Persistence of Haloperidol in Human Brain Tissue
Abstract
OBJECTIVE: After discontinuation of neuroleptic drugs, their antipsychotic and antiparkinsonian effects are still present for a prolonged period. It is not known whether the extended effects of neuroleptic drugs in humans are due to the continued presence of drug in brain tissue or to long-lasting drug-induced physiologic changes. The aim of this study was to directly examine haloperidol concentrations in human brain tissue in relation to drug-free time. METHOD: Haloperidol concentrations were measured in five regions (temporal cortex, cingulate gyrus, caudate nucleus, dentate nucleus, corpus callosum) of the postmortem brains of 11 patients previously treated with haloperidol. Haloperidol was analyzed by means of high-performance liquid chromatography with ultraviolet detection. The half-life in brain tissue was estimated by a population kinetic analysis. RESULTS: Haloperidol concentrations in the human brain tissue were 10–30 times higher than optimal serum concentrations used in the treatment of schizophrenia. Haloperidol concentrations appeared to be homogeneously distributed across different brain areas within a single patient. There was no apparent relation between duration of treatment and mean haloperidol concentration. Higher doses of haloperidol seemed to be related to higher concentrations in brain tissue. The elimination half-life from brain tissue was calculated to be 6.8 days. CONCLUSIONS: The results may have implications for clinical treatment decisions and the design of clinical research protocols. Patients exposed to haloperidol cannot be considered to be free of residual effects of the drug for a number of weeks after withdrawal.
After discontinuation of neuroleptic drugs, their effects are still present for a long time. Clinical relapse in schizophrenic patients may not occur for weeks or months after the administration of drugs is stopped (1). Side effects such as parkinsonian symptoms and neuroleptic malignant syndrome may persist for weeks after discontinuation of neuroleptic drugs (2, 3).
Haloperidol is one of the most frequently prescribed neuroleptic agents. The serum elimination of haloperidol in humans slows with time after dosing, and near-terminal elimination half-life may be measured in days rather than hours (4, 5). A comparable effect is evident in experimental animals. A single dose of haloperidol resulted in signs of central dopaminergic blockade for at least 20 days after administration (6–8). Campbell and co-workers (9) found a half-life of 7.6 days for the disappearance of antidopaminergic effects of a single injection of haloperidol in rats. Similarly, Cohen and colleagues (6, 7) directly measured behavioral effects and brain haloperidol concentrations in rats after single doses of haloperidol. They found a terminal elimination half-life of the drug in the brain of 6.6 days (7) and 16.7 days (6). These results may explain the prolonged clinical effects of neuroleptic drugs even after short-term treatment.
It is not known whether the extended effects of neuroleptic drugs in humans are due to the continued presence of drug in tissue or to long-lasting drug-induced physiologic changes. The aim of the present study was to directly examine haloperidol concentrations in human brain tissue in relation to drug-free time.
METHOD
Brain tissue was taken at autopsy from 11 subjects who had been treated with oral doses of haloperidol. The brains were collected between 1989 and 1994. Case notes were examined in detail to establish the duration and dosage of haloperidol as well as the drug-free time before death. The drug-free time was calculated as the difference between the time of last intake of the drug and the time of death. The postmortem time was calculated as the difference between the time of death and the freezing of brain tissue. The histories of these patients, with prescribed drug dosages, duration of treatment, drug-free time before death, and concomitant medication are shown in Table 1. Tissue samples were taken from several brain regions from either side of the brain (temporal cortex, cingulate gyrus, caudate nucleus, dentate nucleus, corpus callosum); it was not possible to investigate tissue samples of all these regions from every brain. Brain samples were also collected from patients who had never received haloperidol. Postmortem handling of the autopsy material was similar in all cases and was performed according to a standardized procedure (10). The samples were placed in a freezer at –80°C until analysis. The duration of storage at low temperature was between 2.2 and 7.1 years.
