Brain Activation Patterns in Schizophrenic and Comparison Volunteers During a Matched-Performance Auditory Recognition Task
Abstract
OBJECTIVE: The biological characteristics of schizophrenia are often studied by using functional imaging techniques. However, since volunteers with schizophrenia routinely fail to perform as accurately or as quickly as healthy volunteers, it is difficult to ascertain whether a particular deficit in blood flow to a brain region is due to behavior or to the underlying illness. In this report, investigators used an auditory recognition task to assess brain blood flow patterns and behavioral correlates of schizophrenic patient volunteers trained on the task. METHOD: Twelve healthy volunteers and 18 volunteers with schizophrenia were trained to make tone frequency recognitions. Accuracy and stimuli were matched between groups. Participants were required to press a button to indicate whether a briefly presented tone was the high-frequency (1500 Hz) reference tone or one of a lower frequency level (level chosen to elicit an 80% accuracy score). Subjects underwent bolus [15O]H2O blood flow positron emission tomography during inactive rest, a sensory motor control condition, and the decision task. Blood flow patterns were assessed between conditions and between groups. RESULTS: As a group, the patients with schizophrenia (who performed as quickly and accurately as the comparison subjects) exhibited significantly less change in regional cerebral blood flow (rCBF) to the anterior cingulate and supplementary motor cortices when switching from the sensory motor control to the decision condition. There were also marked between-group differences in correlations between rCBF and response time. Whereas the comparison subjects exhibited progressively greater blood flow to the frontal cortex in association with longer response times, the schizophrenic patients exhibited progressively lower blood flow in conjunction with extended response times. CONCLUSIONS: The failure to appropriately enhance cingulate activity when engaged in a demanding task and the progressive, time-dependent decline in frontal blood flow suggest that patients with schizophrenia are unable to make optimal use of frontocingulate systems when maximally engaged in high-error tasks.
Schizophrenia is a disorder of thought, cognition, and affect caused by a functional abnormality of the central nervous system. The psychotic symptoms and cognitive abnormalities that characterize this illness depend on chemical and structural lesions in the brain. Early imaging studies generally used positron emission tomography (PET) in conjunction with the metabolic radiotracer [18F]fluorodeoxyglucose (FDG) (1–3) or single photon emission computed tomography with Xenon inhalation (4, 5) to compare a schizophrenic subject group to healthy comparison subjects in a resting state. Subsequently, brain-mapping strategies changed to assess neural activity changes within and between groups: investigators sought to measure differences in the distribution or magnitude of regional functional patterns between schizophrenic patients and healthy comparison subjects. In order to probe brain behaviors, particularly those that appear dysfunctional in schizophrenic illness (6–8) and to induce more uniform brain activity (9, 10), task behaviors during imaging were added to many studies. Later, techniques to assess regional cerebral blood flow (rCBF) both with PET (11) and with functional magnetic resonance imaging (MRI) (12) were developed; these techniques provided more information per experiment and greater spatial resolution. Unfortunately, the variety of functional imaging techniques and approaches that are used to investigate schizophrenia have often generated seemingly inconsistent or overly complex results, which has led to difficulty generalizing about pathophysiology.
PET associated with glucose metabolism measures such as FDG integrated regional neural activity over longer time periods (i.e., 30–40 minutes) than either PET studies that use [15O]H2O to assess rCBF (40–60 seconds) or functional MRI (4–6 seconds). Many rCBF studies that have incorporated task behavior into the design have intentionally selected cognitive tasks on which schizophrenic subjects are impaired in order to differentiate the brain areas related to these schizophrenia deficits (13–15). However, differences in task performance between subject groups can complicate and potentially confound the interpretation of the functional imaging results. Hierarchical task conditions combined with a subtraction analysis demonstrate many design advantages for imaging studies, such as controlling for extraneous variables, stabilizing brain activity, and probing specific, illness-related brain functions. However, with a task chosen to discriminate between schizophrenic patients and healthy comparison subjects, differences in task performance will likely in themselves cause differences in brain activity patterns. These differing activations may not necessarily be associated with the processing difficulty in schizophrenia but may merely be a reflection of group performance differences. These issues are magnified when floor or ceiling performance effects are evident in either group.
The study reported here used a difficult repetitive auditory recognition task in which performance of the patients with schizophrenia and the comparison subjects was fixed at the same level of accuracy. The schizophrenic patients and the comparison subjects were able to perform an auditory discrimination task equally by slight modifications of the task stimuli (a fixed reference stimulus and a variable test stimulus). When two tones differ by large frequency differences, subjects can easily determine which tone is relatively high and which is relatively low in frequency. But when the two tones are made similar (e.g., 1500 Hz versus 1490 Hz), then it becomes difficult to recognize which tone is relatively high or low with respect to the other (16, 17). In this study, by adjusting the frequency similarity, we made it more or less difficult to ascertain whether the presented auditory stimulus was the high-frequency reference tone (always 1500 Hz) or a tone of varying lower frequencies.
This study sought to identify regional functional differences between schizophrenic and comparison subjects under the conditions of similar performance of an auditory recognition task, one that is known to produce patterns of activation in frontal, cingulate, sensory cortex, and cerebellar regions (18, 19). Patterns of activation associated with inactive rest, a sensory motor control condition, and the decision task were compared and contrasted between the two groups. Also, to better understand the relationships between the task behavior and underlying neural activity, correlations between response time and rCBF were determined.
Method
Subjects
The comparison group consisted of 12 healthy, right-handed volunteers recruited from the community through newspaper advertisements and screened by telephone for good medical health and the lack of any major psychiatric illness. Further screening involved the administration of the Structured Clinical Interview for DSM-III-R—Non-Patient Edition and the Structured Clinical Interview for DSM-III-R Personality Disorders to rule out current or past history of axis I or axis II disorders, respectively. Family history of schizophrenia in any first-degree relative was ruled out by interview. Six comparison group subjects were male, and six were female. Their mean age was 28 years (SD=4.6).
Eighteen medically healthy schizophrenic inpatients were recruited from the Residential Research Unit of the Maryland Psychiatric Research Center in Baltimore. Each patient was administered the Structured Clinical Interview for DSM-III-R at hospital admission. Two research psychiatrists reached a consensus diagnosis of DSM-III-R schizophrenia, which was based on the clinical interview plus all other sources of data. All but one of the patients were right-handed. Fifteen were male, and three were female. Their mean age was 31 years (SD=7.7). The socioeconomic status of their family of origin was 2.7 (SD=1.1). The average age at onset was 21.0 years (SD=5.5), and the mean duration of their illness was 9.5 years (SD=8.2). The average duration of antipsychotic treatment was 6.4 years (SD=5.2).
