Is Regionally Selective D2/D3 Dopamine Occupancy Sufficient for Atypical Antipsychotic Effect? An In Vivo Quantitative [123I]Epidepride SPET Study of Amisulpride-Treated Patients
Abstract
OBJECTIVE: Atypical antipsychotic drug treatment is clinically effective with a low risk of extrapyramidal symptoms. Explanations for the mechanism underlying this beneficial therapeutic profile of atypical over typical antipsychotic agents include 1) simultaneous antagonism of dopamine D2 and serotonin 5-HT2A receptors or 2) selective action at limbic cortical dopamine D2-like receptors with modest striatal D2 receptor occupancy. Amisulpride is an atypical antipsychotic drug with selective affinity for D2/D3 dopamine receptors and provides a useful pharmacological model for examining these hypotheses. The authors’ goal was to evaluate whether treatment with amisulpride results in “limbic selective” D2/D3 receptor blockade in vivo. METHOD: Five hours of dynamic single photon emission tomography data were acquired after injection of [123I]epidepride (approximately 150 MBq). Kinetic modeling was performed by using the simplified reference region model to obtain binding potential values. Estimates of receptor occupancy were made relative to a healthy volunteer comparison group (N=6). RESULTS: Eight amisulpride-treated patients (mean dose=406 mg/day) showed moderate levels of D2/D3 receptor occupancy in the striatum (56%), and significantly higher levels were seen in the thalamus (78%) and temporal cortex (82%). CONCLUSIONS: Treatment with amisulpride results in a similar pattern of limbic cortical over striatal D2/D3 receptor blockade to that of other atypical antipsychotic drugs. This finding suggests that modest striatal D2 receptor occupancy and preferential occupancy of limbic cortical dopamine D2/D3 receptors may be sufficient to explain the therapeutic efficacy and low extrapyramidal symptom profile of atypical antipsychotic drugs, without the need for 5-HT2A receptor antagonism.
Clinically effective antipsychotic drugs share dopamine D2 receptor antagonism. Although prospective neuroimaging studies have suggested a threshold level (>65%) of striatal D2 receptor occupancy necessary for clinical response, occupancy of striatal D2 receptors (nigrostriatal dopamine pathway) by antipsychotic drugs (>80%) seems to be a better predictor of extrapyramidal side effects than clinical response (1, 2). Schizophrenia patients show extrapyramidal side effects at high levels of striatal D2 receptor occupancy regardless of clinical response (3), and some patients resistant to typical antipsychotic treatment respond to clozapine with lower striatal D2 receptor occupancy than the suggested threshold (3–5). It has been suggested that dopamine receptors in limbic cortical areas (mesocortical dopamine pathways) are more relevant than striatal dopamine receptor populations for the pathophysiology of schizophrenia and that they mediate the therapeutic effects of antipsychotic drugs (6).
Atypical antipsychotic drug treatment is clinically useful, with a lower propensity than typical (conventional) antipsychotic treatment to induce extrapyramidal side effects. Different hypotheses have been formulated to explain atypicality. Consideration of the multiple sites of action of atypical antipsychotic drugs such as clozapine, olanzapine, risperidone, and quetiapine has led to suggestions that action at sites other than dopamine D2 receptors, in particular serotonin 5-HT2A receptors, may be necessary for an atypical clinical profile (7). Alternative hypotheses suggest that action at D2 receptors is sufficient to explain atypicality, through either fast dissociation from D2 receptors (8) or selective binding at limbic and cortical regions (9). According to the “limbic selectivity” hypothesis, atypical antipsychotic drugs induce relatively high occupancy of limbic cortical D2 receptors, which is associated with clinical efficacy, and moderate striatal occupancy, which is associated with a low incidence of extrapyramidal side effects.
In vivo neuroreceptor imaging studies have shown that therapeutic doses of typical antipsychotic drugs produce high blockade of D2-like receptors (D2/D3) equally in limbic cortical areas and the striatum and are likely to induce extrapyramidal side effects (10–12). While several atypical antipsychotics (e.g., clozapine, olanzapine, risperidone and quetiapine) show preferential occupancy of D2/D3 receptors in limbic cortical areas (11–16), all of the atypical antipsychotic drugs examined in these studies are 5-HT2A receptor antagonists, and the effects of this antagonism cannot be ruled out as a possible explanation for their clinical efficacy without causing extrapyramidal side effects.
Amisulpride is a highly selective dopamine D2-like receptor antagonist (Ki=2.8 nmol/liter for D2 receptors and Ki=3.2 nmol/liter for D3 receptors), with several orders of magnitude higher affinity for D2/D3 receptors than any other receptor population (17). A PET study of amisulpride-treated patients found no significant binding to 5-HT2A receptors (18). In clinical trials, amisulpride has shown therapeutic benefit with placebo-level extrapyramidal side effects (19). This and its highly specific receptor profile make it ideally suited to test whether antipsychotic efficacy and a low incidence of extrapyramidal side effects may be achieved purely by selective action at limbic cortical dopamine D2/D3 receptors in vivo.