Extraction and Quantitation of Haloperidol
Techniques of extraction, chromatography, and detection were modified from those in a previous publication (11). Brain samples were cleaned of visible blood and weighed, and 0.4–0.9 g were homogenized with the use of a Sonifier (Branson-250, Danbury, Conn.) in 1 M NaHCO3 together with 60 ng of the internal standard, bromperidol. The alkalinized homogenate was extracted with hexane/isoamyl alcohol (98.5%/1.5% by volume) by vigorous rotary action. The layers were separated by centrifugation, and the organic phase was aspirated. The butyrophenones were back-extracted into dilute acid and, after alkalinization, again extracted into the hexane/isoamyl alcohol mixture. The resulting organic phase was evaporated under a gentle stream of nitrogen, and the tubes were sealed and stored in darkness at 4°C until high-performance liquid chromatography analysis, which was performed within 2 days. All samples were reconstituted in 150 µl of the mobile phase, and 100 µl were injected into the high-performance liquid chromatography system.
The high-performance liquid chromatography system consisted of a Constametric 4100 solvent delivery system (LDC Analytical, Gelnhausen, Germany), a manual injection valve with a 100-µl loop (Rheodyne Sokati, Rohnert Park, Calif.), an Astec-Pak 5-µM spherical C8 cartridge column (4.6 × 15 cm; Astec, Whippany, N.J.), and a Spectromonitor 4100-wavelength ultraviolet detector (LDC Analytical). The column was operated at 21°C, and ultraviolet detection was performed at 247 nm. The mobile phase consisted of acetonitrile/water (50%/50% by volume; high-performance liquid chromatography grade) titrated to pH 6.5 with 85% orthophosphoric acid. The mobile phase was pumped at a rate of 2.0 ml/min. Chromatograms were recorded on an IBM-compatible personal computer with LDC Lctalk Software. Haloperidol and bromperidol had stable and reproducible retention times that allowed identification and quantitation without interference of endogenous or other psychotropic substances. The concentrations of haloperidol were calculated by using manually determined peak height ratios of haloperidol to the internal standard, bromperidol. Haloperidol content was expressed relative to the wet weight of brain tissue (nanograms per gram).
The extraction recovery was assessed by adding a standard amount of haloperidol to brain tissue of patients without a known history of haloperidol medication. Absolute recovery of the extraction of haloperidol from brain tissue was 93.5% (SD=5.4%). Within- and between-day coefficients of variation in human brain were below 10%. The detection limit was about 4 ng/g. The peak height of haloperidol showed a linear relation to concentrations over a range of at least 4–3000 ng/g. Calibration curves were consistently linear and passed through the origin.
Bromperidol was donated by Janssen Research Products (Beerse, Belgium). Haloperidol was purchased from Sigma Pharmaceuticals (Deisenhofen, Germany). All other chemicals were purchased from Merck and Co. (Darmstadt, Germany) and were of the purest grade available.
Statistical Analysis
Data were analyzed in an exploratory manner. Since there was only a single measurement per individual in various brain regions and insufficient data to describe the pharmacokinetic profile in each individual patient, a population pharmacokinetic analysis (12) was performed with the computer software program NONMEM (13). The aim of this analysis was to estimate the average half-life of haloperidol in the brain tissue of the group of patients investigated. Several assumptions had to be made. Steady-state conditions in brain tissue were assumed when the duration of treatment was 7 or more days; otherwise, multiple dosing was modeled. The residual variability was fixed to the estimated coefficient of variation of the analytical method, that is, 10%. Individual Bayes post hoc estimates (14) were calculated for the volumes of distribution. For the mean subject in the study group, a dose of 4 mg of haloperidol and steady-state conditions were used. Subroutines ADVAN2 and TRANS1 of the package NONMEM IV, version 2.1, were used. The pharmacokinetic model was C=D·1000/V·e(–k·t), where C=concentration (ng/g), D=dose of drug (mg), V=volume of distribution (liters), k=elimination constant (1/hour), and t=drug-free time after last dose (days).
The model describes the concentration time curves in a one-compartment model after bolus injection. The estimation of an absorption constant was not attempted because of the low number of data points. The analysis used mean concentrations of haloperidol in brain tissue.
Correlations between mean haloperidol concentrations and independent variables were assessed by the Spearman test. Mean values are reported.
RESULTS
The haloperidol concentration was below the detection limit in the three brain tissue samples of patients never treated with haloperidol. Haloperidol was detected in the brain tissue of all patients previously treated with the drug, with concentrations ranging from 8.9 ng/g (caudate nucleus, patient 11) to 226.7 ng/g (temporal cortex, patient 9). Haloperidol concentrations appeared to be homogeneously distributed across different brain areas within a single individual (Figure 1, A). To investigate interindividual differences, mean values of haloperidol concentrations were calculated for every patient. In contrast to the intraindividual differences between several brain regions, the interindividual differences between patients were considerable. The highest mean haloperidol concentration was found in a patient on a relatively high dose of haloperidol that had been given until death (patient 9). Low concentrations in brain tissue were found when haloperidol had been withdrawn for more than a week (patients 1 and 7).
