Abnormal Patterns of Regional Cerebral Blood Flow in Schizophrenia With Primary Negative Symptoms During an Effortful Auditory Recognition Task
Abstract
OBJECTIVE: Using functional brain imaging, the authors sought to replicate their earlier finding of low metabolism in the middle frontal and inferior parietal cortices of schizophrenic patients with primary negative symptoms. METHOD: According to the presence or absence of enduring negative symptoms, patients with schizophrenia were classified as having deficit or nondeficit schizophrenia, respectively. Twelve normal volunteers and 18 drug-free schizophrenic volunteers (deficit, N=8; nondeficit, N=10) were trained in a tone discrimination task. They were trained to perform with 70%–80% accuracy and were then scanned with positron emission tomography with [15O]H2O during three conditions: rest, sensory-motor control task, and decision task. RESULTS: Levels of performance of the auditory recognition task were similar in the three groups. An initial hypothesis-driven analysis revealed that across tasks the deficit group failed to show significant activation in the middle frontal cortex. This was in contrast to both the normal volunteers and nondeficit patients. When the patient groups were contrasted, the deficit patients showed significantly less activation in the middle frontal cortex bilaterally during the control task and in the right middle frontal cortex and inferior parietal cortex during the decision task. An exploratory analysis contrasting deficit and nondeficit patients across conditions did not reveal further differences between groups. CONCLUSIONS: This study replicated the finding of low activation in the middle frontal cortex and inferior parietal cortex in deficit schizophrenia. This deficit was observed without performance confound and may provide a marker of primary negative symptoms and a target for new therapies.
Schizophrenia is a lifelong psychotic illness that begins in the early adult years. It is characterized by bizarre mental, cognitive, and affective symptoms, of both positive and negative types (1). While some aspects of its biology are known (2), the critical components of its pathophysiology remain to be discovered. Its course includes acute psychotic episodes and quiescent periods with residual symptoms, in highly variable combinations (3–8). Since 1974 it has been recognized that psychosis, negative symptoms, and social functioning are relatively independent domains (9). In 1982 Andreasen and Olson (10) separated positive psychosis into reality distortion and disorganization, and many studies have confirmed the three-factor symptom clusters (1, 11). Whether these symptom dimensions represent distinct illnesses with their own etiologies and pathophysiologies or are merely multiple manifestations of a single illness has been a topic of repeated discussion (9, 12–16).
Primary negative symptoms, often called deficit symptoms, are manifestations of schizophrenia. Studying them to answer questions of etiology and mechanism has often been hampered by the almost ubiquitous confound of secondary negative symptoms, which influence deficit ratings. Secondary negative symptoms may occur because of depression, paranoia, medication side effects, and other conditions. We have proposed that decisive studies on the biology of deficit symptoms be based on contrasting deficit schizophrenia with nondeficit schizophrenia (14). Already, distinguishing biologic characteristics of patients with primary deficit symptoms have been defined on the basis of genetic (17, 18), risk factor (19), electrophysiologic (20, 21), and biochemical (22, 23) studies. In a preliminary study (24), we showed that patients with deficit schizophrenia had lower levels of neuronal activity at rest in the middle frontal cortex, inferior parietal cortex, and thalamus than did patients with nondeficit schizophrenia.
The development of modern techniques for human brain imaging has powerfully advanced not only our understanding of normal brain function, but also our concepts of human brain diseases. Brains of individuals with a defined CNS illness or subtype can be directly studied in vivo for CNS structural, neurochemical, or functional abnormalities characteristic of the brain disease. Functional human brain imaging is ideally suited to studying subgroup characteristics in schizophrenia, because the usual illness confounds among the patient volunteers are similar in the subgroups. These confounds include long-term administration of antipsychotic and anticholinergic medication, environmental deprivation, lifelong exposure to trauma and institutionalization, and other factors. Already, imaging techniques have advanced our basic knowledge of schizophrenia (25–35). In particular, several studies have already shown that positive and negative symptom dimensions are associated with different patterns of regional activation. Regional analyses of the correlations between primary negative symptoms and regional cerebral blood flow (rCBF) have implicated the middle frontal regions and inferior frontal cortex (27, 36), as have dichotomies analyses contrasting subjects with and without primary negative symptoms (24, 37).
Therefore, we hypothesized that low rCBF in the middle frontal and inferior parietal cortices bilaterally would occur with performance of an effortful task in deficit schizophrenia. In order to test this hypothesis, we recruited schizophrenic volunteers with and without deficit symptoms but similar in other symptom domains. The two groups were contrasted, without centrally active medication, with matched healthy volunteers free from all CNS disease. Using rCBF measures, we evaluated all volunteers with [15O]H2O and positron emission tomography (PET), performed during an effortful auditory discrimination task in which performance was matched across groups. We previously reported (38) that when the total group of patients was compared to the normal volunteers, the patients with schizophrenia had less activity in the anterior cingulate.
Method
Volunteers
Eighteen medically healthy schizophrenic persons were recruited from the Residential Research Unit of the Maryland Psychiatric Research Center in Baltimore. Each underwent a Structured Clinical Interview for DSM-III-R (SCID) at hospital admission. Two research psychiatrists (A.C.L., M.A.W.) reached a consensus diagnosis of schizophrenia (according to DSM-III-R criteria) on the basis of the clinical interview and all other sources of data. The diagnosis of deficit syndrome (primary negative symptoms) was made by using the Schedule for the Deficit Syndrome (39). This instrument allows the categorization of schizophrenic patients into those with and those without deficit symptoms on the basis of a semistructured interview. Diagnostic categorization is achieved through the use of operational criteria taking into account six negative symptoms (restricted affect, diminished emotional range, poverty of speech, curbing of interests, diminished sense of purpose, and diminished social drive), their stability over time, and whether the symptoms are primary or secondary (for instance, not secondary to factors such as neuroleptic akinesia, paranoid withdrawal, depressive anhedonia, withdrawal during compelling psychotic experience). This instrument has demonstrated good reliability in the schizophrenic population (kappa=0.73).
The total schizophrenia volunteer group included 15 male and three female persons with a mean age of 31.6 years (SD=7.7). Seventeen of the 18 schizophrenic volunteers were right-handed.
The schizophrenic volunteers, all inpatients, were treated with a fixed dose (0.3 mg/kg) of haloperidol and no other neurally active medications for at least 4 weeks before the study. A drug-free period followed haloperidol treatment. All other aspects of inpatient treatment were continued. Symptoms of schizophrenia were assessed repeatedly during the haloperidol baseline and during the subsequent drug-free period by using the Brief Psychiatric Rating Scale (BPRS) (40). Symptoms were rated by two trained raters, and a consensus rating was obtained. Clinical monitoring was continuous, and intervention was available when there was a clinical judgment of significant behavioral change requiring treatment.