The schizophrenic patient volunteers, all inpatients, were treated with a fixed dose (0.3 mg/kg) of haloperidol and no other neurologically active medications for at least 4 weeks before the start of the study. The haloperidol treatment was then entirely withdrawn (mean=31 days, SD=17, range=6–61) before the PET scan. Symptoms of schizophrenia were assessed repeatedly at baseline, during haloperidol treatment, and during the subsequent drug-free period. Brief Psychiatric Rating Scale (BPRS) (20) symptoms were rated by two trained raters. At the time of the brain scan studies, the schizophrenic patient volunteers had an average total BPRS score of 32 (SD=9.7). Patients tolerated medication withdrawal without evidence of serious clinical relapse.
All subjects provided written informed consent. The research protocols were reviewed and approved by clinical investigation committees at the University of Maryland School of Medicine and the Johns Hopkins Medical Institutes.
Task Description
The decision condition was a forced-choice auditory recognition task. Subjects were instructed to press a button held in their right hand when they recognized a high-frequency reference tone (always 1500 Hz) and to press the button in their left hand when they recognized that the tone was of lower frequency (800–1492 Hz). Subjects were told to push the button as soon as they heard the tone. An equal number of high and low tones were randomly presented in each stimulus condition; there were 100 trials per training set and 60 trials per imaging set. Tones lasted 100 msec. Following termination of the signal, the subject had 1.9 seconds to respond before the next trial was presented; the intertrial interval of 2 seconds (from onset of the first tone to onset of the subsequent tone) was fixed. Failure to respond was scored as an error. Response time was calculated from the onset of the stimulus to when the button was pressed.
During the active control task (sensory motor control condition), subjects heard the same sets of tones that were presented during the decision task condition. However, instead of identifying the tones as being of high or lower frequency, they were instructed to simply alternate pressing the right and left hand buttons in synchrony with the tone presentation. During the rest condition, subjects were instructed to lie still and remain quiet. Eyes were open and earphones in place during all scans.
Training
Subjects began training on the decision task 1–5 weeks before the PET scan study. Training occurred in a quiet, isolated room. The purposes of the training were to eliminate stimulus/task novelty, determine for each subject the frequency difference that reliably provided an 80% accuracy performance, and to reduce performance variability within and between subjects. The patients with schizophrenia began training during the haloperidol phase and continued throughout the medication withdrawal period. On average, the comparison subjects participated in 22.1 (SD=7.1) blocks of tone-recognition trials (each block contained 100 trials). The patients with schizophrenia, on average, participated in 29.4 (SD=11.9) blocks of tone trials. The difference was not significant.
During the training, subjects became increasingly accurate in recognizing the two types of stimuli, so we systematically modified the tones presented by decreasing the frequency difference between reference and test tones. In the first training session, the differences in frequency between the reference and test tones ranged from 20 to 700 Hz. If a subject was unable to perform at an accuracy rate of at least 80% at the largest frequency difference (700 Hz) after three training attempts, the subject was dropped from further study. No healthy volunteers were dropped from the study because of performance inadequacies, but one was dropped because of an inability to complete the training schedule. Out of 28 that were tested, 10 of the schizophrenic patient volunteers were unable to participate in the study. Four had begun the training procedure but were not able to continue because of their inability to participate in the full set of training sessions. Six patients attempted to perform the task but were unable to sustain the effort sufficiently well to pass beyond the initial training session.
PET Imaging
PET scans were obtained by using the General Electric 4096+ system, which produces 15 brain image slices at an intrinsic resolution of 6.1 mm in each dimension. The bolus [15O]H2O method (21) was used without arterial blood sampling. Approximately 62 mCi of [15O]H2O were administered on each occasion. Ten to 12 minutes elapsed between scans. The rCBF distribution was measured in each of the three conditions (rest, sensory motor control, and decision) with four scans per condition (12 scans per subject). The order of the scans (rest, sensory motor control, decision) was fixed for all subjects and was consecutively repeated four times. During the rest condition, the subject was inactive, eyes were open, ears were unplugged, and tone stimuli and motor activity were absent, but the earphones were in place. The sensory motor control condition consisted of the subject listening to the same tones presented during the decision condition and alternately pressing the right and left buttons in synchrony with the tones. Subjects were asked to respond as soon as they heard the tone. The decision condition consisted of the subject pressing the right button when the high frequency reference tone (1500 Hz) was recognized and the left button when a lower frequency tone was detected. Within a given scan, the frequencies of the two tones were held constant. The frequencies fell within the range in which that individual performed at an 80% accuracy level during the training session. Sixty trials were delivered during each scan; tones began 15 seconds before tracer delivery, and scan acquisitions began 20 seconds after dose delivery. PET data were acquired for 60 seconds.
Image Analysis
The quantitative PET blood flow images were analyzed with statistical parametric mapping (22). The scans from each subject were realigned by using the first as a reference. To optimize the spatial correlation between data sets, the alignment procedure was modified to use 10 iterations (the default procedure uses three iterations). After realignment, all images were transformed into a standard anatomical space (23). Before generating the statistical parametric map, the data were smoothed by using a 12-mm gaussian kernel. Only clusters that contained voxels that were connected (face or edge) and above a threshold (z=2.33, p=0.01, one-tailed, uncorrected for multiple comparisons) were tested by means of spatial extent statistical theory for significance, which was set at an alpha level of <0.05 after correcting for multiple comparisons (22). The full width at half maximum of the average noise subtraction image was used to determine the number of resels (24) available for analysis. The patients with schizophrenia and the comparison subjects were compared for changes in rCBF following shifts in auditory recognition task conditions: sensory motor control versus rest and sensory motor control versus decision. Increases and decreases were assessed. The spatial/anatomical distribution and extent of each cluster were mapped onto matching magnetic resonance images aligned and warped to “fit” statistical data.
Correlation maps to illustrate the linear relationships between response time and regional blood flow were calculated by using the response times of correct choices made during decision scans. Subjects scored about 70%–80% accuracy on each decision block of 60 trials; this provided 42–48 trials, on average, for each block for each subject performing each decision PET scan. With these rCBF-versus-response-time correlation maps, we then contrasted groups voxel-by-voxel by transforming correlation maps to z maps. Difference maps were generated showing where the two groups (comparison subjects and the patients with schizophrenia) significantly differed in their rCBF and response time correlations. The statistical significance of clusters was assessed by spatial extent criteria.
