A PET Study of the Pathophysiology of Negative Symptoms in Schizophrenia
Abstract
OBJECTIVE: Positron emission tomography (PET) was used to compare cerebral metabolic patterns in schizophrenic subjects with predominantly negative symptoms (alogia, affective flattening, avolition, and attentional impairment) and in those with predominantly positive symptoms. METHOD: Fourteen right-handed male subjects with DSM-IV schizophrenia were assigned to groups with predominantly negative or predominantly positive symptoms on the basis of their post-drug-washout scores on the Positive and Negative Syndrome Scale. The patients were compared to seven age- and gender-matched normal volunteers. PET scans with [18F]fluorodeoxyglucose were obtained during a degraded Continuous Performance Task to measure absolute glucose metabolic rates. Statistical parametric mapping was used to estimate the regional metabolic differences between groups. RESULTS: The subjects with predominantly negative symptoms had significant differences in glucose metabolic rates, compared to both the subjects with predominantly positive symptoms and the normal subjects. Negative symptom subjects had a lower glucose metabolic rate in the right hemisphere, especially in the temporal and ventral prefrontal cortices, compared to the other groups, and higher metabolic rates in the cerebellar cortex and in the lower deep cerebellar nuclei. Negative symptom subscale scores were negatively correlated with glucose metabolic rates for most of the brain areas that differentiated subjects with predominantly negative symptoms from those with predominantly positive symptoms. CONCLUSIONS: Schizophrenic subjects with predominantly negative symptoms have greater metabolic abnormalities than subjects with predominantly positive symptoms, particularly in frontal, temporal, and cerebellar circuitry. These results are consistent with abnormalities in corticocortical, corticobasal ganglia, mesocortical dopamine, and cerebellar-thalamic-prefrontal circuits, which may underlie the negative symptoms of schizophrenia.
The negative symptom constellation in schizophrenia includes psychomotor retardation, avolition, apathy, anhedonia, attentional impairment, and decreased emotional expression (1, 2). Negative symptoms are associated with poor premorbid function, the male sex, and a low IQ (3) and are correlated with poor outcome (4). Mueser et al. (5) found that, compared to subjects with less severe negative symptoms, subjects with high levels of enduring negative symptoms (measured with the Scale for the Assessment of Negative Symptoms and the Quality of Life Scale) had a poorer prognosis and had consistently worse social adjustment, quality of life, and severity of thought disorder. A review of the literature by McGlashan and Fenton (6) suggested that negative symptoms are “semi-independent” of positive symptoms and that, although negative symptoms are variable early in the illness, they accrue in severity, stability, and prognostic weight over time. Conventional antipsychotics have little effect on negative symptoms (3, 7).
Since 1981, more than 100 articles on functional brain imaging in schizophrenia have been published. Positron emission tomography (PET) and single photon emission computed tomography (SPECT), which measure regional cerebral blood flow (rCBF) and/or metabolism, reveal a number of abnormalities and deficiencies in persons with schizophrenia, compared with healthy subjects. A consistent finding (in two-thirds of the published PET or SPECT studies) is a lower level of prefrontal cortical metabolism in schizophrenic subjects (reviewed by Wu et al. [8]). Kishimoto et al. (9) found hyperactive thalamic and cingulate areas in never-medicated patients with acute schizophrenia and hypoactive associative frontal, parietal, and temporal gyri in never-medicated patients with chronic schizophrenia. Kim et al. (10) found lower prefrontal and higher thalamic and cerebellar rCBF in both drug-naive and chronically ill patients with schizophrenia, suggesting that these effects were not related to chronicity of illness and were not a consequence of treatment with medication.
Negative symptoms in schizophrenia have been associated with structural impairment in the prefrontal cortex and have been hypothesized to arise from a central hypodopaminergic substrate (11). By using [99mTc] hexamethylpropyleneamine xomine ([99mTc]HMPAO) brain SPECT, Sabri et al. (12) found that negative symptom scores on the Positive and Negative Syndrome Scale (13) were negatively correlated with bifrontal, bitemporal, cingulate, basal ganglia, and thalamic rCBF (r=–0.59 to –0.74). By applying the same functional imaging technique, Molina Rodriguez et al. (14) found that negative symptom scores correlated negatively with prefrontal perfusion. PET scans of subjects with predominantly negative symptoms have revealed a prominent hypofrontality (15). We were interested in extending previous studies to identify the neuronal circuitry associated with negative symptoms.
Method
Sample
Fourteen male subjects participated in this study. All subjects fulfilled the DSM-IV criteria for schizophrenia. After a complete description of the study to the subjects, written informed consent was obtained.