Twelve healthy volunteers were recruited from the community through newspaper advertising and were screened by telephone for generally good medical health and the lack of major psychiatric illness. If suitable, they were further screened in face-to-face interviews using the Structured Clinical Interview for DSM-III-R—Non-Patient Edition and the Structured Interview for DSM-III-R Personality Disorders (41) to rule out current or past history of axis I or axis II disorders. Family history of schizophrenia in any first-degree relative of the prospective healthy volunteer was ruled out by clinical interview. Six comparison subjects were male, and six were female. All were right-handed, and their mean age was 28.4 years (SD=4.6).
Informed Consent
The normal and schizophrenic volunteers were fully informed about the nature of the protocol, and afterward each gave informed consent. Only patients who were competent and clinically judged to be capable of understanding and appreciating the risks involved in this study were selected to participate. The nature of the protocol was presented on several occasions by separate people, including both the principal investigator (A.C.L.) and noninvestigator clinicians. Family members or care givers were involved in the information process when available.
Tasks
The decision condition was a forced-choice auditory discrimination task. The subject was instructed to immediately press a button held in the right hand when he or she recognized a high-frequency reference tone (1500 Hz) and to press the button in his or her left hand with a lower-frequency tone (800–1492 Hz). Equal numbers of high and low tones, each lasting 100 msec, were randomly presented in each stimulus condition; there were 100 trials in each training set and 60 trials in each imaging set. The interval between trials (onset of first tone to onset of subsequent tone) was 2 seconds. Failure to respond was scored as an error. Response time was calculated as the time from the onset of the stimulus to the button press.
During the active control task (sensory-motor task), the subject heard the same sets of tones that were presented during the decision task. However, he or she was instructed to merely press the right- and left-hand buttons alternately after each tone.
During the rest condition, the subject was instructed to lie quietly. Eyes were open, and earphones were in place during all scans.
Training
All subjects began training on the decision task 2–5 weeks before the scan sessions. Training occurred in a quiet, isolated room. The purposes of the training were to eliminate stimulus or task novelty, determine what frequency difference reliably provided 70%–80% accuracy in performance by the subject, and reduce performance variability within and between volunteer groups. Each volunteer participated in three to five sessions, and there were six training blocks per session. The patients began training during the haloperidol treatment phase and continued throughout the medication withdrawal period.
The tones presented during training were systematically modified by decreasing the frequency difference between the reference and test tones, as the subjects became increasingly accurate in recognizing the two types of stimuli. In the first training session the frequency differences between the high and low tones ranged from 700 to 20 Hz. If a subject was unable to perform with at least 80% accuracy at the largest frequency difference (700 Hz) after three attempts at training, the subject was dropped from further study.
PET Imaging
PET scans were obtained by using the General Electric (Milwaukee) 4096+ system, which produces 15 brain image slices at an intrinsic resolution of 6.1 mm in each dimension. The bolus [15O]H2O method (42) was used without arterial blood sampling. Approximately 62 mCi of [15O]H2O were administered on each occasion. Ten to twelve minutes elapsed between scans. The relative CBF distribution was measured in each of three conditions—1) rest, 2) sensory-motor control task, and 3) decision task—with four scans per condition (12 scans per subject). The order of the scans was fixed for all subjects: rest, control task, decision task, consecutively repeated four times. In the rest condition the subject was inactive, with eyes open and ears unplugged; tone stimuli and motor activity were absent, but the earphones were in place. The sensory-motor control task consisted of exposure to the same tones presented during the decision task with alternation of right and left button presses in synchrony with the tones. During the decision task the subject was asked to press the right button when a high-frequency tone was recognized and the left button when a low-frequency tone was detected. Within a given scan the frequencies of the two tones were held constant. The frequencies were chosen on the basis of the training accuracy for that individual. Sixty trials were delivered during each scan; the tones began 15 seconds before tracer delivery, and scan acquisition began 20 seconds after dose delivery. PET data were acquired for 60 seconds.
Image Analysis
The quantitative PET blood flow images were analyzed with modified statistical parametric mapping routines (43). The scans from each subject were realigned by using the first one as a reference. The alignment procedure was modified to use 10 iterations to optimize the spatial correlation between data sets; the default procedure uses three iterations. After realignment, all images were transformed into a standard anatomical space (44). Before generation of the statistical parametric map, the data were smoothed by using a 12-mm Gaussian kernel. Only clusters of connected voxels (face or edge) above a threshold (z=2.33, p=0.01, one-tailed, uncorrected for multiple comparisons) were tested for significance by means of spatial extent statistical theory, and significance was set at an alpha level of <0.05 after correction for multiple comparisons (43). The full width at half maximum of the average noise subtraction image was used to determine the number of resels (45) available for analysis. Task-related changes in rCBF between the control task and rest and between the control task and decision task were evaluated for each volunteer group; increases and decreases were assessed. Patients with deficit schizophrenia were compared to patients with nondeficit schizophrenia on the same contrasts. The spatial/anatomical distribution and extent of each cluster was mapped onto matching magnetic resonance images aligned and warped to “fit” the statistical data. For hypothesized effects, an uncorrected significance level of alpha=0.05 was used to determine significant areas of activation. For all other analyses, a multiple comparison correction was used. In regions of hypothesized differences, the maxima of significant clusters in the statistical parametric mapping analysis were grown to a 3×3×3-pixel sample volume; adjusted rCBF values were obtained in these samples for each task condition.
By means of Pearson’s product moment correlations, days of medication withdrawal in all patients were correlated with rCBF in regions showing differences between patients with deficit and nondeficit schizophrenia. Similarly, negative symptoms as measured on the BPRS withdrawal subscale were correlated with rCBF in these regions for the patients with deficit schizophrenia only.
Behavioral outcome measures were extracted from all trials generated in the PET scan sessions during the control and decision tasks. These measures include mean accuracy, mean response time, and mean frequency differences between high and low tones for accurate trials. Differences among the three groups on those measures were each tested by using a Kruskal-Wallis test. Significant results were further tested by performing a Wilcoxon rank sum test on all pairs.
Results
Task Performance by Each Group
Of the 18 schizophrenic volunteers in this study, eight met the criteria for deficit schizophrenia while 10 patients did not. The two patient groups, deficit and nondeficit schizophrenia, were similar on several key demographic elements, including age, length of illness, expression of positive symptoms (score on BPRS psychosis subscale), and parental socioeconomic status (Table 1). The patients with deficit schizophrenia had a significantly higher score on the BPRS withdrawal subscale.