For this study, we used [123I]epidepride, a high-affinity radioligand (Kd=24 pmol/liter) for striatal and extrastriatal D2 receptors, and single photon emission tomography (SPET) to evaluate in vivo striatal and corticolimbic dopamine D2/D3 receptor occupancy with amisulpride treatment.
Method
Approval for the study was obtained from the South London and Maudsley National Health Service Trust Ethics Committee and the U.K. Administration of Radioactive Substances Advisory Committee. Patients were recruited from the South London and Maudsley National Health Service Trust, London, and written informed consent was obtained after study procedures and possible side effects were explained.
Subjects
The inclusion criteria were a diagnosis of schizophrenia according to DSM-IV criteria and compliant treatment with amisulpride for more than 3 weeks before scanning. Exclusion criteria were other primary psychiatric or physical illnesses, drug or alcohol dependence syndrome, or concomitant use of another antipsychotic drug. Absence of major cognitive deficit was confirmed by using the Mini-Mental State Examination (20). Nine patients with schizophrenia took part in the study. Three patients were taking adjunctive medications. These included venlafaxine (patients 4 and 6: 150 and 225 mg/day, respectively), sodium valproate (patient 5: 1000 mg/day) and anticholinergic drugs (patient 4: procyclidine, 10 mg/day; patient 6: orphenadrine, 400 mg/day). No amisulpride was detected in a plasma sample from one patient (limit of detection of assay 10 μg/liter), who was therefore not included in the study (exclusion or inclusion of this patient did not change any of the study’s findings).
Healthy comparison subjects (N=6) were recruited from the community. The subjects were sex- and age-matched with the patients (mean age=30 years, SD=3; one subject was female). They completed a general health screening and drug and alcohol misuse checklist. Volunteers with current or previous physical or psychiatric illness including drug or alcohol dependency syndromes were excluded.
Clinical Management and Assessment
Medication was freely titrated for optimal control of symptoms. Amisulpride was the second-line drug in all cases. Clinical ratings were performed by a trained psychiatric rater (R.A.B.) at the time of the scan. In four cases, assessment was also performed before amisulpride treatment. Psychiatric symptoms were assessed with the 24-item Brief Psychiatric Rating Scale (BPRS) (21), the Positive and Negative Syndrome Scale (22), and the Calgary Depression Rating Scale for Schizophrenia (23). Global functioning was assessed with the Global Assessment Scale (GAS) (24). Extrapyramidal side effects were rated with the Simpson-Angus Rating Scale for parkinsonian symptoms (25), the subjective and objective Barnes Rating Scale for Drug-Induced Akathisia (26), and the Abnormal Involuntary Movement Scale (AIMS) for tardive dyskinesia (27).
Preparation of [123I]Epidepride
[123I]Epidepride ((S)-N-[(1-ethyl-2-pyrrolidinyl)methyl]-5-iodo- 2,3-dimethoxy-benzamide) (Kd=24 pmol/liter) was obtained from MAP Medical Technologies (Tikkakoski, Finland). The dose was calibrated to time of the scan and transported to London the day before the scan.
SPET Image Acquisition
Four fiducial markers, each filled with approximately 0.1 MBq of 123I, were attached to the subject’s head in the orbitomeatal line at the corners of the eyes and behind the ears to allow realignment of data from different imaging sessions.
Dynamic SPET studies were performed by using a Prism 3000XP triple-headed scanner equipped with a 153Gd transmission source (Philips Medical Systems, Cleveland). The three detectors were fitted with ultra-high resolution low-energy fan beam collimators (focal length=50 cm). Primary emission data were collected in a 15% wide energy window centered at 159 keV. Two 3% wide windows were placed on either side of the peak to detect scattered and high-energy photons. Projections (N=120) were acquired over 360° in 128×128 matrices with a pixel size of 3.56 mm.
An intravenous bolus injection of approximately 150 MBq of [123I]epidepride was administered. Scanning started at the time of injection and continued for 5 hours postinjection in a series of five imaging sessions with breaks in between. The first session was 60 minutes long for nine subjects and 30 minutes long for the remaining five subjects. The subsequent sessions were 30 minutes long, followed by 30-minute breaks. Data were acquired in time frames of 5 minutes for the first 40 minutes, and then in 10-minute frames for the rest of the scan. Transmission scans were performed at the end, using the simultaneous transmission emission protocol (STEP, Philips Medical Systems) with a 20% energy window centered at 100 keV.
Image Reconstruction
Image reconstruction and processing were done with in-house software implemented in C and IDL 5.1 (Interactive Data Language, Research Systems, Inc., Boulder, Colo.) and is described in more detail in Bressan et al. (16). Transmission images were reconstructed by using an ordered subset implementation of the convex maximum likelihood algorithm (28). The emission data were corrected for scatter by using the triple energy window method (29). Tomographic images were reconstructed by filtered back projection. Attenuation correction was made by the second-order Chang method on the basis of the measured transmission maps (30). The final resolution in the images was approximately 11 mm (full width at half maximum).