Study Design
The study subjects were drawn from among symptomatic patients with schizophrenia who participated in a medication washout period in one of three medication clinical trials for treatment of acute symptoms. The three trials (designed by R.A.) were similar except that one included only patients with negative symptoms. The subjects were assigned to groups with predominantly negative or predominantly positive symptoms on the basis of their post-drug-washout scores on the Positive and Negative Syndrome Scale. The subjects with predominantly negative symptoms had a greater score on the negative than on the positive subscale of the Positive and Negative Syndrome Scale, a negative subscale score >20, at least a moderate global negative symptom score, and an absence of depression. Absence of depression was determined by the Positive and Negative Syndrome Scale depression item and/or clinical examination. Subjects with mild depression could be included. The subjects with predominantly positive symptoms consisted of consecutive right-handed male patients from the other two clinical trials with an equivalent medication washout period. They had Positive and Negative Syndrome Scale positive subscale scores that were greater than their negative subscale scores. Subjects who met the criteria for treatment-refractory schizophrenia (16) were excluded. According to these criteria, seven subjects were classified into the predominantly negative symptoms group and seven into the predominantly positive symptoms group.
Seven age- and gender-matched right-handed male volunteers without a history of psychiatric illness were recruited through advertisement. These subjects were interviewed by a psychiatrist to determine their psychiatric and medical history and current status.
Statistical Analyses
For contrasting baseline characteristics, two-tailed Student’s t test or analyses of variance were performed. Pearson’s product moment correlations were calculated to compare clinical symptom severity scores with regional brain metabolism.
[18F]Fluorodeoxyglucose PET
[18F]Fluorodeoxyglucose (FDG) PET scans during a degraded Continuous Performance Task (17) were obtained for the 14 schizophrenic subjects at baseline, after a 5-day drug washout period. In this task, subjects viewed a series of blurred numbers presented a few seconds apart for 30 minutes. Target numbers appeared at intervals, and the subjects were required to remain vigilant to detect these targets and respond by pressing a button. The PET scans were conducted with a GE 2048 dedicated head scanner (General Electric, Milwaukee) at the University of California, Irvine, Brain Imaging Center. Thirty slices at 6.5-mm intervals (15 in each of two sets) were obtained to cover the entire brain. Scans were reconstructed with a blank and a transmission scan. The transmission scan was used as an attenuation correction for scan reconstruction. A thermosetting plastic face mask was used to hold the subject stationary during the 60 minutes of total image acquisition. The rate of glucose metabolism was calculated on a three-constant model by using arterialized blood samples from the warmed arm, following the procedure used in the validation studies of Phelps et al. (18).
Differences in regional FDG uptake were analyzed by using statistical parametric mapping software (SPM 99) (Wellcome Department of Cognitive Neurology, University College, London) implemented on the Matlab platform (MathWorks Inc., Sherborn, Mass.). Statistical parametric maps are spatially extended statistical processes constructed to test hypotheses about regionally specific effects in neuroimaging data. Statistical parametric mapping combines the general linear model and the theory of Gaussian random fields to make statistical inferences about regional effects (19). The images were spatially normalized by using a 12-parameter affine transformation estimated by minimizing the residual sum of squares between each scan and a reference or template image conforming to the standard space defined by Talairach and Tournoux (20). The original image matrix obtained at 256×256×30 with a voxel size of 4.5×4.5×6.5 mm plane separation was transformed and resliced to more closely match the Talairach standard anatomical space. The images were smoothed with an isotropic Gaussian kernel (5 mm full width at half maximum), as suggested by Poline et al. (21). Before statistical inferences were made, images were scaled to a canonical mean global activity. The design matrix included global activity and D′ scores as covariates in the analysis to account for any differences in glucose metabolic rate and Continuous Performance Task performance between subjects. To test our hypotheses regarding regionally specific condition effects, the estimates were compared by using linear contrasts. The resulting set of voxel values for each contrast represents a parametric mapping of the t statistics, SPM(t), which were transformed into the unit normal distribution SPM(z) and thresholded at t=2.26, p<0.025, uncorrected (22). The resulting foci were then characterized in terms of peak height (u). The significance of each region was estimated on the basis of the probability that the observed peak height could have occurred by chance (probability that zmax>u) (22). A Bonferroni correction for multiple comparisons was not appropriate because the maximum intensity and the spatial extent of the region above u were not independent. Spatial extent thresholding based on the Gaussian random field theory was performed at 35 voxels to correct for multiple comparisons.
Anatomical localization of significant regions was accomplished through comparison with the Talairach and Tournoux atlas and confirmation by a neuroanatomist (J.H.F.) who was blind to group assignments. The Talairach and Tournoux coordinates are provided in parentheses after the names of the relevant brain regions. It should be kept in mind, however, that current PET imaging technology does not allow for precise anatomical localizations to specific subcortical nuclei and sublobular cortical areas. Some approaches have applied coregistration within individual MRI templates to aid in verifying anatomical localization, but such templates were not available in this study. The localization of brain regions by the neuroanatomist (J.H.F.) aided in anatomical verification by employing dynamic interpretation of brain circuits on the basis of known anatomical structures and by following changes in regional metabolism between adjacent slices.