The mean duration of antipsychotic medication treatment was 8.3 years (SD=7.0) for the deficit group and 5.5 years (SD=5.9) for the nondeficit group; the difference was not significant. There were no differences in either smoker status (yes/no: deficit group=5/3, nondeficit group=7/2) or history of drug or alcohol problems (yes/no: deficit group=2/6, nondeficit group=4/5) between the patient groups. Before scanning, the volunteers with deficit schizophrenia had been withdrawn from antipsychotic medication for a mean of 22.6 days (SD=14.1), and those with nondeficit schizophrenia had been without medication for 21.9 days (SD=13.3).
Training exposure was not significantly different among the three groups of volunteers. The mean number of blocks of tone recognition trials needed to achieve 70%–80% accuracy was 28.3 (SD=6.1) for the deficit patient group, 30.6 (SD=16.0) for the nondeficit patient group, and 29.4 (SD=12.0) for the healthy comparison group.
Response times and levels of accuracy in performance of the auditory recognition task during rCBF imaging were similar in the deficit, nondeficit, and healthy volunteers (Table 2). However, there was a significant difference among the three groups in the frequency difference between the high and low tones (Kruskal-Wallis χ2=12.27, df=2, p<0.003). Further analysis of the overall frequency difference revealed a significant difference between the normal and nondeficit groups and between the normal and deficit groups (Table 2) but no difference between the two groups of patients with schizophrenia.
Hypothesis-Driven Analyses: Middle Frontal and Inferior Parietal Cortices
The a priori hypotheses that patients with deficit schizophrenia would have low rCBF in the middle frontal and inferior parietal cortices bilaterally allowed an initial hypothesis-testing analysis of the data. Significant clusters on the statistical parametric mapping analysis (within-group contrasts and between-patients contrasts) that fell within these anatomic areas were analyzed by using a priori criteria.
Within-group contrasts across tasks
All significant task-induced rCBF changes that occurred in the regions hypothesized to show differences are presented in Table 3 for all three groups. For comparison, values are shown for the same regions in groups where no significant effect was seen.
For the normal volunteers, the sensory-motor control task produced no significant activations, relative to the resting condition, in the left or right middle frontal or inferior parietal cortex. The nondeficit patient group showed a significant activation in the right and left middle frontal region. The deficit group showed no significant activations in these cortical regions during this task. No significant decreases during the control task were observed in any group (Table 3).
Compared to the control task, the decision task produced significantly greater activation in the right middle frontal cortex (x, y, z: 34, 38, 12) of the normal volunteer group. Another middle frontal region (x, y, z: 42, 30, 32) was significantly activated in the nondeficit schizophrenia group. However, in the deficit group these regions showed no significant activation. No significant reductions were seen (Table 3).
Deficit versus nondeficit patients
During performance of the sensory-motor control task, the nondeficit patient group showed greater rCBF activation, relative to the resting condition, in the left and right middle frontal cortex than did the deficit group. Table 3 displays the values for the normal volunteers at the same region for comparison.
During the decision task, the patients with nondeficit schizophrenia showed significantly more activation, relative to the control task, in two right middle frontal clusters and one inferior parietal region than did the patients in the deficit group (Table 3).
There was no significant correlation between rCBF activation in these three regions and negative symptoms (measured on the BPRS withdrawal scale) in the deficit group.
Individual rCBF changes in the right middle frontal area (x, y, z: 44, 32, 36) for patients in the three groups during the decision task, relative to the control task, are contrasted in Figure 1. These individual values show that whereas nine of the 10 nondeficit patients demonstrated activation in the middle frontal cortex, seven of the eight deficit patients showed reductions in rCBF.
Exploratory Analysis: Group rCBF Patterns Across Tasks
The usual statistical parametric mapping analysis using hierarchical task subtraction was carried out for each of the three groups. Clusters representing common task-associated CNS regions were identified without a priori hypotheses.
Normal volunteers
The normal rCBF contrast patterns of the healthy volunteers during the control and decision tasks are presented in detail elsewhere (46) and summarized in Table 4. Compared to the resting condition, during the active control condition the auditory cortex of the normal group was activated prominently and bilaterally, along with the inferior frontal cortex bilaterally, the left motor cortex, and the cerebellum. The precuneus, medial frontal cortex, and the posterior middle temporal cortex demonstrated reduced rCBF during the active control condition (Table 4, Figure 2).
The decision condition was associated with significant rCBF activations, compared to the control task, in the right middle and right inferior frontal cortices, the anterior cingulate, the insula, and the cerebellum. The left posterior middle temporal cortex showed a flow decrement (Table 4, Figure 3).
Patients with nondeficit schizophrenia
Compared to the resting condition, the control task produced activations in the auditory cortex, the premotor cortex, the middle frontal cortex, and the supplementary motor cortex, all bilaterally, in the group with nondeficit schizophrenia (Table 5, Figure 2). rCBF was reduced in the right lingual gyrus and the precuneus cortex bilaterally.
For the patients with nondeficit schizophrenia, activation was significantly greater during the decision task than during the control task in the right middle frontal cortical region (Table 5, Figure 3). No regions of rCBF suppression could be demonstrated.
Patients with deficit schizophrenia
During the control task, the right postcentral gyrus of the deficit group was more activated than during rest, but the difference fell short of significance (Table 6, Figure 2). The right superior frontal gyrus showed a significant reduction in rCBF during the task (Table 6).
No regions were significantly activated in the decision task, relative to the control task, in the deficit patient group (Figure 3).
Exploratory Analysis: Deficit Versus Nondeficit Patients
Control task contrasted with rest
In the regions hypothesized to show differences between the two schizophrenia groups (Table 3), both the left (x, y, z: –42, 36, 32) and right (x, y, z: 38, 34, 14) middle frontal cortex were significantly more activated during the control task in the patients with nondeficit schizophrenia than in the deficit group (Figure 4, left panel). No other regions showed differences between the patient groups (either increase or decrease).
Decision task contrasted with control task
In the identified regions (Table 3), the patients with nondeficit schizophrenia had significantly more activation than the deficit group in the right middle frontal cortex (two clusters) (x, y, z: 44, 32, 36 and x, y, z: 38, 6, 34) and the right inferior parietal cortex (x, y, z: 56, –38, 26) (Figure 4, right panel). No other regions showed differences between the patient groups.