Behavioral outcome measures were extracted from all trials generated in sensory motor control and decision PET scan sessions. These measures are mean accuracy and median response time for accurate trials. The median was used to represent the response time in order to minimize the effect of outliers. Accurate trials were used because inaccurate trials included trials in which no response was made.
Results
Task Performance
For the tone recognition task (decision condition), the patients with schizophrenia provided accuracy scores and response times for accurate trials that were similar to those of the comparison subjects (schizophrenic patients: accuracy=75.6% [SD=10.0], response time: 788.0 msec [SD=169.4]; comparison subjects: accuracy=80.6% [SD=4.7], response time: 735.0 msec [SD=102.0]). However, the frequency disparity (i.e., the difference in frequency required to elicit 80% accuracy in recognizing whether a given tone was lower than the 1500 Hz reference tone) was significantly higher for the patients with schizophrenia (mean=195.8 Hz, SD=265.0) than for the comparison subjects (mean=17.2 Hz, SD=8.3) (t=2.32, df=28, p=0.03, two-tailed with unequal variances). There was no significant correlation between clinical ratings and performance on the tone-recognition task, and variations in medication withdrawal times did not contribute to task performance in any systematic way.
Changes in rCBF Activity Following Shifts in Task Conditions
The auditory recognition tasks produced increasing demands on the subjects’ attention and memory resources. Subtraction analyses for the rest and sensory motor control conditions reveal the shift from an inactive state to a simple repetitive motor condition requiring minimal mnemonic demands. Subtraction analyses for the decision and sensory motor control conditions provide brain activity maps of the switch to an active state requiring a high degree of attention and memory from one that only required repetitive motor behavior and a fixed sensory state.
Sensory motor control versus rest
For the comparison subjects, the subtraction analysis of sensory control minus rest reflected the involvement of primary auditory, motor, and low demand attention systems (Figure 1 [group A], Table 1). Bilateral auditory cortex, right and left inferior frontal cortices, left sensory motor cortex, and the cerebellum were significantly activated by this task in the comparison subjects. For the subtraction analysis of rest minus sensory motor control, greater blood flow was observed in midline structures, precuneus, and medial dorsal frontal cortex (Figure 1 [group B], Table 1). Unexpectedly, greater activity during rest was also observed bilaterally in the posterior middle temporal lobe, but only the right cluster was statistically significant.
For the patients with schizophrenia, the sensory control minus rest analysis revealed robust changes in the left and right sensory motor cortex associated with the hand; face and mouth regions were activated on the left (Figure 2 [group A], Table 2). Anterior cingulate and bilateral auditory cortex areas show marked activity enhancement during the alternating hand motor task cued by repeating tones. When sensory motor control was subtracted from the rest condition, the patients with schizophrenia exhibited a pattern similar to that found in the comparison subjects. Table 2 and Figure 2 [group B] list and illustrate the local maxima in these occipital, visual function regions.
Decision versus sensory motor control
When making tone frequency recognitions (decision condition), the comparison subjects displayed longer response times than they did in the sensory motor control condition. This change in behavior is reflected in the greater activation of inferior/middle frontal cortex, anterior cingulate, and cerebellar cortices during recognition than was seen during the sensory motor control state (Figure 1 [group C], Table 1). Only the left middle temporal lobe exhibited greater flow during the sensory motor control than during the decision condition (Figure 1 [group D], Table 1). This focus in the left middle temporal region mirrored the activation differences found in the rest minus sensory motor control analysis.
The patients with schizophrenia exhibited a smaller rCBF change in the anterior cingulate than did the comparison subjects. Changes found in the left inferior frontal gyrus, left insula, and cerebellum of the comparison subjects were absent in the patients with schizophrenia, but patients with schizophrenia exhibited a significant positive rCBF response in the right inferior parietal lobule (Figure 2 [group C], Table 2). Right frontal activation was robust in both the schizophrenic patients and the comparison subjects.
In the patients with schizophrenia, the sensory motor control minus decision analysis generated a change cluster in the same location as that found in the comparison subjects: the left middle temporal gyrus (Talairach/Tournoux [x, y, z] coordinates: –40, –68, 16; z score of maximum value voxel within cluster=4.66). This was not significant according to spatial extent criteria when corrected for multiple comparisons (Figure 2, group D).
Group-by-condition contrasts
In the decision minus sensory motor control analysis, the comparison subjects exhibited a greater rCBF change than the patients with schizophrenia in the anterior cingulate cortex and supplementary motor area (Figure 3). No other regional differences were found between these two groups in association with any other group-by-condition contrast.
Frequency Disparity
The extremely large frequency range (20 to 700 Hz) required to detect differences in tones with 80% accuracy for the 18 patients with schizophrenia prompted post hoc evaluations of potential behavioral and physiological subgroup patterns. One subgroup of patients with schizophrenia (N=12) were able to score 80% accuracy with tones that fell in the same range (20–100 Hz) as the comparison subjects during the initial testing (mean=36.0 Hz, SD=21.2); these subjects were classified as having “normal frequency disparity.” A second patient group (N=6) required differences greater than 100 Hz to score 80% accuracy (mean=515.3 Hz, SD=232.6) and were classified as having “abnormal frequency disparity.” A one-way analysis of variance used to test for group differences was significant (F=56.14, df=2, 27, p=0.0001). Tukey’s post hoc test showed differences between patients with normal and abnormal frequency disparity at an alpha level of 0.05. The accuracy scores and response times for accurate trials were similar for patients with normal and abnormal frequency disparity (normal disparity: accuracy=78.4% [SD=4.7], response time: 778.4 msec [SD=191.1]; abnormal disparity: accuracy=70.0% [SD=15.5], response time: 807.1 msec [SD=128.7]).
Anterior cingulate cortex rCBF activity was equivalent in the comparison subjects and schizophrenic patients with normal frequency disparity during the sensory motor control condition, but it changed differentially during the decision condition (Table 3). rCBF rose in the comparison subjects, fell in the schizophrenic patients with abnormal frequency disparity, and exhibited a small positive change in schizophrenic patients with normal frequency disparity. The decision minus sensory motor control analysis showed that the cingulate response of the schizophrenic patients with abnormal frequency disparity was significantly lower than that of both the comparison subjects (z score of maximum value voxel within cluster=5.47, p<0.0001, by spatial extent) and the schizophrenic patients with normal frequency disparity (z score of maximum value voxel within cluster=4.32, p<0.05, by spatial extent). In contrast, the schizophrenic patients with normal frequency disparity did not differ significantly from the comparison subjects in the cingulate or any other region.