Kinetic Modeling
Images were analyzed by using a customized IDL 5.1 analysis platform. Regions of interest for striatum, temporal cortex, thalamus, and cerebellum were defined on integrated images corresponding to the first 60 minutes of acquisition. Regions were defined in accordance with standard anatomical sections (31) on a minimum of three slices. Regional radioactivity concentrations for each region were computed by pooling the corresponding region of interest values and by averaging the left and right regional values. Regions were applied to coregistered images to 5 hours after radioligand injection. The temporal cortical regions of interest incorporated the inferior and medial temporal cortex, including entorhinal cortex, hippocampus, and amygdala.
Time-activity curves were generated based on regions of interest. Kinetic analysis was done with the simplified reference tissue model, with the cerebellum used as a region of nonspecific binding (32). The cerebellum can be assumed to be free of D2 receptors (33) and represents background uptake (nonspecific binding plus free ligand). Time-activity curves of regions with specific binding were fitted with reference region models according to the following operational equation:
where CT(t) and CR(t) are the activity concentrations in the target and reference regions, respectively, as a function of time. R1 is the target-to-reference region delivery ratio, k2 is the rate constant for transfer from the free plus nonspecific compartment to plasma, BP is the binding potential for the target region, λ is the decay constant for 123I, and ⊗ is the convolution operator.
D2/D3 Receptor Occupancy Estimation
Estimates of receptor occupancy, O, were made relative to a normal volunteer comparison group by using the following formula:
where BPv is the mean binding potential value of the healthy volunteer group and BPp is the binding potential value of each amisulpride-treated patient.
Plasma Amisulpride Measurement
Blood samples were taken by venipuncture in the forearm and were collected in a heparinized tube before the SPET scans. The tubes were centrifuged (1,500g for 3 minutes) to separate plasma, which was in turn drawn off into a tube and stored at approximately –15°C. Plasma amisulpride was measured by high performance liquid chromatography with fluorescence monitoring (excitation=280 nm, emission=370 nm). Internal quality control samples (75 and 250 μg/liter amisulpride) were analyzed with each sample batch. The limit of accurate measurement was 1 μg/liter (200 μ/liter sample). Two samples (patient 1 and patient 4) were lost during storage and were considered missing.
Statistical Analysis
Statistical analysis was performed by using the Statistical Package for Social Sciences (SPSS) version 10.0 for Windows. Unpaired t tests were used to compare D2/D3 binding potential values for the healthy volunteers and the amisulpride-treated patients. A general linear model for repeated measures with within-subjects factor at three levels was applied to compare D2/D3 receptor occupancy in three regions evaluated: the striatum, thalamus, and temporal cortex. The striatum was used as the reference category for individual comparisons. Correlations between amisulpride daily dose and plasma concentration and regional brain D2/D3 receptor occupancy values were performed by using Spearman rank correlation coefficient (two-tailed). The occupancy versus amisulpride plasma concentration data were fitted by using a hyperbolic equation describing the binding of a ligand following the law of mass action:
where O is the receptor occupancy (%), p is the amisulpride plasma concentration (μg/liter), and a1 and a2 are free parameters. One curve was fitted to the striatal data and one to the extrastriatal data.
Results
Subject Characteristics and Clinical Assessment
Eight patients with schizophrenia and six healthy volunteers were studied. There were no significant differences in age between healthy volunteers and patients (t=–4.3, df=12, p=0.70). Table 1 shows patient’s demographic and treatment characteristics, and Positive and Negative Syndrome Scale scores on the day of the scan. Mean scores for the remaining assessments were as follows: Positive and Negative Syndrome Scale, general psychopathology: 33 (SD=10); BPRS: 46 (SD=12); Calgary Depression Rating Scale for Schizophrenia: 6 (SD=6); GAS: 51 (SD=10); Simpson-Angus Rating Scale: 1 (SD=3); Barnes Rating Scale for Drug-Induced Akathisia: 3 (SD=4); and AIMS: 2 (SD=5). Among the four patients evaluated prospectively, there were nonsignificant changes in positive, negative, depression, or general psychopathology symptoms and global assessments scales during amisulpride treatment. Two patients (patients 1 and 7) developed mild to moderate akathisia, and one patient (patient 7) developed minimal extrapyramidal symptoms. No treatment-emergent tardive dyskinesia was observed.
For the mean amisulpride dose of 406 mg/day (range=150–600 mg/day), the mean amisulpride plasma concentration was 278 μg/liter (Table 1). There was a significant correlation between amisulpride dose and plasma concentration (r=0.94, df=4, p<0.01).