Discussion
These data show that medication-free schizophrenic individuals who differ from each other primarily on the basis of the presence or absence of enduring negative symptoms differ profoundly in the pattern of rCBF abnormalities that is generated with effortful task performance, even when performance of the task is standardized. We have previously reported (46) that the normal individuals scanned for this study experience activation of the auditory and motor cortex with the sensory-motor control condition and activation of the anterior cingulate and right frontal cortex with the effortful decision task. Moreover, the task-activated rCBF pattern of the entire schizophrenia group (N=18) differed from the normal pattern only in the anterior cingulate cortex, where rCBF was lower in the patients with schizophrenia (38). Here we report that the deficit schizophrenia group showed low rCBF activation in the left and right middle frontal cortex during the control task and in the right middle frontal and right inferior parietal area during the effortful task. These flow differences occurred despite matched behavioral performance across the three groups. Moreover, these data may explain previous reports of “hypofrontality” in schizophrenia studies with skewed subject subgroups. When the two patient groups in this study were compared to normal volunteers, both showed significant hypoactivation of the anterior cingulate during the effortful task. Neither of the groups showed hypofrontality when contrasted to the normal volunteers.
In this study and in others (9, 12, 14–16, 27), a pathologic dimension within the schizophrenia syndrome was isolated in an attempt to establish homogenous subgroups with distinguishing physiologic, pharmacologic, and anatomic characteristics. Functional imaging analysis provided a critical tool in this comparison of these two populations of psychiatric patients, who were matched on key illness features (positive symptoms and illness characteristics) but were divergent on a single disease characteristic (primary negative symptoms). In this study patients with deficit and nondeficit schizophrenia were prospectively identified by using operational criteria for the presence or the absence of enduring negative symptoms (39). All patients were medication free and trained to perform an auditory discrimination task at similar levels of performance, matched to that of healthy volunteers. Under these conditions, the lower rCBF in deficit schizophrenia in the middle frontal cortex and in the right inferior parietal cortex represents a regional functional deficit without performance confound. Although the patients were medication free for a mean period of 3 weeks, we cannot be certain that their brain function had returned to premedication status at the time of scanning. Postmortem studies (47) have shown that antipsychotic medications are eliminated slowly from brain tissue. Further preclinical studies (48) reveal slow return of receptor function to normal after exposure to antipsychotic medications. These are important considerations. However, because we did not find any correlations between the number of days of medication withdrawal and rCBF in regions showing group differences, we believe that residual medication effects did not significantly alter the results. The findings presented here replicate and extend the results of our previous PET/fluorodeoxyglucose study (24), in which patients with primary negative symptom had low rates of glucose metabolism at rest in the middle frontal and inferior parietal cortices bilaterally.
These results are limited by several considerations. The number of patients was low, particularly in the group with deficit schizophrenia. So, despite the fact that clusters failed to develop in the deficit group as in the normal subjects, even at nonsignificant levels, low subject number and possibly greater task-associated regional variability may explain the failure to find an activation in the subjects with deficit schizophrenia. Moreover, although the subject groups were matched on performance, the frequency required to standardize performance at 70%–80% accuracy was highly variable across groups. These limitations introduce added variability into the results. However, these effects appear insufficient to account entirely for these results.
Low frontal cortex metabolism in deficit, but not nondeficit, schizophrenia has been observed in several other functional imaging laboratories(27, 36, 37, 49, 50); low CBF in the inferior parietal cortex has been reported as well (37). These regional associations between brain metabolism and negative symptoms, or the deficit state, in schizophrenia have characteristically been based on correlational analyses (49, 51, 52) or factor analyses (27, 50, 53, 54). Few studies have used a categorical approach (i.e., dichotomization according to a disease characteristic) to contrast flow characteristics across symptom domains (24, 37). Moreover, because antipsychotic medications already reduce regional metabolism in the frontal cortex (55), concurrent treatment with medications becomes a critical confound in studies conducted without withdrawal.
Heterogeneity of cerebral regions that behave abnormally within the diagnostic category of schizophrenia raises the question of whether schizophrenia, as we diagnose it, is a single disease with multiple manifestations or multiple diseases all within a distinct pathophysiology. While these data can illustrate the dilemma, they cannot answer the question. However, these data demonstrate the problem associated with grouping individual results into mean values across all schizophrenia subjects and the possibility of obscuring significant underlying pathology. The dichotomy of primary negative versus nonnegative symptoms of schizophrenia appears to have reduced the heterogeneity in this experiment, and other phenomenologic dichotomies may accomplish the same purpose.
These data provide additional evidence associating human behaviors with lower than normal functioning of the middle frontal cortex and inferior parietal cortex. We can speculate that deficit or primary negative symptoms, like impaired aspects of social affiliation, impaired interpersonal communication, and minimal goal-directed cognitive performance, are generated by functional failures of neuronal populations in these regions. The normal functions of the middle frontal cortex have been inferred from lesion studies and human in vivo functional imaging experiments. Overall, lesion studies suggest the involvement of this area in personality expression and certain aspects of cognition (56, 57). The specific cognitive processes mediated by the middle frontal cortex depend on region and laterality (58). In vivo functional imaging studies implicate this area (Brodmann’s area 9/46) in working memory (verbal on left and nonverbal on right), episodic retrieval, semantic processing, spatial processing (right hemisphere), and attention (59). Multiple type of experiments with humans and primates (60–63) implicate the inferior parietal cortex in visuomotor integration and spatial attention processing. In imaging studies (64–67), the inferior parietal cortex is activated by tasks requiring selective visual attention and spatial processing. Lesions of the inferior parietal cortex in humans are associated with the inability to look on command at a visual target, with optic ataxia, and with oculomotor deficits. Some but not all of the functions associated with these regions are deficient in schizophrenia. Attention, working memory, and executive function are the deficits most often mentioned (68–70). Buchanan and colleagues (11, 71) have reported that subjects with deficit symptoms have worse neurocognitive performance than nondeficit patients. Patients with deficit symptoms may not be able to integrate internal and external representations or sensory cues in an adaptive, rapid manner. Parietal lobe dysfunction may be responsible for this integrative failure. A secondary consequence of parietal lobe failure may be withdrawal from social and environmental novelty.