Figure 4 vividly illustrates this pattern by plotting repeat fluctuations in rCBF at an area in the cingulate cortex chosen to represent the optimal cingulate change scores of all three groups. The comparison subjects exhibited an abrupt positive rCBF change when switching from the sensory motor control to the decision task. The rCBF of schizophrenic patients with normal frequency disparity tended to rise from rest to sensory motor control and continue rising during decision. Schizophrenic patients with abnormal frequency disparity exhibited no consistent cingulate change pattern.
Anterior cingulate rCBF differences between comparison subjects and schizophrenic patients with abnormal frequency disparity were expected because of the initial finding between the comparison subjects and the entire group of patients with schizophrenia. Statistical parametric mapping (22) was used to analyze the entire cortex of these two groups (Table 4 and Figure 4). In addition to the anterior cingulate, the right premotor cortex and left motor cortex also exhibited high, significant change scores. No differences were found between the comparison subjects and the schizophrenic patients with normal frequency disparity in the between-condition contrasts.
Correlations Between rCBF and Response Time
rCBF activation changes in schizophrenic patients with normal frequency disparity were similar to those of the comparison subjects; accuracy performance and stimuli characteristics were matched across groups. The changes that accompanied the switch from sensory motor control to decision were similar in the two groups. Within the comparison group, clear and physiologically meaningful relationships appeared between the average response time associated with accurate tone recognitions and the blood flow to a particular brain region (25). These response time-rCBF correlation maps were generated for the comparison subjects and the schizophrenic patients with normal frequency disparity by using blood flow and behavioral data acquired during decision condition PET scans. The correlation maps were then contrasted between groups. Local maxima were then extracted and plotted to inspect the distributions around the regression functions. On the basis of previous analyses with healthy comparison subjects, the right frontal cortex and right auditory cortex were expected to generate the largest between-group contrasts. The comparison subjects exhibited strong negative correlations between response time and rCBF in auditory regions (i.e., short response times were associated with maximal blood flow rates). Robust positive correlations between response time and rCBF were found in the frontal cortex (i.e., long response times were associated with maximal flow rates).
Table 5 and Figure 6 illustrate how the correlations between response time and rCBF differed between the schizophrenic patients with normal frequency disparity and the comparison subjects even though their brain activation patterns were similar. The difference maps illustrate where the two groups exhibited opposite correlations between response time and rCBF. Significant differences were associated with regions in which each group exhibited a significant, but opposite, correlation between average decision response time and rCBF measured during a particular set of decisions. Each subject contributed four decision scans to the analysis.
The comparison subjects exhibited progressively greater flow rates in the frontal cortex the longer the subject took to make an accurate response. In marked contrast, the longer the schizophrenic patients with normal frequency disparity waited to make an accurate response, the lower the flow rate to the frontal cortex. The comparison subjects displayed maximum flow rates to the right auditory cortex in association with short response times; the schizophrenic patients with normal frequency disparity exhibited the opposite pattern in this region.
Discussion
After extensive training on a tone recognition task, a subgroup of medication-withdrawn volunteers with schizophrenia was able to perform, on average, as well as a group of healthy volunteers. It is, however, important to note that some of the schizophrenic patient volunteers were not able to participate in this study; their exclusion necessarily tempers the applicability of these findings to the general population of patients with schizophrenia. The schizophrenic participants exhibited only one significant difference from the comparison subjects in their brain blood flow patterns. The comparison subjects exhibited a greater increase than the schizophrenic patients in neural activity in the anterior cingulate cortex and supplementary motor areas when switching from the sensory motor control to the decision condition. When those volunteers with schizophrenia who were able to use “normal” tone stimuli were compared with healthy volunteers, they showed no differences in rCBF activation, but they differed profoundly in their correlation profiles. Whereas the comparison subjects exhibited progressively greater blood flow to the frontal cortex in association with longer response times, the patients with schizophrenia exhibited progressively lower blood flow in conjunction with extended response times. The schizophrenic patients with normal frequency disparity did not, however, fail to exhibit enhanced frontal blood flow when challenged by this high-error auditory memory task. These data suggest that 1) schizophrenic patient volunteers are significantly restricted in their capacity to modulate brain activity in association with task demand; and 2) in conjunction with the need to suppress premature and incorrect responses, patients with schizophrenia exhibit progressive failure of frontal blood flow activation instead of progressive enhancement.
Behavioral Findings
Most patients were able to distinguish and recognize tones that differed from one another by small frequency levels (about 2%). However, one-third of the schizophrenic patients required tone frequency differences of 10%–50% in order to perform the task at accuracy levels above chance (greater than 65%). This finding is consistent with studies reported by Javitt and colleagues (16). It is important to note that the schizophrenic patients with abnormal frequency disparity performed this task at accuracy levels and response times close to those found in the comparison subjects and the schizophrenic patients with normal frequency disparity. Frequency differences seem to be especially important vulnerabilities for patients with schizophrenia (16, 26). The inability to quickly and accurately ascertain one frequency from another may contribute to speech and language perceptual difficulties commonly ascribed to some schizophrenic patients. In particular, the inability to “understand” the pitch fluctuations of words may contribute to a patient’s social mistakes and contribute to his or her social isolation. If a patient cannot easily detect and track changes in the pitch of a word, he or she may be severely disadvantaged in the ability to understand how the word is being used in a particular social setting. Similarly, patients may exhibit a more restricted affective range in their speech if they are unable to “hear” subtle pitch modulations.
Blood Flow Patterns
When attending to tones, but not performing a difficult recognition task, patients with schizophrenia activated their auditory cortices, right and left, similar to the comparison subjects. These findings suggest that patients are able to make simple auditory perceptions by using a neural substrate that is not significantly different from that of comparison subjects. Javitt et al. (16) and Holcomb et al. (26) have reported behavioral studies emphasizing the vulnerability to auditory memory of patients with schizophrenia. Shenton et al. (27) and Menon et al. (28) have described strong correlations between psychotic behavior and auditory cortex structures in patients with schizophrenia. The findings reported here suggest the deficits and abnormalities of patients with schizophrenia may not reside in a primary sensory processing site; they may instead be more closely linked to dynamic relationships within the association and heteromodal areas.
