Apoptotic Proteins in the Temporal Cortex in Schizophrenia: High Bax/Bcl-2 Ratio Without Caspase-3 Activation
Abstract
OBJECTIVE: Neuroimaging findings have identified lower cortical gray matter volume in schizophrenia. Apoptosis (programmed cell death) has been proposed as a contributing pathophysiological mechanism. Levels of antiapoptotic Bcl-2 protein are low in the temporal cortex of schizophrenia patients. Bcl-2 interacts with the proapoptotic Bax protein at an upstream checkpoint to regulate the activation of apoptosis by caspase-3 and other proteolytic caspase proteins. A high Bax/Bcl-2 ratio is associated with greater vulnerability to apoptotic activation, while a high caspase-3 level is often associated with apoptotic activity. It was hypothesized that the Bax/Bcl-2 ratio, but not caspase-3, would be high in the temporal cortex of patients with chronic schizophrenia. METHOD: Bax, Bcl-2, and caspase-3 proteins were measured by semiquantitative Western blot in Brodmann’s area 21 (middle temporal gyrus) of postmortem tissue from patients with schizophrenia (N=15), bipolar disorder (N=15), or major depression (N=15) and nonpsychiatric comparison subjects (N=15). RESULTS: The Bax/Bcl-2 ratio was 50% higher in the schizophrenia patients than the nonpsychiatric comparison subjects. The level of caspase-3 (inactive zymogen and activated subunits) was not significantly different. CONCLUSIONS: The higher Bax/Bcl-2 ratio suggests that cortical cells are vulnerable to apoptosis in chronic schizophrenia. However, the normal caspase-3 level suggests that apoptosis is not active in this illness phase. Furthermore, the results appear to distinguish the pathophysiology of schizophrenia from most classic neurodegenerative disorders, in which postmortem caspase-3 levels are high. Further studies are needed to investigate the implications of abnormal apoptotic proteins in schizophrenia.
Neuronal apoptosis is highly active during early neurodevelopment. It is estimated that, depending on the region, 20%–80% of all neurons formed in the CNS die by apoptosis in early life (1). Apoptosis is regulated by several protein families, including the upstream Bcl-2 family (e.g., the antiapoptotic Bcl-2 and proapoptotic Bax) and the downstream caspase family (e.g., caspase-3). Pathological activation of neuronal apoptosis and abnormal expression of apoptotic regulatory proteins have been demonstrated in classic neurodegenerative disorders, including Alzheimer’s and Parkinson’s diseases (2–5). Apoptosis has also been hypothesized to contribute to the pathophysiology of schizophrenia (6), a neurodevelopmental disorder that may encompass limited neurodegenerative features (7). Postmortem evidence supports apoptotic involvement in schizophrenia; low levels of Bcl-2 have been demonstrated in Brodmann’s area 21 (middle temporal gyrus) (8). It is interesting that this cortical area is also associated with low gray matter volume (9), similar to low volumes in other temporal and frontal areas and in total cortical gray matter (10, 11). Low levels of Bcl-2 in Brodmann’s area 21 may signal a role for apoptotic proteins in the mechanisms underlying the low gray matter volumes in schizophrenia.
The activation of apoptosis is controlled at multiple checkpoints within the cell. Upstream, the proapoptotic Bax and antiapoptotic Bcl-2 are membrane-bound pore-forming proteins that interact through heterodimerization. Together they regulate the mitochondrial transmembrane passage of cytochrome c, which in turn activates caspase proteins. The Bax/Bcl-2 ratio appears more important than the individual Bax or Bcl-2 level in determining a cell’s vulnerability to apoptosis; high Bax/Bcl-2 ratios lead to greater apoptotic activity (12). Neuronal apoptosis in the CNS is significantly lower than normal in Bax-deleted mice and in Bcl-2-overexpressing mice, while in Bcl-2-deleted mice and in Bax-overexpressing mice, apoptosis is greater than normal (13). Furthermore, in rat cortical development, the Bax/Bcl-2 ratio is highest during the first 2 postnatal weeks, which corresponds to the most active interval of neurodevelopmental apoptosis, while the Bax/Bcl-2 ratio decreases during normal aging, when neuronal apoptosis is rare (13, 14). A high Bax/Bcl-2 ratio has been demonstrated in the cortex of brains from patients with Down’s syndrome (15) and in human neuronal cultures treated with amyloid β, a model for Alzheimer’s disease (16).
