was a 10.3% worsening in the haloperidol-treated patients. Second, several studies have documented a relationship between change in negative symptoms and change in extrapyramidal side effects in clozapine-treated patients. In the studies of Kane et al. (26) and Pickar et al. (27), there were marked changes in both negative symptoms and extrapyramidal side effects. Lieberman et al. (28) observed that the presence of extrapyramidal side effects during previous treatment with conventional antipsychotics was a predictor of good response to clozapine, and Rosenheck et al. (29) observed that the differential effect of clozapine on negative symptoms disappeared when the analyses were corrected for change in extrapyramidal side effects. Third, in the post hoc analyses, there was a significant interaction between right prefrontal cortex gray matter volume and treatment for the BPRS anxiety/depression factor. Marder and Van Putten (30, 31) have shown that anxiety and depression are correlated with extrapyramidal side effects and that they may in themselves represent subtle forms of extrapyramidal side effects. There was also a significant interaction for the Simpson-Angus Rating Scale akathisia item, with right prefrontal cortex gray matter volume significantly correlated with change in akathisia severity in the haloperidol-treated patients. In addition, although the interaction was not significant, right prefrontal cortex gray matter volume was significantly inversely correlated with change in Simpson-Angus Rating Scale total score (rs=–0.51, p=0.02) in the clozapine-treated patients. The correlation was nonsignificant but in the opposite direction in the haloperidol-treated patients (rs=0.21, p=0.37).
Clozapine has unique dopamine and serotonin receptor binding properties (32, 33), which may serve to mediate the observed relationship between right prefrontal cortex gray matter volume and treatment response. Specifically, there is an abundance of 5-HT1A and 5-HT2A serotonergic receptors in the prefrontal cortex. These receptors regulate dopamine release. Clozapine has a high affinity for these receptors, and it may increase prefrontal cortex dopamine release through its action at these receptors, which would counteract the adverse effects of conventional antipsychotics (34, 35).
In summary, we found that haloperidol-treated patients were more sensitive to the adverse effects of this drug if they had larger right prefrontal cortex gray matter volumes. In contrast, clozapine-treated patients were more likely to experience the benefit of the benign neurological side effect profile of this agent to the extent to which their right prefrontal cortex gray matter was larger in volume. The mechanisms underlying this relationship may be of considerable interest in developing new therapies for schizophrenia.
![]() |
![]() |
![]() |
![]() |
![]() |
Received July 15, 2002; revision received Feb. 25, 2003; accepted March 3, 2003. From the Maryland Psychiatric Research Center. Address reprint requests to Dr. Buchanan, Maryland Psychiatric Research Center, Department of Psychiatry, University of Maryland, P.O. Box 21247, Baltimore, MD 21228; [email protected] (e-mail). Supported in part by NIMH grants MH-45074 to Dr. Buchanan and MH-40279 to Dr. Carpenter.
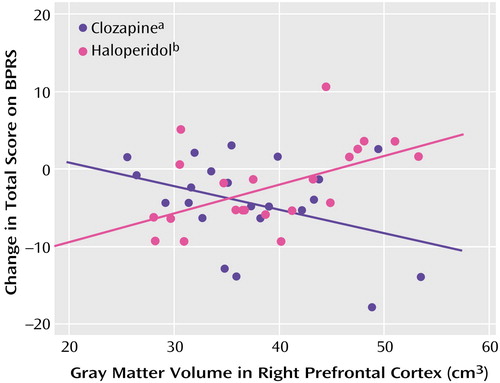
Figure 1. Relation of Change in BPRS Total Score to Right Prefrontal Cortex Gray Matter Volume in Schizophrenia Outpatients Randomly Assigned to Double-Blind Treatment With Clozapine or Haloperidol
ars=–0.29, N=22, p=0.21.
brs=0.59, N=22, p=0.005.
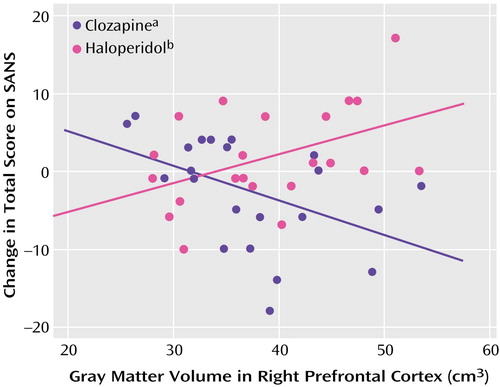
Figure 2. Relation of Change in SANS Total Score to Right Prefrontal Cortex Gray Matter Volume in Schizophrenia Outpatients Randomly Assigned to Double-Blind Treatment With Clozapine or Haloperidol
ars=–0.48, N=22, p=0.03.
brs=0.37, N=22, p=0.10.