The contrast between the sensory motor control and rest conditions in the patients with schizophrenia revealed a striking change in anterior cingulate/supplementary motor area activity. Figure 1 and Figure 3 demonstrate a high level of activity change in conjunction with the simple, repetitive task of pressing alternate buttons that was specific to the motor control condition. The subjects’ rapid responses were consistent with their practiced condition. Work by Carter et al. (29) suggested that cingulate activity is greatest when a subject is performing a task associated with significant error likelihood. However, patients with schizophrenia may not follow this “error context” rule, at least not as it is understood in the normal population. Patients with schizophrenia may be abnormally sensitive to “set maintenance.” When any repetitive task involving side-to-side alternation is engaged, this group may need to use maximum frontal, cingulate, and supplementary motor area activity. This may reflect the group’s difficulty in assessing error likelihood or suppressing distractions. The patients with schizophrenia may “expect” or anticipate greater error than is actually likely to occur, and they may not be able to modulate activity with respect to the likelihood of error. Low-error likelihoods may be treated by the frontocingulate system the same as high-error conditions in volunteer patients with schizophrenia. This may partially explain why no additional change was found in the cingulate cortex/supplementary motor area region when volunteer patients with schizophrenia shifted from the sensory motor control to the decision condition. That behavioral switch did, however, result in a marked activation in the right frontal cortex of patients with schizophrenia. The cingulate/supplementary motor area system may be working optimally in schizophrenic patient volunteers when they are confronted with simple tasks associated with alternate choices; this system may, however, contain fundamental flaws in its dynamic relationships with the frontal lobe (3, 30–34).
Increased frontal rCBF found in the schizophrenic patients and the comparison subjects with the decision minus sensory motor control analysis suggest that the additional demands characteristic of the decision condition are partly represented and mediated by frontal lobe activity. Decision trials are slower and associated with higher error rates than sensory motor control trials. It is not possible to listen to a tone trial in isolation and accurately identify it as high or low frequency. Thus, the decision condition is sufficiently difficult to require a participant to keep in mind what the previous stimuli sounded like. The schizophrenic patient volunteers were probably using frontal regions similar to the comparison subjects for this task. By supplying information about recent events and making rapid comparisons, the frontal regions facilitate subtle perceptual judgments. Over brief time intervals, the frontal lobes of patients with schizophrenia and comparison subjects may function in similar ways. This interval, however, may last no more than about 600 msec; beyond that time the two groups may use substantially different strategies for problem solving.
Frequency Disparity Differences in rCBF Activity
The schizophrenic patients with abnormal frequency disparity exhibited no significant cingulate/supplementary motor area response to changing behavioral demands (Figure 4). Unable to perform tone recognition at low-disparity frequencies, this group may have perceptual abnormalities that are due to severely impaired attention and memory resources. This subgroup of patients with schizophrenia exhibited significant differences from the healthy comparison subjects and schizophrenic patients with normal frequency disparity in motor and premotor regions; this suggests that the circuits associated with stimulus choice, impulse inhibition, and set maintenance are potentially defective. Not clinically distinct, the six schizophrenic patient volunteers with abnormal frequency disparity were not remarkable with respect to symptoms or course of illness. Furthermore, this group of six volunteers showed no capacity to improve in their auditory memory performance in spite of extensive training; the other 12 schizophrenic patient volunteers significantly improved in tone recognition over the training period (31). Unable to use the frontal, cingulate, and supplementary motor area cortices to manipulate incoming information quickly, this subgroup may have relied more heavily on secondary auditory cortex and motor regions for sensory integration and recent stimulus representation. This group’s largest change clusters (seen in the sensory motor control minus rest analyses) were found in the left auditory and motor/hand regions, not in the association cortex. Although distinctly different from the schizophrenic patients with normal frequency disparity in this study, this group may appear more continuous with the other patients with schizophrenia in tasks requiring a different set of cognitive resources.
Correlations Between Response Time and rCBF: How the Frontal Lobe Fails
The blood flow contrast patterns (decision minus sensory motor control) between the comparison subjects and schizophrenic patients with normal frequency disparity were not significantly different in the frontal lobes. Yet, it is widely appreciated that schizophrenic patient volunteers perform particularly poorly on working memory and long-term memory tasks, which are highly dependent on frontal lobe function. The comparison subjects exhibited a marked tendency to have increased rCBF in the frontal lobes that was associated with progressively longer response times. During the first 300–500 msec of a trial, the comparison subjects and patients with schizophrenia may have relied primarily on the auditory cortex for making a match between the percept and its correct representation. Behavioral data (15, 25) suggest that healthy subjects become less and less accurate the longer they suppress a response. Responses made 1500 msec after stimulus presentation are far less likely to be accurate than those made at 400 msec. Response inhibition may be associated with a search for the correct match in the association cortex. Then, the longer the response is suppressed, the noisier becomes the percept, and the less accurate becomes the decision. The response of the comparison subjects to prolonged (greater than 1,000 msec) response inhibition was to facilitate greater activation of the frontal lobe; the response to prolonged response inhibition of patients with schizophrenia was to enhance primary auditory cortex rCBF. Lesion studies (18, 19) have suggested that frontal lobe pathology, especially the right side, strongly compromises auditory memory. When patients with schizophrenia fail to execute a decision after 1 second they may not continue to search for the correct match in the frontal association cortex; they may be required to rely on the primary auditory cortex, a site less equipped to provide accurate long-delay matches. Although attentive to the task, the group of patients with schizophrenia may be unable to engage the optimal search strategy. Attention is not sufficient for successful recognition when searching for ambiguous signals.
This model is consistent with work by investigators who have studied patients with schizophrenia making memory decisions (32). When asked to make automatic decisions, the group of patients with schizophrenia often appeared similar to the comparison subjects. When, however, a time delay is imposed (e.g., 2-Back Working Memory Task) that exceeds 1 second, then marked differences appear between groups physiologically and behaviorally. Blood flow apparently diminishes in frontal lobes of patients with schizophrenia attempting to make long memory decisions; their accuracy also tends to drop precipitously (16). These studies support a strong time component for the frontal lobe failure of patients with schizophrenia. As long as the schizophrenic patient volunteer is able to make most of his or her decisions quickly (e.g., under 800 msec), frontal lobe activity is likely to appear similar to that of comparison subjects. When, however, patients with schizophrenia are either compelled to wait, or choose to wait, for over 1,200 msec, then they are likely to exhibit marked differences in frontal flow from that of comparison subjects.
When the identity of a stimulus becomes extremely ambiguous, schizophrenic patient volunteers became markedly impaired in their recognition abilities. This may be partly due to the increased time needed to search out and confirm an accurate match. The longer the search is maintained, the less available is the frontal lobe. Consequently, the group of patients with schizophrenia will exhibit progressive failure with greater time delays whether the information is either 1) unambiguous and the motor response delay is externally enforced or 2) it requires an extended internal search and greater time delays because of the subject’s uncertainty. In either case, schizophrenic patient volunteers appear unable to use the frontal lobe to sustain a search over a prolonged time period. The cingulate cortex may play an important role in modulating the frontal lobe’s activities (30). If the cingulate cannot shift the frontal lobe’s state from one level of activity to another, then the frontal lobe may prematurely terminate or diminish its probe for the correct answer (31, 33, 34).