Figure 1 shows mean haloperidol concentrations in brain tissue in relation to duration of treatment, dose of the drug, and drug-free time before death. There was no apparent relation between duration of treatment and mean haloperidol concentration. Even after treatment for only 3 days, considerable brain concentrations were measured (Figure 1, B). Higher doses of haloperidol seemed to be related to higher concentrations in brain tissue (Figure 1, C). The brain concentration decreased within days after drug withdrawal (Figure 1, D). In the population kinetic analysis with NONMEM (inset in Figure 1, D) a brain elimination half-life of 6.8 days was calculated for the mean subject (k=0.00427 1/hour, V=266.1). The asymptotic standard deviation, as an estimate of the precision of the calculated elimination constant, was obtained from the variance-covariance matrix by using the NONMEM program. The asymptotic standard deviation of the elimination constant was 0.00164, resulting in a range of brain elimination half-lives from 4.9 to 11.0 days. Postmortem time was not significantly related to mean haloperidol concentration (r=0.35, N=11, p=0.29). Furthermore, there was no significant correlation between storage time of brain tissue at –80°C and mean haloperidol concentration (r=–0.37, N=11, p=0.26), which is consistent with a high chemical stability of haloperidol during prolonged storage (15).
DISCUSSION
We measured haloperidol concentrations in human postmortem brain tissue. Because of its high selectivity and sensitivity, a high-performance liquid chromatography method was used for quantification of haloperidol. High-performance liquid chromatography methods are well-suited to situations like this, where several different drugs have to be reliably separated from each other (11, 16). Korpi et al. (17) previously quantified haloperidol concentrations in postmortem human brain tissue. That study used a smaller sample of cases and was undertaken to understand the conversion of haloperidol to the reduced alcoholic metabolite in human brain tissue. These authors did not further investigate the factors that might have an influence on haloperidol concentrations. The present study was undertaken to understand the elimination pharmacokinetics of haloperidol in human brain tissue.
We had the opportunity to investigate two patients in our series who had prolonged drug-free time. Much of the data depends on these individuals. The main finding of our investigation is that the brain elimination half-life of haloperidol is about 1 week. Therefore, it is likely that the slow disappearance of neuroleptic effects after the discontinuation of therapy is related to a slow elimination of the drug from the brain. However, the persistence of physiologic effects after complete clearance of the drug may also occur. The estimated elimination half-life of haloperidol from human brain tissue is in close agreement with values derived from the measurement of brain haloperidol concentrations (7, 18) and antidopaminergic effects (7, 9) in experimental animals. The elimination half-life appears to be longer in brain tissue compared with serum values, even when one is examining terminal elimination half-lives of haloperidol (4, 5, 19).
The time course of removal of neuroleptic drugs from human brain tissue has been indirectly estimated through D2 dopamine receptor occupancy in human patients by using postmortem D2 receptor measurements, in vivo positron emission tomography (PET), and in vivo single photon emission computed tomography. In postmortem studies, high dissociation constant (Kd) values for the D2 receptor reflect residual neuroleptic drug at the receptor. A decline of Kd values to control levels within 2 weeks after withdrawal of neuroleptic drugs has been reported for postmortem human brain putamen (20). Baron and co-workers (21, 22), using PET, reported on normal receptor availability within 5–15 days after withdrawal of oral neuroleptics. In the PET examination of a single patient, Farde and colleagues (23) found that after withdrawal of oral haloperidol for up to 54 hours, there was a rapid fall in serum drug levels without a significant reduction in D2 receptor occupancy. Withdrawal of depot preparations of fluphenazine decanoate (24) and haloperidol decanoate (25) resulted in high D2 dopamine receptor occupancy for several months.