Amisulpride D2/D3 Receptor Occupancy in Vivo
Figure 1 shows the concentration of D2/D3 receptors in the striatum, thalamus, and temporal cortex in a normal volunteer and in an amisulpride-treated patient, and Figure 2 shows their respective time activity curves. There was good spatial delimitation between the striatum and thalamus, and distinct time activity curves were obtained for each region. Amisulpride-treated patients had statistically significantly lower mean D2/D3 receptor binding potential than healthy volunteers in the striatum (11 [SD=5] versus 24 [SD=5], respectively; t=5.0, df=12, p<0.01), temporal cortex (0.3 [SD=0.1] versus 1.6 [SD=0.5]; t=11.6, df=12, p<0.01), and thalamus (1.0 [SD=0.5] versus 4.3 [SD=0.6]; t=7.8, df=12, p<0.01).
For a mean amisulpride dose of 406 mg/day, the mean D2/D3 receptor occupancy was 56% in the striatum, 78% in the thalamus, and 82% in the temporal cortex (Figure 3). Higher occupancy in extrastriatal than striatal regions is evident at all plasma concentrations reported (Figure 4). Multivariate tests showed significant differences in the D2/D3 receptor occupancy observed in the three regions examined (F=18.2, df=2, 7, p<0.01). Post hoc contrasts showed that D2/D3 receptor occupancy was significantly lower in the striatum than in the thalamus (F=32.0, df=1, 8, p<0.01) and temporal cortex (F=41.5, df=1, 8, p<0.01).
Positive correlations were found between amisulpride plasma concentration and occupancy values in the striatum (r=0.83, df=4, p<0.01), thalamus (r=0.83, df=4, p<0.01), and temporal cortex (r=0.89, df=4, p<0.01). Figure 4 shows the relationship between amisulpride plasma concentration and receptor occupancy in the striatum and the extrastriatal regions. Saturation of D2/D3 dopamine receptors is achieved at lower plasma amisulpride concentrations in extrastriatal regions than in the striatum.
Discussion
In this report, we studied dopamine D2/D3 receptor occupancy in vivo with the atypical antipsychotic drug amisulpride. Amisulpride is an atypical antipsychotic drug with selective action at dopamine D2/D3 receptors. At clinically useful doses, amisulpride, in common with other atypical antipsychotic drug treatment, results in high temporal cortical D2/D3 receptor occupancy and moderate striatal D2/D3 receptor occupancy. These novel quantitative SPET data concur with recent PET studies of amisulpride (34, 35) and challenge the notion that mixed antagonism of different neuroreceptor populations is an essential requirement for an atypical antipsychotic drug.
Methodological Considerations
The SPET technique has a lower spatial resolution than PET, which might result in partial volume effects. Any partial volume differences between individuals are assumed to cancel out between groups in the occupancy calculation.
In this work and in another study (16), we have used the simplified reference tissue model for quantification of receptor binding. Earlier studies with [123I]epidepride (10, 13–15) have been criticized because of the use of the ratio method for quantification of receptor binding of the tracer. Olsson and Farde (36) performed computer simulations with the high-affinity ligand [11C]-FLB 457 and PET. On the basis of their simulation results (simulating a situation in which data acquisition continued for 60 minutes after ligand injection), the authors suggested that the use of the ratio method may result in inaccurate occupancy values (36). Simulation experiments performed in our own laboratory show that the Olsson and Farde results cannot be extrapolated to the data acquisition time period actually used in the earlier epidepride studies (which was 180–240 minutes postinjection) (37). We have recently performed a methodological study based on experimental data. This study showed that the use of the ratio method with epidepride could result in a relative uncertainty in striatal occupancy estimation of about 10% (e.g., this would result in an estimated occupancy of 50% when the true value may be 55%). Both the modeling and ratio methods produce correct temporal cortical occupancy values (37). The minimal extrapyramidal side effects observed here make it highly likely that the true striatal occupancy is in any case lower than the values we obtained for the temporal cortical occupancy.
The present group of subjects was studied after a mean period of 173 days following discontinuation of their previous antipsychotic drug (which was an atypical antipsychotic in all cases). Evidence from primates treated with antipsychotic drugs at a duration and dose similar to humans suggests that D2 receptor binding to [125I]epidepride is increased, and D2 receptor mRNA turnover is also increased if animals are sacrificed and studied immediately after medication withdrawal (6). These effects are regionally selective in that haloperidol affects both cortical and striatal D2 receptors, and clozapine and remoxipride up-regulate D2 receptors only in the cortex. There are no data for animals or humans on whether these effects persist beyond 24 hours. It is unlikely that these effects would account for the regional selectivity of D2 occupancy by amisulpride found in this sample. If previous treatment with atypical antipsychotic drugs had theoretically affected D2 receptor density selectively in the cortex, our finding would have been in the opposite direction.
Two patients (patients 4 and 6) were taking venlafaxine. Antidepressants such as nortriptyline (38) or citalopram (39) do not change D2/D3 receptor occupancy values measured with [11C]raclopride. Excluding patients taking venlafaxine did not change the findings of this study. Differences in the D2/D3 receptor occupancy in the three regions examined remained significant (F=13.5, df=2, 5, p=0.01), as well as post hoc contrasts between striatum and thalamus (F=13.6, df=1, 7, p=0.01) and striatum and temporal cortex (F=32.3, df=1, 7, p<0.01).