Results
The mean ages of the study groups were 38.6 years (SD=7.5) for the subjects with predominantly negative symptoms, 40.6 years (SD=11.0) for the subjects with predominantly positive symptoms, and 38.0 years (SD=17.0) for the comparison subjects (F=0.09, df=2, 18, p=0.92). The negative symptom subjects had been ill for a mean of 14.5 years (SD=8.4), compared to 18.9 years (SD=12.0) for the positive symptom subjects (t=–0.77, df=12, p=0.45). All subjects were right-handed. All negative symptom subjects were classified as having the undifferentiated subtype of schizophrenia according to DSM-IV criteria. Three positive symptom subjects were also classified as having the undifferentiated subtype; the other four were classified as having the paranoid subtype. Half of the patients had previously received conventional neuroleptics (four negative symptom subjects and three positive symptom subjects), and the other half had received atypical antipsychotics (three negative symptom subjects and four positive symptom subjects). The mean number of days in the medication washout period was 4.3 (SD=0.5) for the negative symptom subjects and 5.4 (SD=1.5) for the positive symptom subjects (t=–1.6, df=12, p=0.14).
All negative symptom subjects, but none of the positive symptom subjects, had Positive and Negative Syndrome Scale negative symptom subscale scores >25. All positive symptom subjects, but none of the negative symptom subjects, had Positive and Negative Syndrome Scale positive symptom subscale scores >20.
All significant differences were based on p values <0.025, corrected for multiple comparisons. As expected, the schizophrenic subjects had poorer performance than the comparison group on the Continuous Performance Task, although this difference did not reach statistical significance (D′=2.24 versus 2.77; t=–0.98, df=19, p=0.34). The negative symptom subjects did not differ significantly in performance from the positive symptom subjects (D′=1.81 versus 2.68; t=1.35, df=12, p=0.21). Although no statistically significant group differences in performance were found, the imaging results were covaried for Continuous Performance Task performance in the statistical parametric mapping analysis to take into account individual differences.
Comparison of Patients and Healthy Subjects
In general, the schizophrenic subjects with predominantly negative symptoms and those with predominantly positive symptoms had higher regional glucose metabolic rates than the healthy comparison group (Figure 1 and Table 1). In both groups of subjects with schizophrenia, the higher levels of metabolism were more marked in the right hemisphere in the dorsal prefrontal cortex (Brodmann’s area 9), frontal eye fields (Brodmann’s area 8), motor and premotor cortices (Brodmann’s area 4, 6), supramarginal/angular gyrus (Brodmann’s area 40), and posterior cingulate (Brodmann’s area 23). Both groups of schizophrenic subjects had higher levels of metabolism in the left hemisphere in the ventral posterior occipitotemporal cortex (Brodmann’s area 21 at the border of area 37) and the amygdala and bilaterally in the anterior medial orbital cortex, cuneus, and neocerebellar cortex. Both groups of patients had lower levels of metabolism than the comparison subjects in the lateral occipitotemporal junction (Brodmann’s area 21 at the border of area 37), right anterior dorsolateral prefrontal cortex (Brodmann’s area 9/46), and right dorsal supramarginal gyrus (Brodmann’s area 40).
In addition to the differences between both groups of schizophrenic subjects and the comparison group, distinctions between each group of schizophrenic subjects and the comparison group were observed. In general, the positive symptom subjects tended to have a higher glucose metabolic rate than the comparison subjects in the frontal and temporal lobes, where the negative symptom subjects tended to have a lower level of metabolism than the comparison subjects (Figure 1 and Table 1). The positive symptom subjects had numerous areas with higher levels of metabolism relative to the comparison group throughout the posterior two-thirds of the right and left temporal lobes, where the negative symptom subjects generally had lower levels of metabolism. The positive symptom subjects had higher levels of metabolism relative to the comparison group in the prefrontal cortex. The negative symptom subjects had lower levels of metabolism in this area, especially in the middle and posterior inferior frontal gyri (which are associated with motor and premotor speech). Subcortically, both groups of schizophrenic subjects had higher levels of metabolism in portions of the left amygdala, but only the subjects with predominantly positive symptoms had higher levels of metabolism in the expanse of basal forebrain that includes the substantia innominata, extended amygdala, ventral striatum and ventral pallidum, and bed nucleus of stria terminalis. In addition, only the positive symptom subjects had higher levels of metabolism in the posterior head of the caudate and putamen bilaterally, especially on the right side in the ventral two-thirds of the striatal complex (data not shown). Finally, the negative symptom subjects, but not the positive symptom subjects, had a lower metabolic rate, relative to the comparison group, in the left posterior thalamus.
Comparison of Patient Groups
The regional metabolic profile for the negative symptom subjects was different from that of the positive symptom subjects (Figure 2, Figure 3, and Table 2). In general, the negative symptom subjects had a lower glucose metabolic rate in the right hemisphere, especially in the ventral half of the cerebrum. Thus, there was a lower level of metabolism in the ventral half of the prefrontal and occipital cortex and broad sectors of the entire temporal lobe in the negative symptom subjects. On the other hand, the negative symptom subjects had a higher metabolic rate in dorsal occipital posterior parietal cortices as well as in the cerebellar cortex.