The concentrations of haloperidol reported here are of the same order of magnitude as previously reported values in human (17) and rat (6, 17, 26, 27) brain tissue. The concentrations reported by Korpi et al. (17) for the human brain were in the range between 64 and 3947 ng/g. The highest concentrations reported were 10 times higher than the concentrations found in the present study. However, the patients in that study received higher doses of haloperidol (up to 100 mg/day) and may have had very short drug-free times. When the brain concentrations measured in the present study are compared with clinically effective plasma haloperidol levels reported in the literature (28, 29), the brain-to-blood concentration ratio in humans is about 10–30, even after short-term treatment. Similar concentration ratios were found by Korpi et al. (17) in two subjects. These results are paralleled in animal experiments. A brain-to-blood concentration ratio of 22 for haloperidol was found in rats both after a single dose (27) and after subchronic administration (26).
Haloperidol appeared to be homogeneously distributed across the brain areas investigated. It is unlikely that a homogeneous distribution happened secondarily during the postmortem time. The postmortem interval was less than 6 hours in all but three cases. Previous data (17) suggest that an unequal equilibrium concentration of butyrophenones in certain body areas is retained after death. Korpi and co-workers (17) found no consistent differences in haloperidol concentration across three regions of two postmortem human brains. Similar results have been obtained with the rat brain, where no relevant differences in the concentration of haloperidol between the striatum, limbic system, and cerebellum after a single intraperitoneal injection were found (18). On the other hand, in experimental animals, a preferential accumulation of butyrophenones in brain areas with a large dopamine concentration has previously been suggested (17, 30, 31).
It has been hypothesized that the therapeutic delay of antidepressant and neuroleptic drugs might be related to a slow uptake of the drug into human brain tissue (32). A slow uptake has been found for amantadine in postmortem human brain tissue (33) and for fluoxetine in vivo (34). We did not find a slow uptake for haloperidol in the present study. Even after 3 days of treatment with haloperidol, the concentration in brain tissue was comparable to that for long-term treatment. This indicates that haloperidol rapidly accumulates in brain tissue. Similar results were found in experimental animals. As stated above, high brain concentrations of haloperidol were found even after a single administration of the drug in rats (6, 17, 27), while subchronic treatment did not result in a further increase of the blood-to-brain concentration ratio (17, 26).
During long-term therapy with neuroleptic drugs, about 30% of patients develop tardive dyskinesia, which remains as a chronic disease after withdrawal of the drug in a substantial portion of the patients. The cause of tardive dyskinesia has been associated with neurotoxic properties of neuroleptic drugs. Recently, similarities between the metabolism of haloperidol and the dopaminergic pro-neurotoxin 1-methyl-4-phenyl-1, 2, 3, 6-tetrahydropyridine (MPTP) have been identified (35). Haloperidol is similar in structure to MPTP, which is metabolized to the neurotoxic 1-methyl-4-phenylpyridinium species (MPP+). Haloperidol itself is also metabolized to a pyridinium metabolite, 4-(4-chlorophenyl)-1-4-fluorophenyl-4-oxobutylpyridinium (HPP+) (36, 37). HPP+ and MPP+ have neurotoxic effects on dopaminergic and serotonergic neurons (37–39). The neurotoxic pyridinium metabolite is also found in human brain (40, 41) and may contribute to slowly appearing and irreversible extrapyramidal side effects that occur after long-term treatment. The high concentrations and the long half-life of haloperidol in human brain tissue found in the present study suggest that neurotoxic metabolites may be present in brain tissue for several weeks, even after short-term treatment.
In conclusion, we directly measured concentrations of haloperidol under therapeutic conditions in human brain tissue. Concentrations in brain tissue are 10–30 times higher than the optimum serum concentrations in the treatment of schizophrenia. The estimated elimination half-life of the drug in brain tissue is 6.8 days. After two half-lives (about 2 weeks) there is still a considerable amount of haloperidol in brain tissue. The brain concentration at this time is in the order of therapeutically relevant plasma concentrations. The results reported here may have implications for clinical treatment decisions and the design of clinical research protocols. Patients exposed to haloperidol cannot be considered to be free of residual effects of the drug for a number of weeks after withdrawal, even after acute treatment and even when the drug concentration is below the detection level in the blood.