D2/D3 Dopamine Receptor Occupancy by Amisulpride
Few studies have evaluated in vivo D2/D3 receptor blockade induced by amisulpride treatment. Martinot et al. (34) used [76Br]-bromolisuride and PET to measure striatal D2/D3 receptor occupancy in patients taking different amisulpride doses and found 12%–25% for 100 mg/day, 38%–52% for 200 mg/day, and 60% for 500 mg/day of amisulpride.
Xiberas et al. (35) used the high affinity D2/D3 receptor ligand FLB 457 labeled with 76Br, which enables longer data acquisition than the [11C]-FLB 457 (several hours). The authors investigated amisulpride-treated schizophrenia patients (doses between 200 and 600 mg/day) and found D2/D3 receptor occupancy values between 16% and 46% in the striatum, 51% and 82% in the thalamus, and 53% and 93% in temporal cortex. These D2/D3 receptor occupancy values are in agreement with those obtained in the present study (Table 1). In accordance with our study, the amisulpride plasma concentration positively correlated with occupancy values in all brain regions evaluated. Xiberas et al. (35) found that high doses of amisulpride resulted in high striatal D2/D3 receptor occupancy and extrapyramidal side effects, suggesting that preferential occupancy of temporal cortical D2/D3 receptors by amisulpride is dose dependent and that selectivity is lost at higher doses (as striatal D2/D3 receptor occupancy increases). This was confirmed in the present data (Figure 4) and is in keeping with evidence from PET studies of olanzapine and risperidone (2).
Amisulpride-treated schizophrenia patients in our study had higher D2/D3 receptor occupancy in the temporal cortex and thalamus than in the striatum, and the difference between extrastriatal and striatal regions were highly significant. This suggests that amisulpride selectively binds to limbic D2/D3 receptors, which replicates previous in vitro studies with amisulpride (17) and in vivo PET and SPET studies with other atypical antipsychotic drugs (11–16).
Relevance of the Findings to Hypotheses of Atypical Antipsychotic Drug Action
The fact that amisulpride is virtually devoid of affinity for receptor sites other than D2/D3 receptors confirms suggestions that combined 5-HT2A/D2 antagonism is not essential for atypical antipsychotic actions (8, 19). The data from this study suggest that selective occupancy of dopamine D2/D3 receptors is sufficient to explain the action of amisulpride. Kapur and Seeman (8) suggested that low affinity and fast dissociation from D2 receptors are critical for atypicality. Atypical antipsychotic drugs including amisulpride have higher affinity constants than dopamine itself and may be easily displaced by endogenous dopamine (40). Strange (9) suggests the mechanism underlying a limbic cortical selective action of atypical antipsychotic drugs is related to regional tissue dopamine concentration relative to receptor density, and intra- or extrasynaptic receptor localization. In areas with higher dopamine concentrations such as the striatum, dopamine could displace the antipsychotic drug from the receptor, resulting in apparent lower D2/D3 receptor occupancy by these drugs. However, in areas with lower dopamine concentration (such as the cortex), there is less competition with dopamine for binding to the receptor. This leads to greater occupancy of dopamine D2/D3 receptors by the atypical antipsychotic drug.
Further research is necessary to better clarify the exact neurochemical basis of “limbic selective” D2/D3 receptor occupancy by atypical antipsychotic drugs. Our findings provide further impetus for antipsychotic drug development based solely on selective action on the dopamine system by exploiting characteristics associated with modest, partial, or inverse antagonism of dopamine D2-like receptors or selectively targeting D2-like receptor subtypes involved in regulating or modulating aberrant dopamine release.
![]() |
Presented in part at the summer meeting of the British Association of Psychopharmacology, Harrogate, U.K., July 21–24, 2002; the 11th Biennial Winterworkshop on Schizophrenia Research, Davos, Switzerland, Feb. 24–April 1, 2002; the 57th annual meeting of the Society of Biological Psychiatry, Philadelphia, May 16–18, 2002; and the Neuroreceptor Mapping Conference, Oxford, U.K., July 19–22, 2002. Received Aug. 16, 2002; revision received Jan. 16, 2003; accepted March 19, 2003. From the Section of Neurochemical Imaging, Institute of Psychiatry, King’s College London; the Institute of Nuclear Medicine, Middlesex Hospital, University College London Medical School, London; and the Medical Toxicology Unit, Guy’s and St Thomas’ Hospital Trust, London. Address reprint requests to Dr. Bressan, Section of Neurochemical Imaging, Division of Psychological Medicine, Institute of Psychiatry, De Crespigny Park, Denmark Hill, London, SE5 8AF, U.K.; [email protected] (e-mail). Supported by a charitable educational grant from Sanofi Synthelabo during data collection (Dr. Bressan); a charitable educational grant from Astra-Zeneca (Dr. Jones); and a U.K. Medical Research Council (MRC) Senior Clinical Research Fellowship (Dr. Erlandsson). Dr. Pilowsky is a U.K. MRC Senior Clinical Research Fellow. The authors thank Dr. J.M. Stone for suggestions regarding this study.