Caspase proteins are cysteine proteases that act downstream of the Bcl-2 family by initiating cellular breakdown during apoptosis (17). Initiator caspases (e.g., caspase-8 and caspase-9) serve to activate effector caspases (e.g., caspase-3, caspase-6, and caspase-7). Among the effector caspases, caspase-3 is most frequently involved in neuronal apoptosis (18). Cleavage of inactive procaspase-3 protein into active caspase-3 subunits is a marker of apoptotic activity (19), and levels of activated caspase-3 subunits are significantly higher than normal in both the cortex of patients with Alzheimer’s disease (4) and the substantia nigra of patients with Parkinson’s disease (5). Thus, measurement of caspase-3 can yield information about ongoing neuronal apoptosis and provide a pathophysiological comparison to classic neurodegeneration.
This is a continuing investigation of apoptotic proteins in the temporal cortex of schizophrenia patients. It has been demonstrated that in these patients the temporal cortex, including Brodmann’s area 21, has a low volume of gray matter (9, 20–22), experiences progressive volume loss in early stages of the illness (23, 24), and is involved in certain clinical manifestations of schizophrenia, including auditory hallucinations (20, 25). Western blot analysis was used to measure Bax, Bcl-2, procaspase-3, and activated caspase-3 subunit levels in blocks of Brodmann’s area 21. The primary hypothesis was that the Bax/Bcl-2 ratio would be high in patients with schizophrenia.
Method
Postmortem Samples
This study was approved by the institutional review board of the University of North Carolina School of Medicine. The postmortem middle temporal gyrus (Brodmann’s area 21) from 60 subjects was obtained from the Stanley Medical Research Institute (Bethesda, Md.); the subjects comprised patients with schizophrenia, bipolar disorder, or major depression and nonpsychiatric comparison subjects (N=15 for each group). Subjects over the age of 68 years were excluded to minimize comorbid neurological disorders. The subjects were group matched for age, gender, ethnicity, side of brain, brain pH, and postmortem interval (Table 1). Demographic and clinical characteristics for individual subjects, including cumulative antipsychotic medication exposure and medications at the time of death, have been extensively detailed elsewhere (8, 26). All samples were stored at –80°C until use. The schizophrenia and nonpsychiatric comparison groups were the primary focus of this study. All data were collected by investigators blind to diagnosis.
Procedure
Tissue homogenization
Tissue (100–300 mg) was placed in 10 volumes of 10 mM HEPES buffer (pH 7.0) with 0.32 M sucrose, 0.1 mM phenylmethylsulfonyl fluoride, 10 μg/ml aprotinin, 5 μg/ml pepstatin A, 1 mM benzamidine, and 0.1 mM benzethonium chloride. The samples were homogenized on ice for 30 seconds and sonicated for 10 seconds at 10 mV. The tissue samples were centrifuged for 15 minutes at 3,000 rpm and 4°C. The supernatants were assayed for total protein by the bicinchoninic acid method (Micro BCA Protein Assay Kit, Pierce Chemical, Rockford, Ill.). All chemicals were obtained from Sigma (St. Louis).