Presented in abstract form at the 6th World Congress of Biological Psychiatry, Nice, France, June 22–27, 1997. Received Aug. 24, 1998; revision received Nov. 30, 1998; accepted Dec. 10, 1998. From the Department of Psychiatry, University of Göttingen; the Department of Psychiatry, University of Würzburg, Germany; the Department of Clinical Pharmacology, University of Göttingen; the Lower Austria Landeskrankenhaus for Psychiatry, Mauer/Amstetten; and the Landeskrankenhaus for Psychiatry, Linz, Austria. Address reprint requests to Dr. Kornhuber, Department of Psychiatry, University of Göttingen, von-Siebold-Str. 5, D-37075 Göttingen, Germany; [email protected] (e-mail). The authors thank Mrs. C. Jambor and Mr. T. Elpel for technical assistance.
![]() |
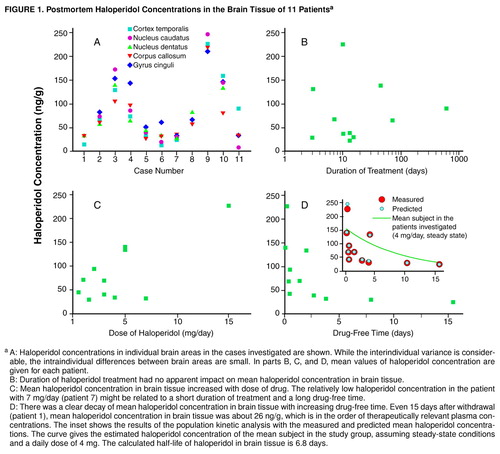
FIGURE 1. Postmortem Haloperidol Concentrations in the Brain Tissue of 11 Patientsa
aA: Haloperidol concentrations in individual brain areas in the cases investigated are shown. While the interindividual variance is considerable, the intraindividual differences between brain areas are small. In parts B, C, and D, mean values of haloperidol concentration are given for each patient. B: Duration of haloperidol treatment had no apparent impact on mean haloperidol concentration in brain tissue. C: Mean haloperidol concentration in brain tissue increased with dose of drug. The relatively low haloperidol concentration in the patient with 7 mg/day (patient 7) might be related to a short duration of treatment and a long drug-free time. D: There was a clear decay of mean haloperidol concentration in brain tissue with increasing drug-free time. Even 15 days after withdrawal (patient 1), mean haloperidol concentration in brain tissue was about 26 ng/g, which is in the order of therapeutically relevant plasma concentrations. The inset shows the results of the population kinetic analysis with the measured and predicted mean haloperidol concentrations. The curve gives the estimated haloperidol concentration of the mean subject in the study group, assuming steady-state conditions and a daily dose of 4 mg. The calculated half-life of haloperidol in brain tissue is 6.8 days.
1. Viguera AC, Baldessarini RJ, Hegarty JD, van Kammen DP, Tohen M: Clinical risk following abrupt and gradual withdrawal of maintenance neuroleptic treatment. Arch Gen Psychiatry 1997; 54:49–55Crossref, Medline, Google Scholar
2. Addonizio G, Susman VL, Roth SD: Neuroleptic malignant syndrome: review and analysis of 115 cases. Biol Psychiatry 1987; 22:1004–1020Google Scholar
3. Kornhuber J, Weller M: Neuroleptic malignant syndrome. Curr Opin Neurol 1994; 7:353–357Crossref, Medline, Google Scholar
4. Hubbard JW, Ganes D, Midha KK: Prolonged pharmacologic activity of neuroleptic drugs. Arch Gen Psychiatry 1987; 44:99–100Crossref, Medline, Google Scholar
5. Ereshefsky L, Jann MW, Saklad SR, Davis CM: Bioavailability of psychotropic drugs: historical perspective and pharmacokinetic overview. J Clin Psychiatry 1986; 47(Sept suppl):6–15Google Scholar
6. Cohen BM, Babb S, Campbell A, Baldessarini RJ: Persistence of haloperidol in the brain. Arch Gen Psychiatry 1988; 45:879–880Crossref, Medline, Google Scholar
7. Cohen BM, Tsuneizumi T, Baldessarini RJ, Campbell A, Babb SM: Differences between antipsychotic drugs in persistence of brain levels and behavioral effects. Psychopharmacology (Berl) 1992; 108:338–344Crossref, Medline, Google Scholar
8. Campbell A, Baldessarini RJ: Prolonged pharmacologic activity of neuroleptics (letter). Arch Gen Psychiatry 1985; 42:637Crossref, Medline, Google Scholar