1. Buchanan RW: Clozapine: efficacy and safety. Schizophr Bull 1995; 21:579–591Crossref, Medline, Google Scholar
2. Farde L, Nordstrom AL, Wiesel FA, Pauli S, Halldin C, Sedvall G: Positron emission tomographic analysis of central D1 and D2 dopamine receptor occupancy in patients treated with classical neuroleptics and clozapine: relation to extrapyramidal side effects. Arch Gen Psychiatry 1992; 49:538–544Crossref, Medline, Google Scholar
3. Friedman L, Knutson L, Shurell M, Meltzer HY: Prefrontal sulcal prominence is inversely related to response to clozapine in schizophrenia. Biol Psychiatry 1991; 29:865–877Crossref, Medline, Google Scholar
4. Honer WG, Smith GN, Lapointe JS, MacEwan GW, Kopala L, Altman S: Regional cortical anatomy and clozapine response in refractory schizophrenia. Neuropsychopharmacology 1995; 13:85–87Crossref, Medline, Google Scholar
5. Bilder RM, Wu H, Chakos MH, Bogerts B, Pollack S, Aronowitz J, Ashtari M, Degreef G, Kane JM, Lieberman JA: Cerebral morphometry and clozapine treatment in schizophrenia. J Clin Psychiatry 1994; 55(suppl B):53–56Medline, Google Scholar
6. Lauriello J, Mathalon DH, Rosenbloom M, Sullivan EV, Faustman WO, Ringo DL, Lim KO, Pfefferbaum A: Association between regional brain volumes and clozapine response in schizophrenia. Biol Psychiatry 1998; 43:879–886Crossref, Medline, Google Scholar
7. Molina Rodríguez V, Montz Andreé R, Pérez Castejón MJ, Capdevila García E, Carreras Delgado JL, Rubia Vila FJ: SPECT study of regional cerebral perfusion in neuroleptic-resistant schizophrenic patients who responded or did not respond to clozapine. Am J Psychiatry 1996; 153:1343–1346Link, Google Scholar
8. Molina Rodríguez V, Montz Andreé R, Pérez Castejón MJ, Zamora ML, Alvaro PC, Carreras Delgado JL, Rubia Vila FJ: Fronto-striato-thalamic perfusion and clozapine response in treatment-refractory schizophrenic patients: a 99mTc-HMPAO study. Psychiatry Res 1997; 76:51–61Crossref, Medline, Google Scholar
9. Buchanan RW, Carpenter WT Jr: The neuroanatomies of schizophrenia. Schizophr Bull 1997; 23:367–372Crossref, Medline, Google Scholar
10. Carpenter WT Jr, Buchanan RW, Kirkpatrick B, Tamminga C, Wood F: Strong inference, theory testing, and the neuroanatomy of schizophrenia. Arch Gen Psychiatry 1993; 50:825–831Crossref, Medline, Google Scholar
11. Breier A, Buchanan RW, Kirkpatrick B, Davis OR, Irish D, Summerfelt A, Carpenter WT Jr: Effects of clozapine on positive and negative symptoms in outpatients with schizophrenia. Am J Psychiatry 1994; 151:20–26Link, Google Scholar
12. Buchanan RW, Breier A, Kirkpatrick B, Ball P, Carpenter WT Jr: Positive and negative symptom response to clozapine in schizophrenic patients with and without the deficit syndrome. Am J Psychiatry 1998; 155:751–760Link, Google Scholar
13. Overall JE, Gorham DR: The Brief Psychiatric Rating Scale. Psychol Rep 1962; 10:799–812Crossref, Google Scholar
14. Andreasen NC: The Scale for the Assessment of Negative Symptoms (SANS): conceptual and theoretical foundations. Br J Psychiatry Suppl 1989; 7:49–58Medline, Google Scholar
15. Buchanan RW, Carpenter WT: Domains of psychopathology: an approach to the reduction of heterogeneity in schizophrenia. J Nerv Ment Dis 1994; 182:193–204Crossref, Medline, Google Scholar
16. Simpson GM, Angus JWS: A rating scale for extrapyramidal side effects. Acta Psychiatr Scand Suppl 1970; 212:11–19Crossref, Medline, Google Scholar
17. Breier A, Buchanan RW, Elkashef A, Munson RC, Kirkpatrick B, Gellad F: Brain morphology and schizophrenia: a magnetic resonance imaging study of limbic, prefrontal cortex, and caudate structures. Arch Gen Psychiatry 1992; 49:921–926Crossref, Medline, Google Scholar
18. Buchanan RW, Breier A, Kirkpatrick B, Elkashef A, Munson RC, Gellad F, Carpenter WT Jr: Structural abnormalities in deficit and nondeficit schizophrenia. Am J Psychiatry 1993; 150:59–65Link, Google Scholar
19. Loats H, Holcomb H: Image analysis, in Medical Radiology Innovations in Diagnostic Radiology. Edited by Anderson J. Berlin, Springer-Verlag, 1989, pp 23–45Google Scholar