These data demonstrate functional heterogeneity within the overall diagnosis of schizophrenia, an observation that has implications for diagnosis and clinical management of the illness. This heterogeneity has been reduced by the deficit/nondeficit dichotomization. Whether both subgroups have the same underlying pathology, only differently distributed, or entirely different pathologies is still a matter of conjecture. In either case, these kinds of data can advance the field by suggesting a focus for studies of postmortem tissue pathology. Prospective analyses of postmortem tissue from these hypothesized regions of the brains of people with schizophrenia have already been productive (72, 73). Although it is premature to suggest the diagnostic use of rCBF patterns, one could propose this rCBF abnormality associated with the deficit state as a surrogate marker of primary negative symptoms in the illness and as a target rCBF pattern for development of new therapies for negative symptoms. Currently there is no treatment for deficit symptoms in schizophrenia, and clinical outcome for this patient group is poor (74, 75). For these reasons, the needs for advances in the treatment of this schizophrenia cohort and technical tools to expedite discovery are particularly pressing.
![]() |
![]() |
![]() |
![]() |
![]() |
![]() |
Received Oct. 20, 2000; revision received May 1, 2001; accepted May 10, 2001. From the Maryland Psychiatric Research Center, University of Maryland School of Medicine. Address reprint requests to Dr. Lahti, Maryland Psychiatric Research Center, University of Maryland School of Medicine, P.O. Box 21247, Baltimore, MD 21228; [email protected] (e-mail). Supported by the William K. Warren Medical Research Institute and NIMH grant MH-40279. The authors thank the schizophrenic and normal volunteers who took part in this project, the staff of the Residential Research Unit of the Maryland Psychiatric Research Center, Ning Ma and Kristin Frey for statistical expertise, and Kristin Ricasa for administrative assistance.
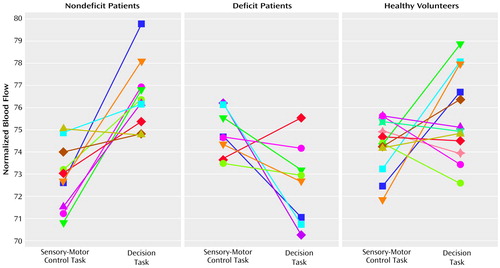
Figure 1. Normalized Cerebral Blood Flowa in the Right Middle Frontal Cortexb During a Sensory-Motor Control Task and a Decision Task Performed by Healthy Volunteers and Schizophrenic Patients With and Without Primary Negative Symptoms (Deficit Schizophrenia)c
aNormalized to an arbitrary flow value.
bTalairach-Tournoux coordinates: x=44, y=32, z=36.
cNine of the 10 patients with nondeficit schizophrenia had activation in this region, whereas seven of the eight patients with deficit schizophrenia had a reduction in blood flow.
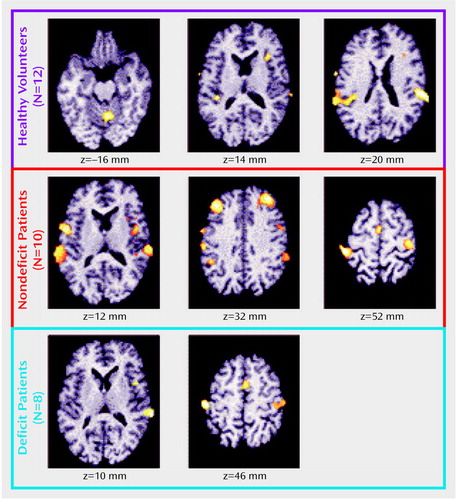
Figure 2. Changes in Cerebral Blood Flow Between Rest and a Sensory-Motor Control Task in Healthy Volunteers and Schizophrenic Patients With and Without Primary Negative Symptoms (Deficit Schizophrenia)a
aIn the healthy volunteers, the control task activated limited regions of the cerebellum and the inferior frontal, auditory, and motor cortices, whereas in the patients with nondeficit schizophrenia the control task activated broader and additional regions, including the auditory and motor cortices and the middle frontal and medial frontal cortices. In the deficit schizophrenia group, none of the activations was significant.
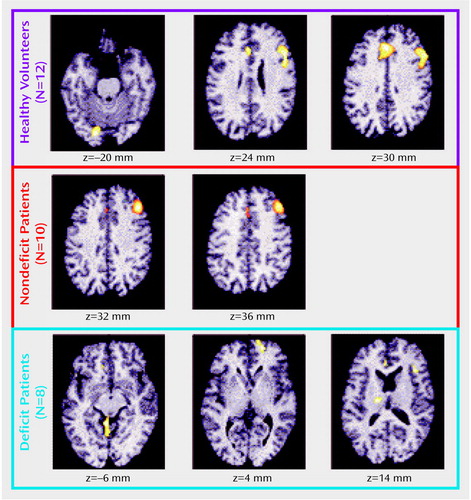
Figure 3. Changes in Cerebral Blood Flow Between a Sensory-Motor Control Task and a Decision Task in Healthy Volunteers and Schizophrenic Patients With and Without Primary Negative Symptoms (Deficit Schizophrenia)a
aDuring the decision task, the healthy volunteers showed clear activations in the cerebellum, middle and inferior frontal cortices, insula, and anterior cingulate/medial frontal cortex. The patients with nondeficit schizophrenia showed significant activation only in the right middle frontal cortex. The patients in the deficit group did not show significant activation in any brain region, despite task performance.
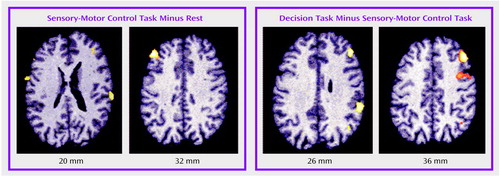
Figure 4. Brain Regions Activated More in 10 Schizophrenic Patients Without Primary Negative Symptoms Than in Eight Patients With Primary Negative Symptoms (Deficit Schizophrenia) During a Sensory-Motor Control Task and a Decision Taska
aAs shown in the left panel, the regions significantly more activated in nondeficit than deficit patients during the control task were the right and left middle frontal cortex. Right cluster size: 104 voxels with a maximum z score of 2.61. Left cluster size: 316 voxels with a maximum z score of 3.98. As shown in the right panel, the regions significantly more activated in the nondeficit patients during the decision task were the right middle frontal (two clusters) and inferior parietal cortices. Right frontal cluster sizes: 299 voxels with a maximum z score of 4.81 and 138 voxels with a maximum z score of 3.28. Right inferior parietal cluster size: 185 voxels with a maximum z score of 3.32.