9. Campbell A, Baldessarini RJ, Teicher MH, Kula NS: Prolonged antidopaminergic actions of single doses of butyrophenones in the rat. Psychopharmacology (Berl) 1985; 87:161–166Crossref, Medline, Google Scholar
10. Gsell W, Lange KW, Pfeuffer R, Heckers S, Heinsen H, Senitz D, Jellinger K, Ransmayr G, Wichart I, Vock R, Beckmann H, Riederer P: How to run a brain bank: a report from the Austro-German brain bank. J Neural Transm Suppl 1993; 39:31–70Medline, Google Scholar
11. Parkinson D: Sensitive analysis of butyrophenone neuroleptics by high-performance liquid chromatography with ultraviolet detection at 254 nm. J Chromatogr 1985; 341:465–472Crossref, Medline, Google Scholar
12. Aarons L: Population pharmacokinetics: theory and practice. Br J Clin Pharmacol 1991; 32:669–670Medline, Google Scholar
13. Beal SL, Sheiner LB: NONMEM Users Guide, Part I: Users Basic Guide: Technical Report of the Division of Clinical Pharmacology. San Francisco, University of California, 1980Google Scholar
14. Sheiner LB, Beal SL: Bayesian individualization of pharmacokinetics: simple implementation and comparison with non-Bayesian methods. J Pharm Sci 1982; 71:1344–1348Google Scholar
15. Demoen PJAW: Properties and analysis of haloperidol and its dosage form. J Pharm Sci 1961; 50:350–353Crossref, Medline, Google Scholar
16. Curry SH, Brown EA, Hu OY, Perrin JH: Liquid chromatographic assay of phenothiazine, thioxanthene and butyrophenone neuroleptics and antihistamines in blood and plasma with conventional and radial compression columns and UV and electrochemical detection. J Chromatogr 1982; 231:361–376Crossref, Medline, Google Scholar
17. Korpi ER, Kleinman JE, Costakos DT, Linnoila M, Wyatt RJ: Reduced haloperidol in the post-mortem brains of haloperidol-treated patients. Psychiatry Res 1984; 11:259–269Crossref, Medline, Google Scholar
18. Öhman R, Larsson M, Nilsson IM, Engel J, Carlsson A: Neurometabolic and behavioural effects of haloperidol in relation to drug levels in serum and brain. Naunyn Schmiedebergs Arch Pharmacol 1977; 299:105–114Crossref, Medline, Google Scholar
19. Forsman A, Öhman R: Pharmacokinetic studies on haloperidol in man. Curr Ther Res Clin Exp 1976; 20:319–336Medline, Google Scholar
20. Kornhuber J, Riederer P, Reynolds GP, Beckmann H, Jellinger K, Gabriel E:3H-Spiperone binding sites in post-mortem brains from schizophrenic patients: relationship to neuroleptic drug treatment, abnormal movements, and positive symptoms. J Neural Transm 1989; 75:1–10Google Scholar
21. Cambon H, Baron JC, Boulenger JP, Loc’h C, Zarifian E, Maziere B: In vivo assay for neuroleptic receptor binding in the striatum: positron tomography in humans. Br J Psychiatry 1987; 151:824–830Crossref, Medline, Google Scholar
22. Baron JC, Martinot JL, Cambon H, Boulenger JP, Poirier MF, Caillard V, Blin J, Huret JD, Loc’h C, Maziere B: Striatal dopamine receptor occupancy during and following withdrawal from neuroleptic treatment: correlative evaluation by positron emission tomography and plasma prolactin levels. Psychopharmacology (Berl) 1989; 99:463–472Crossref, Medline, Google Scholar
23. Farde L, Wiesel F-A, Halldin C, Sedvall G: Central D2-dopamine receptor occupancy in schizophrenic patients treated with antipsychotic drugs. Arch Gen Psychiatry 1988; 45:71–76Crossref, Medline, Google Scholar
24. Harasko-van der Meer C, Brücke T, Wenger S, Fischer P, Deecke L, Podreka I: Two cases of long term dopamine D2 receptor blockade after depot neuroleptics. J Neural Transm Gen Sect 1993; 94:217–221Crossref, Medline, Google Scholar