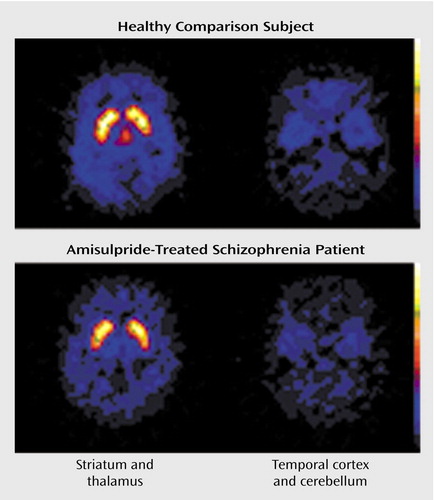
Figure 1. D2/D3 Receptor Concentrations in the Striatum, Thalamus, and Temporal Cortex of a Healthy Comparison Subject and an Amisulpride-Treated Schizophrenia Patienta
aReceptor concentrations determined through single photon emission tomography scans after injection of [123I]epidepride. The healthy volunteer scan reveals high tracer binding to D2/D3 receptors in the striatum, thalamus, and temporal cortex (in relation to the cerebellum, which has a very low signal). In the amisulpride-treated schizophrenia patient, D2/D3 receptor occupancy by the drug results in moderate attenuation of the signal in the striatum and marked attenuation in the thalamus and temporal cortex relative to the cerebellum.
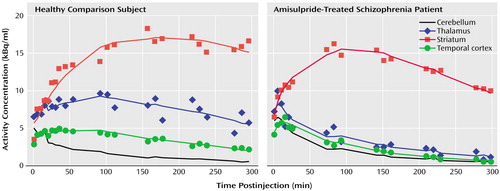
Figure 2. Time Activity Curves of [123I]Epidepride in the Striatum, Thalamus, and Temporal Cortex of a Healthy Comparison Subject and an Amisulpride-Treated Schizophrenia Patient During SPET Imaging
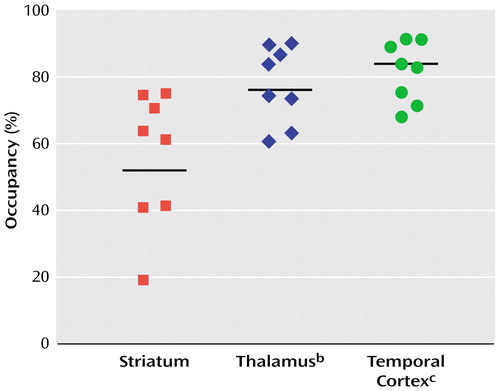
Figure 3. D2/D3 Receptor Occupancy in the Striatum and Extrastriatal Brain Regions of Amisulpride-Treated Schizophrenia Patients (N=8)a
aReceptor occupancy determined through single photon emission tomography scans after injection of [123I]epidepride. Horizontal lines represent mean receptor occupancy for the region.
bSignificantly higher occupancy than striatum (F=24.2, df=1, 7, p<0.01).
cSignificantly higher occupancy than striatum (F=31.6, df=1, 7, p<0.01).
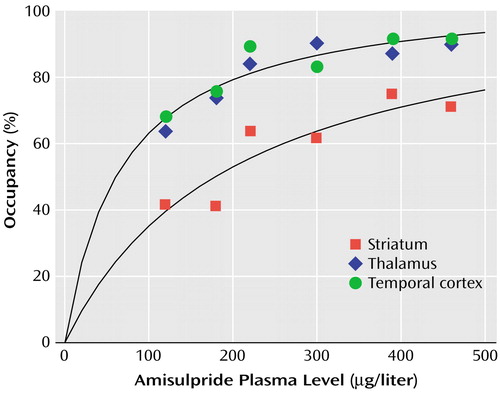
Figure 4. Relationship Between Amisulpride Plasma Concentration and D2/D3 Receptor Occupancy in the Striatum and Extrastriatal Regions of Amisulpride-Treated Schizophrenia Patients (N=6)a
aThe solid lines represent curves fitted to the data by using a hyperbolic equation describing the binding of a ligand following the law of mass action, as detailed in the Method section (for striatum: a 1=107%, a2=206 μg/liter; for the extrastriatal regions: a1=106%, a2=68.2 μg/liter). Higher occupancy in extrastriatal than striatal regions is evident at all plasma concentrations reported.