Semiquantitative Western blots
Adapting previously described methods (8), we resolved the samples on 12% (Bcl-2, procaspase-3), 14% (Bax), and 16% (activated caspase-3) Tris-glycine polyacrylamide gels using a mini-cell electrophoresis unit (XCell II, NOVEX, San Diego). Equal amounts of total protein (10 μg for Bax, 30 μg for Bcl-2, 20 μg for procaspase-3, and 90 μg for activated caspase-3) were boiled for 5 minutes in Tris-glycine sodium dodecyl sulfate sample buffer and applied to triplicate gels. All gels were run with a low-range molecular weight ladder (Rainbow MW Marker, Amersham Pharmacia, Piscataway, N.J.) and controls as follows: HeLa cell lysate (Transduction Laboratories, Lexington, Ky.) for Bax and Jurkat cell lysate (Transduction Laboratories) for Bcl-2 and procaspase-3. The proteins were transferred to polyvinylidene difluoride membranes (Immobilon-P, Millipore, Billerica, Mass.) at 25 V for 90 minutes, and complete transfer was ascertained with Ponceau S stain. Nonspecific protein binding was blocked for 1 hour with 5% blocking reagent in 0.1% Tween in Tris-buffered saline. The membranes were incubated for 90 minutes at 25°C with primary antihuman antibodies as follows: rabbit polyclonal Bax (N-20, 1:2000, Santa Cruz Biotechnology, Santa Cruz, Calif.), mouse monoclonal Bcl-2 (1:500, Transduction Laboratories), mouse monoclonal procaspase-3 (CPP32, 1:1000, Transduction Laboratories), mouse monoclonal activated caspase-3 (IMG 144, 1:200, Imgenex, San Diego, Calif.). The membranes were then incubated for 1 hour with appropriate secondary antibodies labeled with horseradish peroxidase, as follows: antimouse antibodies (1:1000) for monoclonal primary antibodies and antirabbit antibodies (1:1000) for polyclonal primary antibodies. The membranes were developed by using chemiluminescence (ECL, Amersham), and protein bands were detected on radiographic film (Hyperfilm ECL, Amersham). Optical densitometry was performed by using the public domain program NIH Image 1.62 (http://rsb.info.nih.gov/nih-image/), and image analysis was accomplished by means of Adobe Photoshop 5.0 (Adobe Systems, San Jose, Calif.). All raw optical densities were normalized to a pooled control sample applied to all gels to allow for intergel comparisons. In addition, a standard curve of pooled control sample was immunoblotted for each protein to ascertain that all samples fell within the linear portion of the densitometric curve (data not shown). The Bax/Bcl-2 ratio was determined as follows: 1) the optical densities of Bax and Bcl-2 for each sample were standardized to the mean optical densities of the nonpsychiatric comparison group for Bax and Bcl-2, respectively, then 2) Bax/Bcl-2 quotients of the standardized Bax and Bcl-2 values were calculated for each sample. These indicate the difference in the Bax/Bcl-2 ratio for each sample from the mean of the comparison group.
Protein Stability and Drug Effects
Postmortem stability of Bax and caspase-3
Sprague-Dawley rats (150–200 g, Charles River Laboratories, Raleigh, N.C.) were killed and separated into two gender-matched groups (N=4 per group). In one group, the frontal cortex was dissected and immediately frozen at –80°C. To approximate the human postmortem condition, the heads of the other group were kept at 25°C for 6 hours and then at 4°C for 18 hours, after which the frontal cortex was dissected and frozen. All tissues were homogenized and sonicated, and Western blotting of Bax and caspase-3 was performed as described in the preceding by using primary antibodies for Bax and caspase-3. Bcl-2 postmortem stability has previously been determined (27).
Haloperidol effects on Bax, Bcl-2, and caspase-3
Sprague-Dawley rats (150–200 g) received haloperidol, 1 mg/kg per day (N=7), or saline (N=6) intraperitoneally for 7 days. One hour after the final dose, the rats were killed and the frontal cortex was dissected and homogenized. Western blotting was performed by using primary antibodies for Bax, caspase-3, and Bcl-2 as described in the preceding.
Statistical Analysis
Statistical analysis was performed by using GraphPad Prism, version 3.00 (GraphPad Software, San Diego, Calif.). The a priori, primary analysis was limited to the specimens of the nonpsychiatric comparison subjects versus those of the schizophrenia patients, given our hypothesis. The Bax/Bcl-2 ratio was the primary outcome variable, and the two groups were compared by using a Student’s t test with two-tailed p values considered significant at p<0.05. Secondary analyses included individual comparisons of Bax, Bcl-2, and caspase-3 levels (active and zymogen forms) by Student’s t tests in the schizophrenia and comparison groups. Apoptotic protein levels were also compared by means of one-way analysis of variance (ANOVA) across all diagnostic groups. The Bax/Bcl-2 ratio and activated caspase-3 subunit levels were analyzed for age, gender, side of brain, and antipsychotic medication effects. In the rat studies, postmortem stability and haloperidol effects were analyzed by using Student’s t tests.