25. Nyberg S, Farde L, Halldin C: Delayed normalization of central D2 dopamine receptor availability after discontinuation of haloperidol decanoate: preliminary findings. Arch Gen Psychiatry 1997; 54:953–958Crossref, Medline, Google Scholar
26. Tsuneizumi T, Babb SM, Cohen BM: Drug distribution between blood and brain as a determinant of antipsychotic drug effects. Biol Psychiatry 1992; 32:817–824Crossref, Medline, Google Scholar
27. Sunderland T, Cohen BM: Blood to brain distribution of neuroleptics. Psychiatry Res 1987; 20:299–305Crossref, Medline, Google Scholar
28. Volavka J, Cooper TB, Czobor P, Meisner M: Plasma haloperidol levels and clinical effects in schizophrenia and schizoaffective disorder. Arch Gen Psychiatry 1995; 52:837–845Crossref, Medline, Google Scholar
29. McEvoy JP, Hogarty GE, Steingard S: Optimal dose of neuroleptic in acute schizophrenia: a controlled study of the neuroleptic threshold and higher haloperidol dose. Arch Gen Psychiatry 1991; 48:739–745Crossref, Medline, Google Scholar
30. Laduron PM, Janssen PF, Leysen JE: Spiperone: a ligand of choice for neuroleptic receptors, 2: regional distribution and in vivo displacement of neuroleptic drugs. Biochem Pharmacol 1978; 27:317–321Crossref, Medline, Google Scholar
31. Janssen PAJ, Soudijn W, Wijngaarden I, Dresse A: Pimozide, a chemically novel, highly potent and orally long-acting neuroleptic drug, III: regional distribution of pimozide and of haloperidol in the dog brain. Arzneimittelforschung 1968; 18:282–287Medline, Google Scholar
32. Kornhuber J, Retz W, Riederer P: Slow accumulation of psychotropic substances in the human brain: relationship to therapeutic latency of neuroleptic and antidepressant drugs? J Neural Transm Suppl 1995; 46:311–319Google Scholar
33. Kornhuber J, Quack G, Danysz W, Jellinger K, Danielczyk W, Gsell W, Riederer P: Therapeutic brain concentration of the NMDA receptor antagonist amantadine. Neuropharmacology 1995; 34:713–721Crossref, Medline, Google Scholar
34. Karson CN, Newton JEO, Livingston R, Jolly JB, Cooper TB, Sprigg J, Komoroski RA: Human brain fluoxetine concentrations. J Neuropsychiatry Clin Neurosci 1993; 5:322–329Crossref, Medline, Google Scholar
35. Gorrod JW, Fang J: On the metabolism of haloperidol. Xenobiotica 1993; 23:495–508Crossref, Medline, Google Scholar
36. Subramanyam B, Woolf T, Castagnoli N Jr: Studies on the in vitro conversion of haloperidol to a potentially neurotoxic pyridinium metabolite. Chem Res Toxicol 1991; 4:123–128Crossref, Medline, Google Scholar
37. Subramanyam B, Rollema H, Woolf T, Castagnoli N Jr: Identification of a potentially neurotoxic pyridinium metabolite of haloperidol in rats. Biochem Biophys Res Commun 1990; 166:238–244Crossref, Medline, Google Scholar
38. Rollema H, Skolnik M, D’Engelbronner J, Igarashi K, Usuki E, Castagnoli N Jr: MPP+-like neurotoxicity of a pyridinium metabolite derived from haloperidol: in vivo microdialysis and in vitro mitochondrial studies. J Pharmacol Exp Ther 1994; 268:380–387Medline, Google Scholar
39. Bloomquist J, King E, Wright A, Mytilineou C, Kimura K, Castagnoli K, Castagnoli N Jr:1-Methyl-4-phenylpyridinium-like neurotoxicity of a pyridinium metabolite derived from haloperidol: cell culture and neurotransmitter uptake studies. J Pharmacol Exp Ther 1994; 270:822–830Google Scholar
40. Eyles DW, McGrath JJ, Pond SM: Formation of pyridinium species of haloperidol in human liver and brain. Psychopharmacology (Berl) 1996; 125:214–219Crossref, Medline, Google Scholar
41. Eyles DW, Avent KM, Stedman TJ, Pond SM: Two pyridinium metabolites of haloperidol are present in the brain of patients at post-mortem. Life Sci 1997; 60:529–534Crossref, Medline, Google Scholar