1. Carpenter WT Jr, Buchanan RW: Schizophrenia. N Engl J Med 1994; 330:681-690Crossref, Medline, Google Scholar
2. Tamminga CA: Neuropsychiatric aspects of schizophrenia, in The American Psychiatric Press Textbook of Neuropsychiatry, 3rd ed. Edited by Yudofsky SC, Hales RE. Washington, DC, American Psychiatric Press, 1997, pp 855-882Google Scholar
3. Ciompi L, Muller C: [Lifestyle and Age of Schizophrenics: A Catamnestic Long-Term Study Into Old Age]. Monogr Gesamtgeb Psychiatr Psychiatry Ser 1976; 12:1-242 (German)Medline, Google Scholar
4. Bleuler M: The Schizophrenic Disorders: Long-Term Patient and Family Studies. New Haven, Conn, Yale University Press, 1978Google Scholar
5. Angst J: European long-term followup studies of schizophrenia. Schizophr Bull 1988; 14:501-513Crossref, Medline, Google Scholar
6. Harding CM: Course types in schizophrenia: an analysis of European and American studies. Schizophr Bull 1988; 14:633-643Crossref, Medline, Google Scholar
7. Huber G, Gross G, Schuttler R, Linz M: Longitudinal studies of schizophrenic patients. Schizophr Bull 1980; 6:592-605Crossref, Medline, Google Scholar
8. McGlashan TH: A selective review of recent North American long-term followup studies of schizophrenia. Schizophr Bull 1988; 14:515-542Crossref, Medline, Google Scholar
9. Strauss JS, Carpenter WT Jr, Bartko JJ: The diagnosis and understanding of schizophrenia, part III: speculations on the processes that underlie schizophrenic symptoms and signs. Schizophr Bull 1974; 11:61-69Crossref, Medline, Google Scholar
10. Andreasen NC, Olsen S: Negative v positive schizophrenia: definition and validation. Arch Gen Psychiatry 1982; 39:789-794Crossref, Medline, Google Scholar
11. Buchanan RW, Strauss ME, Kirkpatrick B, Holstein C, Breier A, Carpenter WT Jr: Neuropsychological impairments in deficit vs nondeficit forms of schizophrenia. Arch Gen Psychiatry 1994; 51:804-811Crossref, Medline, Google Scholar
12. Carpenter WT Jr, Buchanan RW: Domains of psychopathology relevant to the study of etiology and treatment in schizophrenia, in Schizophrenia: Scientific Progress. Edited by Schulz SC, Tamminga CA. New York, Oxford University Press, 1989, pp 13-22Google Scholar
13. Weinberger DR, Berman KF, Suddath R, Torrey EF: Evidence of dysfunction of a prefrontal-limbic network in schizophrenia: a magnetic resonance imaging and regional cerebral blood flow study of discordant monozygotic twins. Am J Psychiatry 1992; 149:890-897Link, Google Scholar
14. Carpenter WT Jr, Buchanan RW, Kirkpatrick B, Tamminga CA, Wood F: Strong inference, theory testing, and the neuroanatomy of schizophrenia. Arch Gen Psychiatry 1993; 50:825-831Crossref, Medline, Google Scholar
15. Crow TJ: The two-syndrome concept: origins and current status. Schizophr Bull 1985; 11:571-586Crossref, Google Scholar
16. Andreasen NC: A unitary model of schizophrenia: Bleuler’s “fragmented phrene” as schizencephaly. Arch Gen Psychiatry 1999; 56:781-787Crossref, Medline, Google Scholar
17. Dollfus S, Ribeyre JM, Petit M: Family history and deficit form in schizophrenia. Eur Psychiatry 1996; 11:260-267Crossref, Medline, Google Scholar
18. Ross DE, Kirkpatrick B, Karkowski LM, Straub RE, MacLean CJ, O’Neill FA, Compton AD, Murphy B, Walsh D, Kendler KS: Sibling correlation of deficit syndrome in the Irish Study of High-Density Schizophrenia Families. Am J Psychiatry 2000; 157:1071-1076Link, Google Scholar
19. Kirkpatrick B, Castle D, Murray RM, Carpenter WT Jr: Risk factors for the deficit syndrome of schizophrenia. Schizophr Bull 2000; 26:233-242Crossref, Medline, Google Scholar
20. Ross DE, Thaker GK, Buchanan RW, Lahti AC, Medoff D, Bartko JJ, Moran M, Hartley J: Association of abnormal smooth pursuit eye movements with the deficit syndrome in schizophrenic patients. Am J Psychiatry 1996; 153:1158-1165Link, Google Scholar
21. Ross DE, Thaker GK, Buchanan RW, Kirkpatrick B, Lahti AC, Medoff DR, Bartko JJ, Goodman J, Tien AY: Eye tracking disorder in schizophrenia is characterized by specific ocular motor defects and is associated with the deficit syndrome. Biol Psychiatry 1997; 42:781-796Crossref, Medline, Google Scholar
22. Ribeyre JM, Lesieur P, Varoquaux O, Dollfus S, Pays M, Petit M: A comparison of plasma homovanillic acid in the deficit and nondeficit subtypes of schizophrenia. Biol Psychiatry 1994; 36:230-236Crossref, Medline, Google Scholar
23. Thibaut F, Ribeyre JM, Dourmap N, Menard JF, Dollfus S, Petit M: Plasma 3-methoxy-4-hydroxyphenylglycol and homovanillic acid measurements in deficit and nondeficit forms of schizophrenia. Biol Psychiatry 1998; 43:24-30Crossref, Medline, Google Scholar
24. Tamminga CA, Thaker GK, Buchanan R, Kirkpatrick B, Alphs LD, Chase TN, Carpenter WT: Limbic system abnormalities identified in schizophrenia using positron emission tomography with fluorodeoxyglucose and neocortical alterations with deficit syndrome. Arch Gen Psychiatry 1992; 49:522-530Crossref, Medline, Google Scholar
25. Berman KF, Zec RF, Weinberger DR: Physiologic dysfunction of dorsolateral prefrontal cortex in schizophrenia. Arch Gen Psychiatry 1986; 43:126-135Crossref, Medline, Google Scholar
26. Weinberger DR, Berman KF, Zec RF: Physiologic dysfunction of dorsolateral prefrontal cortex in schizophrenia. Arch Gen Psychiatry 1986; 43:114-124Crossref, Medline, Google Scholar
27. Liddle PF, Friston KJ, Frith CD, Hirsch SR, Jones T, Frackowiak RS: Patterns of cerebral blood flow in schizophrenia. Br J Psychiatry 1992; 160:179-186Crossref, Medline, Google Scholar