Future studies that use single-event functional MRI methods may be better suited to untangle these relationships. Schizophrenic patient volunteers engaged in a task similar to one performed by comparison subjects exhibited similar activity change patterns. They did, however, exhibit exactly opposite blood flow/response time correlations in sensory cortex and frontal lobes. Functional MRI may be able to assess to what extent the cingulate/supplementary motor area region becomes “disconnected” from the frontal lobe during responses requiring particularly long time intervals.
![]() |
![]() |
![]() |
![]() |
![]() |
Received June 18, 1999; revisions received Feb. 29 and April 10, 2000; accepted April 20, 2000. From the Maryland Psychiatric Research Center and the Nuclear Medicine Division, Department of Radiology, Johns Hopkins Medical Institutes, Baltimore. Address reprint requests to Dr. Holcomb, Maryland Psychiatric Research Center, University of Maryland at Baltimore, P.O. Box 21247, Baltimore, MD 21228; [email protected] (e-mail).Supported by grants from NIMH (MH-44211, MH-40279, and MH-42234) and the William K. Warren Foundation.The authors thank Jeff Sieracki for technical support with the tone recognition software; Ms. Ning Ma and Zuo Zhao for computer image analyses; Dr. Haydn Ravert, Robert Smoot, and David Clough for PET support; Pamela Caudill for tone recognition training for the schizophrenic and comparison participants; Dale Warfel, Tamara Michaelidis, and the nursing staff of the Residential Research Unit for providing clinical care; Drs. Milton Strauss, June Corwin, and Anne Summerfelt for the theoretical background to this project; and Ms. Susan Basham and Ms. Carlette Daniels for administrative assistance.
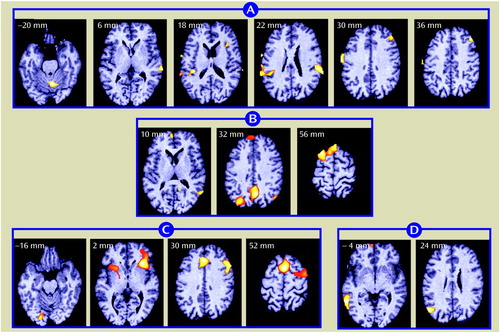
Figure 1. Changes in rCBF in 12 Healthy Comparison Subjects Following Shifts in Auditory Recognition Task Conditionsa
aImages show areas of maximum change. The change clusters extended beyond the anatomical planes containing the maxima; additional images were included to illustrate spatial spread. The right side of each image is the true right side of the brain. The numbers attached to each image indicate anatomical location (i.e., the number of millimeters above or below the bicommissural reference plane as represented in the Talairach and Tournoux atlas [23]). Changes in rCBF between an inactive state (rest condition) to one requiring minimal attention and memory (sensory motor control condition)—determined through subtraction analyses—are depicted in groups A (sensory motor control minus rest) and B (rest minus sensory motor control). rCBF changes occurring between the sensory motor control condition and one requiring a high degree of attention and memory (decision condition) are depicted in groups C (decision minus sensory motor control) and D (sensory motor control minus decision). Activations occurred in the midline cerebellum, primary auditory cortex, and right frontal cortex (group A); in the occipital and posterior cingulate cortex and superior frontal gyrus (group B); and in the insula/claustrum (bilaterally), right inferior frontal cortex, cingulate and supplementary motor cortices, and right premotor cortex (group C). In group D, activations were restricted to the left middle temporal gyrus.
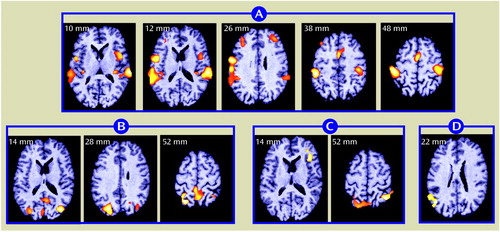
Figure 2. Changes in rCBF in 18 Schizophrenic Patient Volunteers Following Shifts in Auditory Recognition Task Conditionsa
aFor anatomical locations and information on image groupings, see Figure 1 footnote. In group A, bilateral activations occurred in primary and secondary auditory cortices and the sensorimotor cortex. Changes also occurred in the cingulate and supplementary motor areas. In group B, activations occurred in the posterior cingulate and occipital cortices. In group C, the activation pattern included the right inferior frontal cortex and the parietal cortex, right side. In group D, activation was restricted to the left middle temporal gyrus.
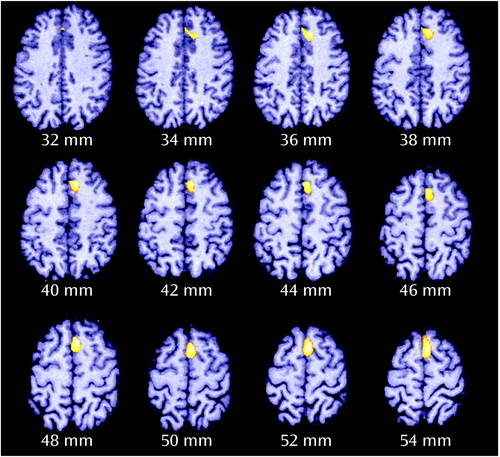
Figure 3. Differences in rCBF Changes Between 12 Healthy Comparison Subjects and 18 Schizophrenic Patient Volunteers Following Shifts in Auditory Recognition Task Conditionsa
aFor definitions of task conditions, see Figure 1 footnote. The comparison subjects exhibited greater rCBF changes in the anterior cingulate cortex (extending from a plane 32 mm above the bicommissural axis, through the supplementary motor area, to a level 54 mm above the reference axis), as determined by subtraction analysis (decision minus sensory motor control; z score of maximum value voxel within cluster=3.88, p<0.06)
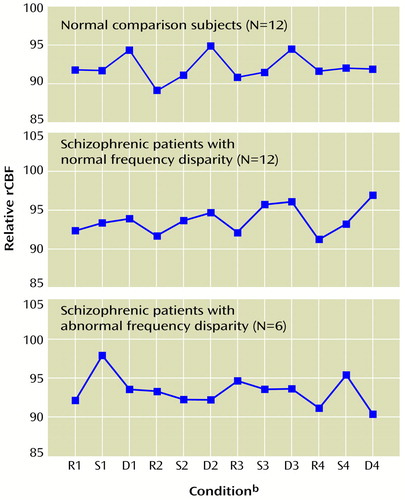
Figure 4. Fluctuations in Cingulate/Supplementary Motor Area rCBF During Auditory Recognition Task Conditions for 12 Healthy Comparison Subjects and 18 Schizophrenic Patient Volunteers With Normal and Abnormal Frequency Disparitya
aFor definitions of task conditions, see Figure 1 footnote. Frequency disparity was the difference between auditory tones required to achieve 80% accuracy in distinguishing between tones of lower and higher frequency in the decision condition. Local blood flow fluctuations occurring in the cingulate/supplementary motor area region were tightly coupled with the decision condition in comparison subjects. The schizophrenic patients with normal frequency disparity exhibited elevations during the sensory motor control and decision conditions. The schizophrenic patients with abnormal frequency disparity displayed no consistent, task-related fluctuations.
bR=rest, S=sensory motor control, D=decision.