1. Nordstrom AL, Farde L, Wiesel FA, Forslund K, Pauli S, Halldin C, Uppfeldt G: Central D2-dopamine receptor occupancy in relation to antipsychotic drug effects: a double-blind PET study of schizophrenic patients. Biol Psychiatry 1993; 33:227–235Crossref, Medline, Google Scholar
2. Kapur SJ, Zipursky R, Jones C, Remington G, Houle S: Relationship between dopamine D2 occupancy, clinical response, and side effects: a double-blind PET study of first-episode schizophrenia. Am J Psychiatry 2000; 157:514–520Link, Google Scholar
3. Pilowsky LS, Costa DC, Ell PJ, Murray RM, Verhoeff NP, Kerwin RW: Antipsychotic medication, D2 dopamine receptor blockade and clinical response: a 123I IBZM SPET study. Psychol Med 1993; 23:791–797Crossref, Medline, Google Scholar
4. Pilowsky LS, Costa DC, Ell PJ, Murray RM, Verhoeff NP, Kerwin RW: Clozapine, single photon emission tomography, and the D2 dopamine receptor blockade hypothesis of schizophrenia. Lancet 1992; 340:199–202Crossref, Medline, Google Scholar
5. Farde L, Nordstrom AL, Wiesel FA, Pauli S, Halldin C, Sedvall G: Positron emission tomographic analysis of central D1 and D2 dopamine receptor occupancy in patients treated with classical neuroleptics and clozapine: relation to extrapyramidal side effects. Arch Gen Psychiatry 1992; 49:538–544Crossref, Medline, Google Scholar
6. Lidow MS, Goldman-Rakic PS: A common action of clozapine, haloperidol, and remoxipride on D1- and D2-dopaminergic receptors in the primate cerebral cortex. Proc Natl Acad Sci USA 1994; 91:4353–4356Crossref, Medline, Google Scholar
7. Meltzer HY: New insights into schizophrenia through atypical antipsychotic drugs. Neuropsychopharmacology 1988; 1:193–196Crossref, Medline, Google Scholar
8. Kapur S, Seeman P: Does fast dissociation from the dopamine D2 receptor explain the action of atypical antipsychotics? a new hypothesis. Am J Psychiatry 2001; 158:360–369Link, Google Scholar
9. Strange PG: Antipsychotic drugs: importance of dopamine receptors for mechanisms of therapeutic actions and side effects. Pharmacol Rev 2001; 53:119–133Medline, Google Scholar
10. Bigliani V, Mulligan RS, Acton PD, Visvikis D, Ell PJ, Stephenson C, Kerwin RW, Pilowsky LS: In vivo occupancy of striatal and temporal cortical D2/D3 dopamine receptors by typical antipsychotic drugs: [123I]epidepride SPET study. Br J Psychiatry 1999; 175:231–238Crossref, Medline, Google Scholar
11. Xiberas X, Martinot JL, Mallet L, Artiges E, Loc’h C, Maziere B, Paillère-Martinot ML: Extrastriatal and striatal D2 dopamine receptor blockade with haloperidol or new antipsychotic drugs in patients with schizophrenia. Br J Psychiatry 2001; 179:503–508Crossref, Medline, Google Scholar
12. Kessler RM, Ansari MS, Li R, Dawant B, Lee M, Meltzer HY: Occupancy of striatal and extrastriatal dopamine D2 receptors by atypical antipsychotic drugs. J Nucl Med 2002; 43:15PMedline, Google Scholar
13. Pilowsky LS, Mulligan RS, Acton PD, Ell PJ, Costa DC, Kerwin RW: Limbic selectivity of clozapine. Lancet 1997; 350:490–491Crossref, Medline, Google Scholar
14. Bigliani V, Mulligan RS, Acton PD, Ohlsen RI, Pike VW, Ell PJ, Gacinovic S, Kerwin RW, Pilowsky LS: Striatal and temporal cortical D2/D3 receptor occupancy by olanzapine and sertindole in vivo: a [123I]epidepride SPET study. Psychopharmacology (Berl) 2000; 150:132–140Crossref, Medline, Google Scholar
15. Stephenson CM, Bigliani V, Jones HM, Mulligan RS, Acton PD, Visvikis D, Ell PJ, Kerwin RW, Pilowsky LS: Striatal and extra-striatal D2/D3 dopamine receptor occupancy by quetiapine in vivo: [123I]-epidepride single photon emission tomography (SPET) study. Br J Psychiatry 2000; 177:408–415Crossref, Medline, Google Scholar
16. Bressan RA, Erlandsson K, Jones HM, Mulligan RS, Ell PJ, Pilowsky LS: Optimizing limbic selective D2/D3 receptor occupancy by risperidone: a [123I]-epidepride SPET study. J Clin Psychopharmacol 2003; 23:5–14Crossref, Medline, Google Scholar
17. Schoemaker H, Claustre Y, Fage D, Rouquier L, Chergui K, Curet O, Oblin A, Gonon F, Carter C, Benavides J, Scatton B: Neurochemical characteristics of amisulpride, an atypical dopamine D2/D3 receptor antagonist with both presynaptic and limbic selectivity. J Pharmacol Exp Ther 1997; 280:83–97Medline, Google Scholar
18. Trichard C, Paillère-Martinot M-L, Attar-Levy D, Recassens C, Monnet F, Martinot J-L: Binding of antipsychotic drugs to cortical 5-HT2A receptors: a PET study of chlorpromazine, clozapine, and amisulpride in schizophrenic patients. Am J Psychiatry 1998; 155:505–508Link, Google Scholar