The negative symptom subjects had a lower glucose metabolic rate than the positive symptom subjects in broad areas of the frontal lobe, including the left and right frontopolar cortex (Brodmann’s area 10), left medial prefrontal cortex (Brodmann’s area 32), left anterior inferior frontal gyrus (Brodmann’s area 47), posterior inferior frontal gyrus bilaterally (Brodmann’s area 44, 45), right dorsolateral prefrontal cortex (Brodmann’s area 46/9), right premotor cortex (Brodmann’s area 6), and ventral-most motor cortex (transcortical speech area) on the right side (Brodmann’s area 43). In the parietal lobe, the negative symptom subjects had a higher metabolic rate in the right angular gyrus (Brodmann’s area 39) and precuneus bilaterally (Brodmann’s area 7/31) and a lower metabolic rate in the right posterior cingulate (Brodmann’s area 23) and right retrosplenial cortex (Brodmann’s area 29, 30). In the occipital lobe, the negative symptom subjects had a higher metabolic rate in the cuneus (Brodmann’s area 17, 18, 19: dorsal stream) but a lower metabolic rate in the lingual gyrus (Brodmann’s area 17, 18, 19: ventral stream). In the occipitotemporal convergence zone (Brodmann’s area 37), the negative symptom subjects had a higher metabolic rate in areas on the left side but a lower rate on the right side. In the temporal lobe, the negative symptom subjects had a lower metabolic rate in the middle (Brodmann’s area 21) and inferior temporal gyrus (Brodmann’s area 20), including the fusiform gyrus. In the cerebellum, the negative symptom subjects had a higher metabolic rate in the neocerebellar cortex but a lower rate bilaterally in the deep cerebellar nuclei. Subcortically, the only differences between the two patient groups were in the posterior lateral thalamus on the left side, with the negative symptom subjects having a lower level of metabolism in this area.
Correlations of Symptoms With Regional Brain Metabolism
The Positive and Negative Syndrome Scale negative symptom subscale scores were correlated with glucose metabolic rates in the regions that distinguished the positive symptom subjects from the negative symptom subjects (Table 2). No significant correlations were observed for the Positive and Negative Syndrome Scale total scores or positive symptom subscale scores. In addition, we applied the five-factor model of Undenmayer et al. (23) to the items from the Positive and Negative Syndrome Scale. The positive, excitement, cognitive, and depression/anxiety factors did not significantly correlate with glucose metabolic rates. In contrast, the negative symptom factor was significantly correlated with glucose metabolic rate in many of the brain areas that distinguished the positive symptom subjects from the negative symptom subjects. The Lindenmayer negative symptom factor correlation coefficients were very similar to the correlation coefficients achieved for the two-factor negative symptom scale (data not shown).
Discussion
Methodological Considerations
The lack of precise anatomical localization with PET is a recognized problem. Anatomical localization can be addressed by using atlas brain coordinates or by using individual MRI templates coregistered with individual PET scans. However, individual MRI templates were not available for this study. We used statistical parametric mapping analysis with Talairach and Tournoux coordinates (20) for localization, but this method does not consider continuity of structure and shape analysis in identifying anatomical coordinates and associated structural nomenclature. Thus, an additional independent analysis of anatomical localization was done by a neuroanatomist (J.H.F.) who was blind to the subjects’ group assignments. The neuroanatomist used known locations and considered three-dimensional shapes spanning adjacent slices.
Anatomical localizations resulting from both the statistical parametric mapping analysis and the neuroanatomist’s analysis are presented in Table 1 and Table 2. Eighty percent of the localizations developed by the two methods were equivalent. Five percent were not equivalent because of different designations of the border between the cerebellar cortex and the inferomedial occipitotemporal cortex. In these cases, the neuroanatomist considered the continuity of the activation signals across three to five sections that revealed “migration” of signals in two directions—one toward an unequivocal cerebellum and the other toward an unequivocal occipitotemporal cortex. Thus, in the statistical parametric mapping analysis that used Talairach coordinates, localization of the signal at the single local maximum within a cluster underestimated the true extent of the entire signal. In the other cases of disagreement (mostly in the temporal lobe), the maximum resulting from the statistical parametric mapping analysis with Talairach coordinates was located at the border of two cortical areas, e.g., at the depth of a sulcus or at the border of white matter and an identified structure. Again, the neuroanatomist followed the activation signal through several adjacent sections and compared the result to the maximum inside the cluster derived from the statistical parametric mapping analysis. The single local maximum was within the anatomical area but did not represent the entire activation cluster as one moved from anatomical section to section.
Partial volume effects, due to enlarged ventricles or cortical thinning, might explain the lower levels of glucose metabolism in some regions. The areas of difference between the subjects with predominantly negative symptoms and those with predominantly positive symptoms, however, are not periventricular and therefore are less likely to be subject to partial volume effects related to ventricular enlargement. In addition, the areas with structural differences reported in schizophrenia (including in the superior temporal gyrus, medial temporal lobe, thalamus, basal ganglia, corpus callosum, and vermis of the cerebellum [24–26]) were not the areas that uniquely distinguished the negative symptom subjects from the positive symptom subjects in this study. The cerebellar/inferior occipital lobe border was the one area where unequivocal assignment of location was most problematic. No established structural changes in the inferior occipital lobe, the neocerebellar cortex, or deep cerebellar nuclei suggested partial volume confounds. The structural findings associated with the cerebellum have been confined to the vermis (26). The lack of signal localization in the ventricle or external to the brain substance also argued against partial volume effects underlying our findings.