28. Frith CD, Friston KJ, Herold S, Silbersweig D, Fletcher P, Cahill C, Dolan RJ, Frackowiak RSJ, Liddle PF: Regional brain activity in chronic schizophrenic patients during the performance of a verbal fluency task. Br J Psychiatry 1995; 167:343-349Crossref, Medline, Google Scholar
29. Gur RE, Mozley PD, Resnick SM, Mozley LH, Shtasel DL, Gallacher F, Arnold SE, Karp JS, Alavi A, Reivich M, Gur RC: Resting cerebral glucose metabolism in first-episode and previously treated patients with schizophrenia relates to clinical features. Arch Gen Psychiatry 1995; 52:657-667Crossref, Medline, Google Scholar
30. Silbersweig DA, Stern E, Frith C, Cahill C, Holmes A, Grootoonk S, Seaward J, McKenna P, Chua SE, Schnorr L, Jones T, Frackowiak RSJ: A functional neuroanatomy of hallucinations in schizophrenia. Nature 1995; 378:176-179Crossref, Medline, Google Scholar
31. Andreasen NC, O’Leary DS, Cizadlo T, Arndt S, Rezai K, Boles Ponto LL, Watkins GL, Hichwa RD: Schizophrenia and cognitive dysmetria: a positron-emission tomography study of dysfunctional prefrontal-thalamic-cerebellar circuitry. Proc Natl Acad Sci USA 1996; 93:9985-9990Crossref, Medline, Google Scholar
32. O’Leary DS, Andreasen NC, Hurtig RR, Kesler ML, Rogers M, Arndt S, Cizadlo T, Watkins GL, Boles Ponto LL, Kirchner PT, Hichwa RD: Auditory attentional deficits in patients with schizophrenia: a positron emission tomography study. Arch Gen Psychiatry 1996; 53:633-641Crossref, Medline, Google Scholar
33. Carter CS, Mintun M, Nichols T, Cohen JD: Anterior cingulate gyrus dysfunction and selective attention deficits in schizophrenia: [15O]H2O PET study during single-trial Stroop task performance. Am J Psychiatry 1997; 154:1670-1675Link, Google Scholar
34. Carter CS, Perlstein W, Ganguli R, Brar J, Mintun M, Cohen JD: Functional hypofrontality and working memory dysfunction in schizophrenia. Am J Psychiatry 1998; 155:1285-1287Link, Google Scholar
35. Fletcher PC, McKenna PJ, Frith CD, Grasby PM, Friston KJ, Dolan RJ: Brain activations in schizophrenia during a graded memory task studied with functional neuroimaging. Arch Gen Psychiatry 1998; 55:1001-1008Crossref, Medline, Google Scholar
36. Andreasen NC, Rezai K, Alliger R, Swayze VW II, Flaum M, Kirchner P, Cohen G, O’Leary DS: Hypofrontality in neuroleptic-naive patients and in patients with chronic schizophrenia: assessment with xenon 133 single-photon emission computed tomography and the Tower of London. Arch Gen Psychiatry 1992; 49:943-958Crossref, Medline, Google Scholar
37. Heckers S, Goff D, Schacter DL, Savage CR, Fischman AJ, Alpert NM, Rauch SL: Functional imaging of memory retrieval in deficit vs nondeficit schizophrenia. Arch Gen Psychiatry 1999; 56:1117-1123Crossref, Medline, Google Scholar
38. Holcomb HH, Lahti AC, Medoff DR, Weiler M, Dannals RF, Tamminga CA: Brain activation patterns in schizophrenic and comparison volunteers during a matched-performance auditory recognition task. Am J Psychiatry 2000; 157:1634-1645Link, Google Scholar
39. Kirkpatrick B, Buchanan RW, McKenney PD, Alphs LD, Carpenter WT Jr: The Schedule for the Deficit Syndrome: an instrument for research in schizophrenia. Psychiatry Res 1989; 30:119-123Crossref, Medline, Google Scholar
40. Overall JE, Gorham DR: The Brief Psychiatric Rating Scale. Psychol Rep 1962; 10:799-812Crossref, Google Scholar
41. Pfohl B, Blum N, Zimmerman M, Stangl D: Structured Interview for DSM-III-R Personality Disorders, Revised (SIDP-R). Iowa City, University of Iowa College of Medicine, Department of Psychiatry, 1989Google Scholar
42. Raichle ME, Martin WR, Herseovitch P, Mintun MA, Markham J: Brain blood flow measured with intravenous H215O, II: implementation and validation. J Nucl Med 1983; 24:790-798Medline, Google Scholar
43. Friston KJ, Holmes A, Poline JB, Price CJ, Frith CD: Detecting activations in PET and fMRI: levels of inference and power. Neuroimage 1996; 4:223-235Crossref, Medline, Google Scholar
44. Talairach J, Tournoux P: Co-Planar Stereotaxic Atlas of the Human Brain: Three-Dimensional Proportional System. Stuttgart, Germany, Georg Thieme, 1988Google Scholar
45. Worsley KJ, Evans AC, Marrett S, Neelin P: A three-dimensional statistical analysis for CBF activation studies in human brain. J Cereb Blood Flow Metab 1992; 12:900-918Crossref, Medline, Google Scholar
46. Holcomb HH, Medoff DR, Caudill PJ, Zhao Z, Lahti AC, Dannals RF, Tamminga CA: Cerebral blood flow relationships associated with a difficult tone recognition task in trained normal volunteers. Cereb Cortex 1998; 8:534-542Crossref, Medline, Google Scholar
47. Kornhuber J, Schultz A, Wiltfang J, Meineke I, Gleiter CH, Zöchling R, Boissl K-W, Leblhuber F, Riederer P: Persistence of haloperidol in human brain tissue. Am J Psychiatry 1999; 156:885-890Link, Google Scholar
48. Murugaiah K, Fleminger S, Theodorou A, Jenner P, Marsden CD: Persistent increase in striatal dopamine stimulated adenylate cyclase activity persists for more than 6 months but disappears after 1 year following withdrawal from 18 months cis-flupenthixol intake. Biochemistry and Pharmacology 1983; 32:2495-2499Crossref, Medline, Google Scholar
49. Wolkin A, Sanfilipo M, Wolf AP, Angrist B, Brodie JD, Rotrosen JP: Negative symptoms and hypofrontality in chronic schizophrenia. Arch Gen Psychiatry 1992; 49:959-965Crossref, Medline, Google Scholar
50. Kaplan RD, Szechtman H, Franco S, Szechtman B, Nahmias C, Garnett ES, List S, Cleghorn JM: Three clinical syndromes of schizophrenia in untreated subjects: relation to brain glucose activity measured by positron emission tomography. Schizophr Res 1993; 11:47-54Crossref, Medline, Google Scholar