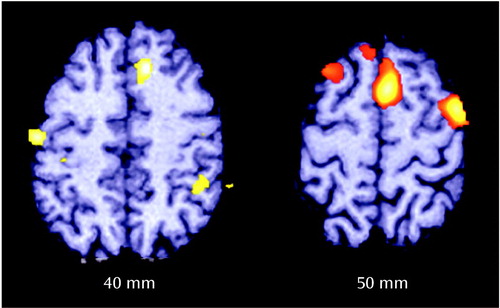
Figure 5. Differences in rCBF Changes Following Shifts in Auditory Recognition Task Conditions Between 12 Healthy Comparison Subjects and Six Schizophrenic Patient Volunteers With Abnormal Frequency Disparitya
aFor definitions of task conditions, see Figure 1 footnote. Frequency disparity was the difference between auditory tones required to achieve 80% accuracy in distinguishing between tones of lower and higher frequency in the decision condition. The comparison subjects exhibited greater rCBF changes in the anterior cingulate, supplementary motor area, right premotor cortex, and right sensory motor regions, as determined by subtraction analysis (decision minus sensory motor control).
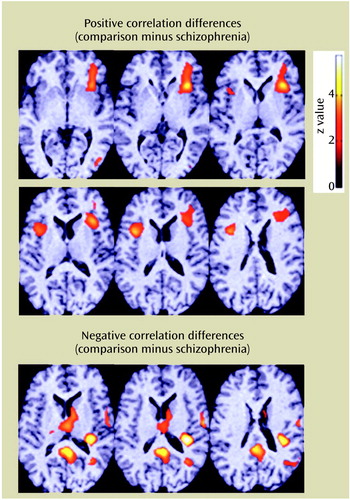
Figure 6. Differences in Correlations Between Response Time and rCBF for 12 Healthy Comparison Subjects and 12 Schizophrenic Patient Volunteers With Normal Frequency Disparity in Auditory Recognition Taska
aThe top six images show the areas of the frontal cortex in which the comparison subjects displayed strong positive correlations between blood flow and response times, whereas the patients with schizophrenia exhibited equally strong negative correlations. The bottom three images show the areas of the posterior cingulate, primary auditory cortex, and thalamus in which the comparison subjects exhibited significant negative correlations of blood flow with response time. The schizophrenic patients maintained the opposite correlation pattern: higher blood flow in conjunction with longer response times.
1.. Buchsbaum MS, Wu JC, DeLisi LE, Holcomb HH, Hazlett E, Cooper-Langston K, Kessler R: Positron emission tomography studies of basal ganglia and somatosensory cortex neuroleptic drug effects: differences between normal control subjects and schizophrenic patients. Biol Psychiatry 1987; 22:479–494Crossref, Medline, Google Scholar
2.. Gur RE, Mozley PD, Resnick SM, Mozley LH, Shtasel DL, Gallacher F, Arnold SE, Karp JS, Alavi A, Reivich M, Gur RC: Resting cerebral glucose metabolism in first-episode and previously treated patients with schizophrenia relates to clinical features. Arch Gen Psychiatry 1995; 52:657–667Crossref, Medline, Google Scholar
3.. Tamminga CA, Thaker GK, Buchanan R, Kirkpatrick B, Alphs LD, Chase TN, Carpenter WT: Limbic system abnormalities identified in schizophrenia using positron emission tomography with fluorodeoxyglucose and neocortical alterations with deficit syndrome. Arch Gen Psychiatry 1992; 49:522–530Crossref, Medline, Google Scholar
4.. Steinberg JL, Devous MD Sr, Paulman RG, Gregory RR: Regional cerebral blood flow in first break and chronic schizophrenic patients and normal control subjects. Schizophr Res 1995; 17:229–240Crossref, Medline, Google Scholar
5.. Paulman RG, Devous MD Sr, Gregory RR, Herman JH, Jennings L, Bonte FJ, Nasrallah HA, Raese JD: Hypofrontality and cognitive impairment in schizophrenia: dynamic single-photon tomography and neuropsychological assessment of schizophrenic brain function. Biol Psychiatry 1990; 27:377–399Crossref, Medline, Google Scholar
6.. Weinberger DR, Berman KF, Suddath R, Torrey EF: Evidence of dysfunction of a prefrontal-limbic network in schizophrenia: a magnetic resonance imaging and regional cerebral blood flow study of discordant monozygotic twins. Am J Psychiatry 1992; 149:890–897Link, Google Scholar
7.. Berman KF, Torrey EF, Daniel DG, Weinberger DR: Regional cerebral blood flow in monozygotic twins discordant and concordant for schizophrenia. Arch Gen Psychiatry 1992; 49:927–934Crossref, Medline, Google Scholar
8.. Andreasen NC, Rezai K, Alliger R, Swayze VW II, Flaum M, Kirchner P, Cohen G, O’Leary DS: Hypofrontality in neuroleptic-naive patients and in patients with chronic schizophrenia: assessment with Xenon 133 single-photon emission computed tomography and the Tower of London. Arch Gen Psychiatry 1992; 49:943–958Crossref, Medline, Google Scholar
9.. Gur RE, Resnick SM, Alavi A, Gur RC, Caroff S, Dann R, Silver FL, Saykin AJ, Chawluk JB, Kushner M, Reivich M: Regional brain function in schizophrenia. Arch Gen Psychiatry 1987; 44:119–125Crossref, Medline, Google Scholar
10.. Holcomb HH, Cascella NG, Medoff DR, Gastineau EA, Loats H, Thaker GK, Conley RR, Dannals RF, Wagner HN Jr, Tamminga CA: PET-FDG test-retest reliability during a visual discrimination task in schizophrenia. J Comput Assist Tomogr 1993; 17:704–709Crossref, Medline, Google Scholar
11.. Holcomb HH, Medoff DR, Lahti AC, Tamminga CA: Functional neuroimaging in schizophrenia: symptoms, treatment, and etiology, in Schizophrenia in a Molecular Age. Edited by Tamminga CA. Washington, DC, American Psychiatric Press, 1999, pp 77–107Google Scholar
12.. Blamire AM, Ogawa S, Ugurbil K, Rothman D, McCarthy G, Ellermann JM, Hyder F, Rattner Z, Shulman RG: Dynamic mapping of the human visual cortex by high-speed magnetic resonance imaging. Proc Natl Acad Sci USA 1992; 89:1069–1073Google Scholar