Our PET study groups were relatively small; however, the subjects were matched between the three groups for gender, age, and handedness. The small size of the study groups may have explained why we did not find statistically significant differences between groups in performance on the Continuous Performance Task. In addition, it is often difficult to separate depression from negative symptoms and from the deficit syndrome (7). We excluded subjects who had evidence of depression from the negative symptom group. However, we did not assess whether subjects met the criteria for the deficit syndrome (7). Our findings may not extend to schizophrenic patients with deficit syndrome, concurrent depression, or a treatment-refractory illness. Further, we only studied right-handed male schizophrenic subjects. Therefore, we have not explored potential gender or handedness differences.
The FDG PET activation task used in this study, a degraded Continuous Performance Task, requires the subject to exercise visual perception, discrimination, perception of salience, attention, and cognition. No statistically significant differences in performance on the Continuous Performance Task were found between the positive and negative symptom groups, but, given the small size of the groups, some of the observed differences in glucose metabolic rate could reflect differences in Continuous Performance Task performance. However, this possibility was accounted for in the statistical parametric mapping analysis by covarying for Continuous Performance Task performance. Thus, differences in performance are unlikely to account for the observed differences in metabolic rate. Further, this study substantiates the value of a controlled cognitive task in revealing the circuitry underlying normal brain function and the pathophysiological mechanisms of neuropsychiatric disorders.
Significance of Findings
The negative symptoms of schizophrenia are difficult to treat, and the pathophysiological mechanisms underlying negative symptoms have not been adequately described. The present study was undertaken to examine the pattern of differences in glucose metabolic rate in subjects with predominantly negative symptoms, compared with subjects with predominantly positive symptoms.
The negative symptoms of schizophrenia include attentional impairments, impoverished emotional expression, social withdrawal, and the inability to develop good rapport. Patients with predominantly negative symptoms are characterized as having blunted affect and as apathetic and passive. It is expected that these symptoms would be reflected in abnormalities in several well-described neural systems. Attentional impairments may be reflected in changes in the medial prefrontal, anterior cingulate, and dorsolateral prefrontal regions. In this study, significant differences between the negative symptom subjects and those with predominantly positive symptoms, as well as the comparison group, were indeed observed in the medial prefrontal, dorsolateral prefrontal, and posterior cingulate cortices (but not the anterior cingulate cortices). These differences were most apparent in the subjects with predominantly negative symptoms, as reflected by their lower glucose metabolic rates in these areas.
The lower levels of emotional expression in patients with negative symptoms may be reflected in the strikingly lower glucose metabolic rates in the ventral prefrontal and orbital cortices. These differences were most apparent on the right side of the brain, the side that subserves the preponderance of emotional expression.
Language is affected more severely in subjects with predominantly negative symptoms than in those with predominantly positive symptoms. Not only are the amount and content of language impoverished in patients with negative symptoms, but the emotional expression and prosody are markedly poorer, contributing to the flat affect characteristic of the negative symptom patients. These symptoms are consistent with the dramatically lower metabolic levels in the sensory associative (Brodmann’s area 22), transcortical (Brodmann’s area 43), premotor (Brodmann’s areas 45 and 44), and motor (Brodmann’s areas 4 and 6) language cortices on the right side of the brain in the negative symptom subjects in this study. One language-associated cortex that had a higher level of metabolism in the negative symptom subjects is Brodmann’s area 39 on the right side, suggesting that at least part of the speech circuitry can be activated to a greater degree in negative symptom subjects.
We observed increases in the visual eye fields (Brodmann’s area 8) and the occipitotemporal convergence zone (Brodmann’s area 21 at the border of area 37) possibly overlapping V5 visual cortical area for both subjects with predominantly negative symptoms and those with predominantly positive symptoms. Perception of motion and visuospatial function are not well studied in schizophrenic patients. Stuve and colleagues (27) concluded that a dysfunction in V5 leading to a deficit in motion perception could explain the deficit in smooth pursuit gain observed in schizophrenia. Saccadic (28) and exploratory eye movement deficits and visual tracking abnormalities are well documented in schizophrenia and reported to be greater in patients with negative symptoms by some investigators (29, 30).
Patients with negative symptoms are especially subject to difficulties in correctly identifying and expressing the emotional content of both faces and scenes (31). Consistent with this symptom was the lower glucose metabolic rate in the ventral visual stream/inferotemporal/fusiform area in the right hemisphere that we observed in the subjects with predominantly negative symptoms. By using facial emotion recognition tests, Borod and colleagues (32) provided support that negative symptoms in schizophrenia are associated with right hemisphere dysfunction, a finding consistent with the present results.