19. Leucht S, Pitschel-Walz G, Engel RR, Kissling W: Amisulpride, an unusual “atypical” antipsychotic: a meta-analysis of randomized controlled trials. Am J Psychiatry 2002; 159:180–190Link, Google Scholar
20. Folstein MF, Folstein SE, McHugh PR: “Mini-Mental State”: a practical method for grading the cognitive state of patients for the clinician. J Psychiatr Res 1975; 12:189–198Crossref, Medline, Google Scholar
21. Overall JE, Gorham DR: The Brief Psychiatric Rating Scale. Psychol Rep 1962; 10:799–812Crossref, Google Scholar
22. Kay SR, Fiszbein A, Opler LA: The Positive and Negative Syndrome Scale (PANSS) for schizophrenia. Schizophr Bull 1987; 13:261–276Crossref, Medline, Google Scholar
23. Addington D, Addington J, Maticka-Tyndale E, Joyce J: Reliability and validity of a depression rating scale for schizophrenics. Schizophr Res 1992; 6:201–208Crossref, Medline, Google Scholar
24. Endicott J, Spitzer RL, Fleiss JL, Cohen J: The Global Assessment Scale: a procedure for measuring overall severity of psychiatric disturbance. Arch Gen Psychiatry 1976; 33:766–771Crossref, Medline, Google Scholar
25. Simpson GM, Angus JWS: A rating scale for extrapyramidal side effects. Acta Psychiatr Scand Suppl 1970; 212:11–19Crossref, Medline, Google Scholar
26. Barnes TRE: A rating scale for drug-induced akathisia. Br J Psychiatry 1989; 154:672–676Crossref, Medline, Google Scholar
27. Guy W (ed): ECDEU Assessment Manual for Psychopharmacology: Publication ADM 76–338. Washington, DC, US Department of Health, Education, and Welfare, 1976, pp 534–537Google Scholar
28. Lange K, Fessler JA: Globally convergent algorithms for maximum a posteriori transmission tomography. IEEE Trans Image Process 1995; 4:1430–1438Crossref, Medline, Google Scholar
29. Ogawa K, Harata Y, Ichihara T, Kubo A, Hashimoto S: A practical method for position-dependent Compton-scatter correction in single emission CT. IEEE Trans Med Imaging 2002; 10:408–412Crossref, Google Scholar
30. Chang LT: A method for attenuation correction in radionuclide computed tomography. IEEE Trans Nucl Sci 1978; 25:638–643Crossref, Google Scholar
31. Talairach J, Tournoux P: Co-Planar Stereotaxic Atlas of the Human Brain: Three-Dimensional Proportional System. New York, Thieme Medical, 1988Google Scholar
32. Lammertsma AA, Hume SP: Simplified reference tissue model for PET receptor studies. Neuroimage 1996; 4:153–158Crossref, Medline, Google Scholar
33. Camps M, Cortes R, Gueye B, Probst A, Palacios JM: Dopamine receptors in human brain: autoradiographic distribution of D2 sites. Neuroscience 1989; 28:275–290Crossref, Medline, Google Scholar
34. Martinot JL, Paillère-Martinot ML, Poirier MF, Dao-Castellana MH, Loc’h C, Maziere B: In vivo characteristics of dopamine D2 receptor occupancy by amisulpride in schizophrenia. Psychopharmacology (Berl) 1996; 124:154–158Crossref, Medline, Google Scholar
35. Xiberas X, Martinot JL, Mallet L, Artiges E, Canal M, Loc’h C, Maziere B, Paillère-Martinot ML: In vivo extrastriatal and striatal D2 dopamine receptor blockade by amisulpride in schizophrenia. J Clin Psychopharmacol 2001; 21:207–214Crossref, Medline, Google Scholar
36. Olsson H, Farde L: Potentials and pitfalls using high affinity radioligands in PET and SPET determinations on regional drug induced D2 receptor occupancy—a simulation study based on experimental data. Neuroimage 2001; 14:936–945Crossref, Medline, Google Scholar
37. Erlandsson K, Bressan RA, Mulligan RM, Ell PJ, Cunningham VJ, Pilowsky LS: Analysis of D2 dopamine receptor occupancy with quantitative SPET using the high-affinity ligand [123I]epidepride: resolving conflicting findings. Neuroimage (in press)Google Scholar
38. Farde L, Wiesel FA, Halldin C, Sedvall G: Central D2-dopamine receptor occupancy in schizophrenic patients treated with antipsychotic drugs. Arch Gen Psychiatry 1988; 45:71–76Crossref, Medline, Google Scholar
39. Tiihonen J, Kuoppamki M, Nagren K, Bergman J, Eronen E, Syvalahti E, Hietala J: Serotonergic modulation of striatal D2 dopamine receptor binding in humans measured with positron emission tomography. Psychopharmacology (Berl) 1996; 126:277–280Crossref, Medline, Google Scholar
40. Seeman P: Atypical antipsychotics: mechanism of action. Can J Psychiatry 2002; 47:27–38Medline, Google Scholar