13.. Volkow ND, Wolf AP, Van Gelder P, Brodie JD, Overall JE, Cancro R, Gomez-Mont F: Phenomenological correlates of metabolic activity in 18 patients with chronic schizophrenia. Am J Psychiatry 1987; 144:151–158Link, Google Scholar
14.. Buchsbaum MS, Nuechterlein KG, Haier RJ, Wu J, Sicotte N, Hazlett E, Asarnow R, Potkin SG, Guich S: Glucose metabolic rate in normals and schizophrenics during the continuous performance test assessed by positron emission tomography. Br J Psychiatry 1990; 156:216–227Crossref, Medline, Google Scholar
15.. Holcomb HH, Cascella NG, Thaker GK, Medoff DR, Dannals RF, Tamminga CA: Functional sites of neuroleptic drug action in the human brain: PET/FDG studies with and without haloperidol. Am J Psychiatry 1996; 153:41–49Link, Google Scholar
16.. Javitt DC, Strous RD, Grochowski S, Ritter W, Cowan N: Impaired precision, but normal retention, of auditory sensory (“echoic”) memory information in schizophrenia. J Abnorm Psychol 1997; 106:315–324Crossref, Medline, Google Scholar
17.. Urbach D, Spitzer H: Attentional effort modulated by task difficulty. Vision Res 1995; 35:2169–2177Google Scholar
18.. Samson S, Zatorre RJ: Learning and retention of melodic and verbal information after unilateral temporal lobectomy. Neuropsychologia 1992; 30:815–826Crossref, Medline, Google Scholar
19.. Samson S, Zatorre RJ: Contribution of the right temporal lobe to musical timbre discrimination. Neuropsychologia 1994; 32:231–240Crossref, Medline, Google Scholar
20.. Overall JE, Gorham DR: The Brief Psychiatric Rating Scale. Psychol Rep 1962; 10:799–812Crossref, Google Scholar
21.. Raichle ME, Martin WR, Herseovitch P, Mintun MA, Markham J: Brain blood flow measured with intravenous H215O.11: implementation and validation. J Nucl Med 1983; 24:790–798Medline, Google Scholar
22.. Friston KJ, Holmes A, Poline JB, Price CJ, Frith CD: Detecting activations in PET and fMRI: levels of inference and power. Neuroimage 1996; 4(3, part 1):223–235Google Scholar
23.. Talairach J, Tournoux P: Co-Planar Stereotaxic Atlas of the Human Brain: Three-Dimensional Proportional System. Stuttgart, Germany, Georg Thieme, 1988Google Scholar
24.. Worsley KJ, Evans AC, Marrett S, Neelin P: A three-dimensional statistical analysis for CBF activation studies in human brain. J Cereb Blood Flow Metab 1992; 12:900–918Crossref, Medline, Google Scholar
25.. Holcomb HH, Medoff DR, Caudill PJ, Zhao Z, Lahti AC, Dannals RF, Tamminga CA: Cerebral blood flow relationships associated with a difficult tone recognition task in trained normal volunteers. Cereb Cortex 1998; 8:534–542Crossref, Medline, Google Scholar
26.. Holcomb HH, Ritzl EK, Medoff DR, Nevitt J, Gordon B, Tamminga CA: Tone discrimination performance in schizophrenic patients and normal volunteers: impact of stimulus presentation levels and frequency differences. Psychiatry Res 1995; 57:75–82Crossref, Medline, Google Scholar
27.. Shenton ME, Kikinis R, Jolesz FA, Pollak SD, LeMay M, Wible CG, Hokama H, Martin J, Metcalf D, Coleman M: Abnormalities of the left temporal lobe and thought disorder in schizophrenia: a quantitative magnetic resonance imaging study. N Engl J Med 1992; 327:604–612Crossref, Medline, Google Scholar
28.. Menon RR, Barta PE, Aylward EH, Richards SS, Vaughn DD, Tien AY, Harris GJ, Pearlson GD: Posterior superior temporal gyrus in schizophrenia: grey matter changes and clinical correlates. Schizophr Res 1995; 16:127–135Crossref, Medline, Google Scholar
29.. Carter CS, Mintun M, Nichols T, Cohen JD: Anterior cingulate gyrus dysfunction and selective attention deficits in schizophrenia: [15O]H2O PET study during single-trial Stroop task performance. Am J Psychiatry 1997; 154:1670–1675Google Scholar
30.. Tamminga CA, Thaker GK, Buchanan R, Kirkpatrick B, Alphs LD, Chase TN, Carpenter WT: Limbic system abnormalities identified in schizophrenia using positron emission tomography with fluorodeoxyglucose and neocortical alterations with deficit syndrome. Arch Gen Psychiatry 1992; 49:522–530Crossref, Medline, Google Scholar
31.. Kakoyonnis A, Frey K, Weiler M, Medoff DR, Lahti AC, Holcomb HH, Tamminga CA: Response to training, do patients with schizophrenia differ from healthy control group subjects in their response to auditory discrimination training? (abstract). Schizophr Res 1999; 36:171Google Scholar
32.. Callicott JH, Ramsey NF, Tallent K, Bertolino A, Knable MB, Coppola R, Goldberg T, van Gelderen P, Mattay VS, Frank JA, Moonen CT, Weinberger DR: Functional magnetic resonance imaging brain mapping in psychiatry: methodological issues illustrated in a study of working memory in schizophrenia. Neuropsychopharmacology 1998; 18:186–196Crossref, Medline, Google Scholar
33.. Fletcher P, McKenna PJ, Friston KJ, Frith CD, Dolan RJ: Abnormal cingulate modulation of fronto-temporal connectivity in schizophrenia. Neuroimage 1999; 9:337–342Crossref, Medline, Google Scholar
34.. Haznedar MM, Buchsbaum MS, Luu C, Hazlett EA, Siegel BV Jr, Lohr J, Wu J, Haier RJ, Bunney WE Jr: Decreased anterior cingulate gyrus metabolic rate in schizophrenia. Am J Psychiatry 1997; 154:682–684Link, Google Scholar