The majority of theories about the etiology and treatment of schizophrenia implicate dopaminergic mechanisms. Subcortical hyperdopaminergic function in schizophrenia, with concomitant hypometabolism in the prefrontal cortex and striatum, has been a dominant theme, reflecting dysfunctional cortical-striatal-pallidal-thalamic-cortical loops (33). Our data suggest an even greater metabolic deficit in the prefrontal and temporal cortices in the subjects with predominantly negative symptoms, which is consistent with a greater cortical dopamine dysfunction in these subjects. The lack of subcortical differences between the negative symptom subjects and the positive symptom subjects is noteworthy.
The negative symptom subjects had a higher glucose metabolic rate in the cerebellar cortex bilaterally but a lower rate in the deep cerebellar nuclei. These cerebellar abnormalities are consistent with a higher level of neocerebellar inhibition of deep cerebellar nuclei, leading to a lower level of excitation of cerebellar-thalamic-frontal output. Thus, the lower metabolic rates bilaterally in the frontal lobes of subjects with predominantly negative symptoms are consistent with cerebellar output dysfunction or “attempts” to compensate for the primary frontal deficit.
Cognitive dysmetria, described by Andreasen and colleagues (34) as an impairment in synchrony of mental processes, has been ascribed to dysfunction in the cortical-thalamic-cerebellar-cortical circuit. This feedback loop permits a “constant checking and updating of input and output at the nanosecond level, and facilitates the rapid and smooth execution of complex motor acts;” it also contributes to “monitoring and coordinating the fluid execution of mental activity” (34). In general, subjects with predominantly negative symptoms have greater deficits in these motor and cognitive operations. Although most patients have both positive and negative symptoms cross-sectionally, our study groups were chosen on the basis of a predominance of symptoms in order to facilitate contrasts in symptoms, functions, and associated circuitry. It is possible that the higher cerebellar metabolic rates in the negative symptom subjects constituted compensation to overcome a relative failure of activation of the corticocortical, corticostriatal, and associated basal ganglia loop circuitry, especially in the frontal lobe. This interpretation is consistent with the positive correlation between cerebellar blood flow and number of negative symptoms found by Kim et al. (10). The negative symptom subjects appeared to have more abnormalities of these circuits than the positive symptom subjects. Further studies involving subjects with schizophrenia and schizoaffective disorder who manifest both prominent positive and negative symptoms are needed to validate and extend our findings.
![]() |
![]() |
Received Feb. 5, 2001; revision received Aug. 21, 2001; accepted Aug. 29, 2001. From the Department of Psychiatry and Human Behavior and the Department of Anatomy and Neurobiology, University of California, Irvine; and Novartis Pharmaceuticals Corporation, East Hanover, N.J. Address reprint requests to Dr. Potkin, Department of Psychiatry and Human Behavior, University of California, Irvine, Irvine Hall, Room 166, Irvine, CA 92697-3960; [email protected] (e-mail). Data analysis supported by grant RR-00827 from the National Center for Research Resources. PET scans were supported in part by Novartis Pharmaceuticals.
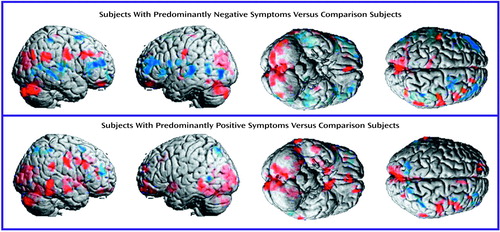
Figure 1. Surface Renderings of Mean Differences in Regional Brain Metabolic Activity Assessed by PET in Schizophrenic Subjects With Predominantly Negative Symptoms (N=7) and Predominantly Positive Symptoms (N=7), Compared With Matched Normal Subjects (N=7)a
aMean levels of metabolic activity (mg/100 g tissue per minute) are superimposed on averaged MRI images. Red indicates a higher level of metabolic activity and blue a lower level in the schizophrenic subjects, relative to the comparison group. Statistically significant (p<0.025) regional differences were determined by using a 35-voxel extent threshold to estimate the corrected p value for multiple comparisons.
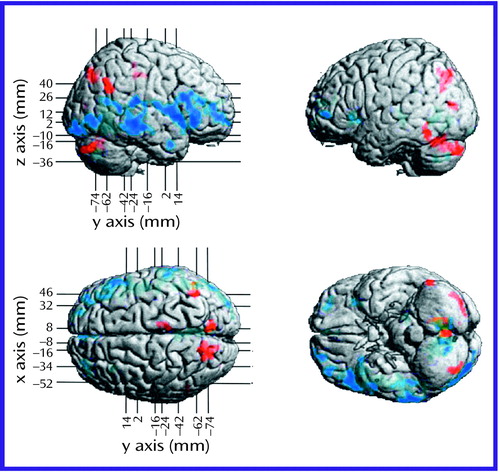
Figure 2. Surface Renderings of Mean Differences in Regional Brain Metabolic Activity Assessed by PET Between Schizophrenic Subjects With Predominantly Negative Symptoms (N=7) and Schizophrenic Subjects With Predominantly Positive Symptoms (N=7)a
aMean levels of metabolic activity (mg/100 g tissue per minute) are superimposed on averaged MRI images. Red indicates a higher level of metabolic activity and blue a lower level in the schizophrenic subjects with predominantly negative symptoms, relative to those with predominantly positive symptoms. Statistically significant (p<0.025) regional differences were determined by using a 35-voxel extent threshold to estimate the corrected p value for multiple comparisons. The x, y, and z coordinates correspond to Talairach space (20).