20. Schad LR, Brix G, Zuna I, Harle W, Lorenz WJ, Semmler W: Multiexponential proton spin-spin relaxation in MR imaging of human brain tumors. J Comput Assist Tomogr 1989; 13:577–587Crossref, Medline, Google Scholar
21. Wolkin A, Sanfilipo M, Wolf AP, Angrist B, Brodie JD, Rotrosen J: Negative symptoms and hypofrontality in chronic schizophrenia. Arch Gen Psychiatry 1992; 49:959–965Crossref, Medline, Google Scholar
22. Scheepers FE, de Wied CC, Hulshoff Pol HE, van de Flier W, van der Linden JA, Kahn RS: The effect of clozapine on caudate nucleus volume in schizophrenic patients previously treated with typical antipsychotics. Neuropsychopharmacology 2001; 24:47–54Crossref, Medline, Google Scholar
23. Scheepers FE, Gispen de Wied CC, Hulshoff Pol HE, Kahn RS: Effect of clozapine on caudate nucleus volume in relation to symptoms of schizophrenia. Am J Psychiatry 2001; 158:644–646Link, Google Scholar
24. Kane JM, Marder SR, Schooler NR, Wirshing WC, Umbricht D, Baker RW, Wirshing DA, Safferman A, Ganguli R, McMeniman M, Borenstein M: Clozapine and haloperidol in moderately refractory schizophrenia: a 6-month randomized and double-blind comparison. Arch Gen Psychiatry 2001; 58:965–972Crossref, Medline, Google Scholar
25. Rosenheck R, Dunn L, Peszke M, Cramer J, Xu W, Thomas J, Charney D (Department of Veterans Affairs Cooperative Study Group on Clozapine in Refractory Schizophrenia): Impact of clozapine on negative symptoms and on the deficit syndrome in refractory schizophrenia. Am J Psychiatry 1999; 156:88–93Link, Google Scholar
26. Kane J, Honigfeld G, Singer J, Meltzer H (Clozaril Collaborative Study Group): Clozapine for the treatment-resistant schizophrenic: a double-blind comparison with chlorpromazine. Arch Gen Psychiatry 1988; 45:789–796Crossref, Medline, Google Scholar
27. Pickar D, Owen RR, Litman RE, Konicki E, Gutierrez R, Rapaport MH: Clinical and biologic response to clozapine in patients with schizophrenia: crossover comparison with fluphenazine. Arch Gen Psychiatry 1992; 49:345–353Crossref, Medline, Google Scholar
28. Lieberman JA, Safferman AZ, Pollack S, Szymanski S, Johns C, Howard A, Kronig M, Bookstein P, Kane JM: Clinical effects of clozapine in chronic schizophrenia: response to treatment and predictors of outcome. Am J Psychiatry 1994; 151:1744–1752Link, Google Scholar
29. Rosenheck R, Charney D, Cramer J, Xu W, Thomas J (VA CSH 17 Study Group): A randomized, double-blind trial of the efficacy and cost-effectiveness of clozapine (abstract). Schizophr Res 1997; 24:188Google Scholar
30. Marder SR, Van Putten T, Mintz J, McKenzie J, Lebell M, Faltico G, May PRA: Costs and benefits of two doses of fluphenazine. Arch Gen Psychiatry 1984; 41:1025–1029Crossref, Medline, Google Scholar
31. Van Putten T, May PR, Marder SR: Akathisia with haloperidol and thiothixene. Arch Gen Psychiatry 1984; 41:1036–1039Crossref, Medline, Google Scholar
32. Nyberg S, Nakashima Y, Nordstrom AL, Halldin C, Farde L: Positron emission tomography of in-vivo binding characteristics of atypical antipsychotic drugs: review of D2 and 5-HT2 receptor occupancy studies and clinical response. Br J Psychiatry Suppl 1996; 29:40–44Medline, Google Scholar
33. Kapur S, Zipursky RB, Remington G: Clinical and theoretical implications of 5-HT2 and D2 receptor occupancy of clozapine, risperidone, and olanzapine in schizophrenia. Am J Psychiatry 1999; 156:286–293Abstract, Google Scholar
34. Rollema H, Lu Y, Schmidt AW, Zorn SH: Clozapine increases dopamine release in prefrontal cortex by 5-HT1A receptor activation. Eur J Pharmacol 1997; 338:R3-R5Google Scholar
35. Ichikawa J, Ishii H, Bonaccorso S, Fowler WL, O’Laughlin IA, Meltzer HY: 5-HT(2A) and D(2) receptor blockade increases cortical DA release via 5-HT(1A) receptor activation: a possible mechanism of atypical antipsychotic-induced cortical dopamine release. J Neurochem 2001; 76:1521–1531Crossref, Medline, Google Scholar