51. Sabri O, Erkwoh R, Schreckenberger M, Cremerius U, Schulz G, Dickmann C, Kaiser HJ, Steinmeyer EM, Sass H, Buell U: Regional cerebral blood flow and negative/positive symptoms in 24 drug-naive schizophrenics. J Nucl Med 1997; 38:181-188Medline, Google Scholar
52. Min SK, An SK, Jon DI, Lee JD: Positive and negative symptoms and regional cerebral perfusion in antipsychotic-naive schizophrenic patients: a high-resolution SPECT study. Psychiatry Res 1999; 90:159-168Crossref, Medline, Google Scholar
53. Yuasa S, Kurachi M, Suzuki M, Kadono Y, Matsui M, Saitoh O, Seto H: Clinical symptoms and regional cerebral blood flow in schizophrenia. Eur Arch Psychiatry Clin Neurosci 1995; 246:7-12Crossref, Medline, Google Scholar
54. Kawasaki Y, Maeda Y, Sakai N, Higashima M, Yamaguchi N, Koshino K, Suzuki M, Matsuda H: Regional cerebral blood flow in patients with schizophrenia: relevance to symptom structures. Psychiatry Res 1996; 67:49-58Crossref, Medline, Google Scholar
55. Holcomb HH, Cascella NG, Thaker GK, Medoff DR, Dannals RF, Tamminga CA: Functional sites of neuroleptic drug action in the human brain: PET/FDG studies with and without haloperidol. Am J Psychiatry 1996; 153:41-49Link, Google Scholar
56. Cummings JL: Frontal-subcortical circuits and human behavior. Arch Neurol 1993; 50:873-880Crossref, Medline, Google Scholar
57. Eslinger PJ, Grattan LM: Frontal lobe and frontal-striatal substrates for different forms of human cognitive flexibility. Neuropsychologia 1993; 31:17-28Crossref, Medline, Google Scholar
58. Stuss DT, Eskes GA, Foster JK: Experimental neuropsychological studies of frontal lobe functions, in Handbook of Neuropsychology. Edited by Boller F, Spinnler H, Hendler JA. Amsterdam, Elsevier, 1994, pp 149-186Google Scholar
59. Grady CL: Neuroimaging and activation of the frontal lobes, in The Human Frontal Lobes: Function and Disorders. Edited by Miller BL, Cummings JL. New York, Guilford Press, 1999, pp 196-230Google Scholar
60. Posner MI, Cohen Y: Components of performance, in Attention and Performance. Edited by Bouma H, Bowhuis D. Hillsdale, NJ, Erlbaum, 1984, pp 531-556Google Scholar
61. Lynch JC, McClaren JW: Deficits of visual attention and saccadic eye movements after lesions of parieto-occipital cortex in monkeys. J Neurophysiol 1989; 61:74-90Crossref, Medline, Google Scholar
62. Goldberg ME, Colby CL, Duhamel JR: The representation of visuomotor space in the parietal lobe of the monkey. Cold Spring Harb Symp Quant Biol 1990; 55:729-739Crossref, Medline, Google Scholar
63. Mangun GR, Hillyard SA, Luck SJ: Electrocortical substrates of visual selective attention, in Attention and Performance. Edited by Meyer DE, Kornblum S. Cambridge, Mass, MIT Press, 1993, pp 219-243Google Scholar
64. Haxby JV, Grady CL, Horwitz B, Ungerleider LG, Mishkin M, Carson RE, Herscovitch P, Schapiro MB, Rappoport SI: Dissociation of object and spatial visual processing pathways in human extrastriate cortex. Proc Natl Acad Sci USA 1991; 88:1621-1625Crossref, Medline, Google Scholar
65. Corbetta M, Miezin FM, Shulman GL, Petersen SE: A PET study of visuospatial attention. J Neurosci 1993; 13:1202-1226Crossref, Medline, Google Scholar
66. Petersen SE, Corbetta M, Miezin FM, Shulman GL: PET studies of parietal involvement in spatial attention: comparison of different task types. Can J Exp Psychol 1994; 48:319-338Crossref, Medline, Google Scholar
67. Gevins A, Smith ME, Le J, Leong H, Bennett J, Martin N, McEvoy L, Du R, Whitfield S: High resolution evoked potential imaging of the cortical dynamics of human working memory. Electroencephalogr Clin Neurophysiol 1996; 98:327-348Crossref, Medline, Google Scholar
68. Gruzelier J, Seymour K, Wilson L, Jolley A, Hirsch S: Impairments on neuropsychologic tests of temporohippocampal and frontohippocampal functions and word fluency in remitting schizophrenia and affective disorders. Arch Gen Psychiatry 1988; 45:623-629Crossref, Medline, Google Scholar
69. Goldberg E, Seidman L: Higher cortical functions in normals and in schizophrenia: a selective review, in Handbook of Schizophrenia, vol 5: Neuropsychology, Psychophysiology and Information Processing. Edited by Steinhauer SR, Gruzelier JH, Zubin J. New York, Elsevier Science, 1991, pp 553-597Google Scholar
70. Gold JM, Carpenter C, Randolph C, Goldberg TE, Weinberger DR: Auditory working memory and Wisconsin Card Sorting Test performance in schizophrenia. Arch Gen Psychiatry 1997; 54:159-165Crossref, Medline, Google Scholar
71. Buchanan RW, Strauss ME, Breier A, Kirkpatrick B, Carpenter WT Jr: Attentional impairments in deficit and nondeficit forms of schizophrenia. Am J Psychiatry 1997; 154:363-370Link, Google Scholar
72. Kirkpatrick B, Conley RC, Kakoyannis A, Reep RL, Roberts RC: Interstitial cells of the white matter in the inferior parietal cortex in schizophrenia: an unbiased cell-counting study. Synapse 1999; 34:95-102Crossref, Medline, Google Scholar
73. Gao X-M, Sakai K, Roberts RC, Conley RR, Dean B, Tamminga CA: Ionotropic glutamate receptors and expression of N-methyl-d-aspartate receptor subunits in subregions of human hippocampus: effects of schizophrenia. Am J Psychiatry 2000; 157:1141-1149Link, Google Scholar
74. Green MF: What are the functional consequences of neurocognitive deficits in schizophrenia? Am J Psychiatry 1996; 153:321-330Link, Google Scholar
75. Ho B-C, Nopoulos P, Flaum M, Arndt S, Andreasen NC: Two-year outcome in first-episode schizophrenia: predictive value of symptoms for quality of life. Am J Psychiatry 1998; 155:1196-1201Link, Google Scholar