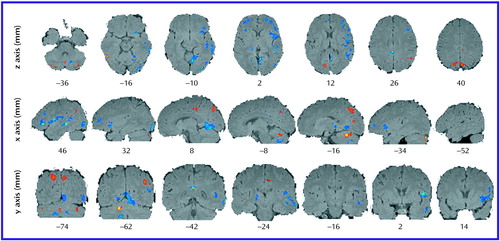
Figure 3. Axial, Sagittal, and Coronal Sections Showing Mean Differences in Regional Brain Metabolic Activity Assessed by PET Between Schizophrenic Subjects With Predominantly Negative Symptoms (N=7) and Schizophrenic Subjects With Predominantly Positive Symptoms (N=7)a
aTop row shows axial sections, middle row sagittal sections, and bottom row coronal sections. Mean levels of metabolic activity (mg/100 g tissue per minute) are superimposed on averaged MRI images. Red indicates a higher level of metabolic activity and blue a lower level in the schizophrenic subjects with predominantly negative symptoms, relative to those with predominantly positive symptoms. Statistically significant (p<0.025) regional differences determined by using a 35-voxel extent threshold to estimate the corrected p value for multiple comparisons. The x, y, and z coordinates correspond to Talairach space (20).
1. Ananth J, Djenderdjian A, Shamasunder P, Costa J, Herrera J, Sramek J: Negative symptoms: psychopathological models. J Psychiatry Neurosci 1991; 16:12-18Medline, Google Scholar
2. Andreasen NC, Olsen S: Negative v positive schizophrenia: definition and validation. Arch Gen Psychiatry 1982; 39:789-794Crossref, Medline, Google Scholar
3. Tamminga CA, Buchanan RW, Gold JM: The role of negative symptoms and cognitive dysfunction in schizophrenia outcome. Int Clin Psychopharmacol 1998; 13(suppl 3):S21-S26Google Scholar
4. Crow TJ: Brain changes and negative symptoms in schizophrenia. Psychopathology 1995; 28:18-21Crossref, Medline, Google Scholar
5. Mueser KT, Douglas MS, Bellack AS, Morrison RL: Assessment of enduring deficit and negative symptom subtypes in schizophrenia. Schizophr Bull 1991; 17:565-582Crossref, Medline, Google Scholar
6. McGlashan TH, Fenton WS: The positive-negative distinction in schizophrenia: review of natural history validators. Arch Gen Psychiatry 1992; 49:63-72Crossref, Medline, Google Scholar
7. Buchanan RW, Breier A, Kirkpatrick B, Ball P, Carpenter WT Jr: Positive and negative symptom response to clozapine in schizophrenic patients with and without the deficit syndrome. Am J Psychiatry 1998; 155:751-760Link, Google Scholar
8. Wu JC, Amen D, Bracha HS: Neuroimaging in clinical practice, in Kaplan and Sadock’s Comprehensive Textbook of Psychiatry, 7th ed. Edited by Sadock BJ, Sadock VA. New York, Williams & Wilkins, 2000, pp 373-385Google Scholar
9. Kishimoto H, Yamada K, Iseki E, Kosaka K, Okoshi T: Brain imaging of affective disorders and schizophrenia. Psychiatry Clin Neurosci 1998; 52(suppl):S212-S214Google Scholar
10. Kim J-J, Mohamed S, Andreasen NC, O’Leary DS, Watkins GL, Boles Ponto LL, Hichwa RD: Regional neural dysfunctions in chronic schizophrenia studied with positron emission tomography. Am J Psychiatry 2000; 157:542-548Link, Google Scholar
11. Lynch MR: Schizophrenia and the D1 receptor: focus on negative symptoms. Prog Neuropsychopharmacol Biol Psychiatry 1992; 16:797-832Crossref, Medline, Google Scholar
12. Sabri O, Erkwoh R, Schreckenberger M, Cremerius U, Schulz G, Dickmann C, Kaiser HJ, Steinmeyer EM, Sass H, Buell U: Regional cerebral blood flow and negative/positive symptoms in 24 drug-naive schizophrenics. J Nucl Med 1997; 38:181-188Medline, Google Scholar
13. Kay SR, Fiszbein A, Opler LA: The Positive and Negative Syndrome Scale (PANSS) for schizophrenia. Schizophr Bull 1987; 13:261-276Crossref, Medline, Google Scholar
14. Molina Rodriguez V, Montz Andree R, Perez Castejon MJ, Gutierrez Labrador R, Ferre Navarrete F, Carreas Delgado JL, Rubia Vila FJ: Cerebral perfusion correlates of negative symptomatology and parkinsonism in a sample of treatment-refractory schizophrenics: an exploratory 99mTc-HMPAO SPET study. Schizophr Res 1997; 25:11-20Crossref, Medline, Google Scholar
15. Schroder J, Buchsbaum MS, Siegel BV, Geider FJ, Lohr J, Tang C, Wu J, Potkin SG: Cerebral metabolic activity correlates of subsyndromes in chronic schizophrenia. Schizophr Res 1996; 19:41-53Crossref, Medline, Google Scholar
16. Kane J, Honigfeld G, Singer J, Meltzer H: Clozapine for the treatment-resistant schizophrenic. Arch Gen Psychiatry 1988; 45:789-796Crossref, Medline, Google Scholar
17. Nuechterlein KH, Parasuraman R, Jiang Q: Visual sustained attention: image degradation produces rapid sensitivity decrement over time. Science 1983; 220:327-329Crossref, Medline, Google Scholar
18. Phelps ME, Huang SC, Hoffman EJ, Selin CJ, Sokoloff L, Kuhl DE: Tomographic measurement of local cerebral glucose metabolic rate in humans with [18F]2-fluoro-2-deoxy-d-glucose: validation of method. Ann Neurol 1979; 6:371-388Crossref, Medline, Google Scholar
19. Friston KJ, Holmes AP, Worsley KJ, Poline JP, Frith CD, Frackowiak RSJ: Statistical parametric maps in functional imaging: a general linear approach. Hum Brain Mapp 1995; 2:189-210Crossref, Google Scholar
20. Talairach J, Tournoux P: Co-Planar Stereotaxic Atlas of the Human Brain: Three-Dimensional Proportional System. New York, Thieme Medical, 1988Google Scholar
21. Poline JB, Worsley KJ, Holmes AP, Frackowiak RSJ, Friston KJ: Estimating smoothness in statistical parametric maps: variability of p values. J Comput Assist Tomogr 1995; 19:788-796Crossref, Medline, Google Scholar
22. Friston KJ, Worsley KJ, Frackowiak RSJ, Mazziotta JC, Evans AC: Assessing the significance of focal activation using their spatial extent. Hum Brain Mapp 1994; 1:214-220Google Scholar
23. Lindenmayer JP, Bernstein-Hyman R, Grochowski S: A new five factor model of schizophrenia. Psychiatr Q 1994; 65:299-322Crossref, Medline, Google Scholar
24. Bunney BG, Potkin SG, Bunney WE Jr: New morphological and neuropathological findings in schizophrenia: a neurodevelopmental perspective. Clin Neurosci 1995; 3:81-88Medline, Google Scholar
25. Henn FA, Braus DF: Structural neuroimaging in schizophrenia: an integrative view of neuromorphology. Eur Arch Psychiatry Clin Neurosci 1999; 249(suppl 4):48-56Google Scholar
26. Nopoulos PC, Ceilley JW, Gailis EA, Andreasen NC: An MRI study of cerebellar vermis morphology in patients with schizophrenia: evidence in support of the cognitive dysmetria concept. Biol Psychiatry 1999; 46:703-711Crossref, Medline, Google Scholar
27. Stuve TA, Friedman L, Jesberger JA, Gilmore GC, Strauss ME, Meltzer HY: The relationship between smooth pursuit performance, motion perception and sustained visual attention in patients with schizophrenia and normal controls. Psychol Med 1997; 27:143-152Crossref, Medline, Google Scholar
28. Abel LA, Levin S, Holzman PS: Abnormalities of smooth pursuit and saccadic control in schizophrenic and affective disorders. Vision Res 1992; 32:1009-1014Crossref, Medline, Google Scholar
29. Kojima T, Matsushima E, Ando K, Ando H, Sakurada M, Ohta K, Moriya H, Shimazono Y: Exploratory eye movements and neuropsychological tests in schizophrenic patients. Schizophr Bull 1992; 18:85-94Crossref, Medline, Google Scholar
30. Roitman SE, Keefe RS, Harvey PD, Siever LJ, Mohs RC: Attentional and eye tracking deficits correlate with negative symptoms in schizophrenia. Schizophr Res 1997; 26:139-146Crossref, Medline, Google Scholar
31. Bryson G, Bell M, Kaplan E, Greig T, Lysaker P: Affect recognition in deficit syndrome schizophrenia. Psychiatry Res 1998; 77:113-120Crossref, Medline, Google Scholar
32. Borod JC, Martin CC, Alpert M, Brozgold A, Welkowitz J: Perception of facial emotion in schizophrenic and right brain-damaged patients. J Nerv Ment Dis 1993; 181:494-502Crossref, Medline, Google Scholar
33. Andreasen NC: A unitary model of schizophrenia: Bleuler’s “fragmented phrene” as schizencephaly. Arch Gen Psychiatry 1999; 56:781-787Crossref, Medline, Google Scholar
34. Andreasen NC, Nopoulos P, O’Leary DS, Miller DD, Wassink T, Flaum M: Defining the phenotype of schizophrenia: cognitive dysmetria and its neural mechanisms. Biol Psychiatry 1999; 46:908-920Crossref, Medline, Google Scholar