Results
Bax and Bcl-2 Immunoblots
The mean Bax/Bcl-2 ratio was 50% higher in the schizophrenia group (mean=1.65, SD=0.90) than in the nonpsychiatric comparison group (mean=1.10, SD=0.37) (Figure 1 and Figure 2). Individually, the mean Bax level in the patients with schizophrenia (mean=1.18 normalized optical density, SD=0.59) did not differ from that of the comparison subjects (mean=1.06, SD=0.25) (t=0.72, df=28, p=0.48) (Figure 2), and the mean Bcl-2 level of the schizophrenia group (mean=1.39, SD=0.75) did not differ from that of the comparison group (mean=1.75, SD=0.69) (t=1.35, df=28, p=0.19) (Figure 2). It was the effect of differences in the Bax and Bcl-2 levels and the resulting Bax/Bcl-2 ratio for each individual subject that produced a substantial difference in Bax/Bcl-2 ratio between the two groups.
There was a nearly significant group effect on the mean Bax/Bcl-2 ratio across all psychiatric groups according to ANOVA (F=2.36, df=3, 59, p=0.09). The mean ratios for the patients with bipolar disorder (mean=1.18, SD=0.71) and major depression (mean=1.12, SD=0.52) were close to that of the nonpsychiatric comparison subjects (mean=1.10, SD=0.37). The ratio did not correlate with age across all groups (r2=0.01, N=60, p=0.53) or with lifetime fluphenazine-equivalent dose in the schizophrenia group (r2=0.16, N=15, p=0.14). The Bax/Bcl-2 ratio also did not differ between women (mean=1.11, SD=0.53) and men (mean=1.37, SD=0.75) (t=1.46, df=58, p=0.15) or between the left (mean=1.34, SD=0.57) and right (mean=1.20, SD=0.76) (t=0.80, df=58, p=0.43) sides of the brain across all samples.
Caspase-3 Immunoblots
The immunoblots exposed to activated caspase-3 antibodies revealed two large-fragment bands migrating to 20 and 17 kDa (Figure 1). Three isoforms of the large caspase-3 subunit have previously been described at 20, 19, and 17 kDa (28). Among these bands, the 20-kDa isoform was most readily quantifiable on our gels, and it was 8% lower in the schizophrenia group (mean=1.01 normalized optical density, SD=0.09) than in the nonpsychiatric comparison group (mean=1.10, SD=0.16) (t=2.00, df=27, p=0.06) (Figure 3). The small caspase-3 subunit migrating to 12–14 kDa could not be measured reliably across all samples owing to faint expression and blot artifacts, but no qualitative group differences were evident (Figure 1). Although the difference was not quite significant, the procaspase-3 levels also appeared somewhat lower in the schizophrenia group (mean=0.87 normalized optical density, SD=0.33) than in the nonpsychiatric comparison group (mean=1.08, SD=0.29) (t=1.87, df=28, p=0.08) (Figure 1 and Figure 3).
The mean values for the 20-kDa caspase-3 subunit for the patients with major depression (mean=1.03 normalized optical density, SD=0.09) and bipolar disorder (mean=1.01, SD=0.21) did not produce a group effect by ANOVA (F=1.29, df=3, 58, p=0.29). The 20-kDa caspase-3 values did not correlate with age across all groups (r2=0.00, N=59, p=0.89) or with lifetime fluphenazine-equivalent dose in the schizophrenia group (r2=0.00, N=14, p=0.84). They also did not differ between women (mean=1.00, SD=0.12) and men (mean=1.06, SD=0.16) (t=1.43, df=57, p=0.16) or between the left (mean=1.05, SD=0.17) and right (mean=1.03, SD=0.13) (t=0.57, df=57, p=0.58) sides of the brain across all groups. Finally, the procaspase-3 levels of the patients with major depression (mean=0.90 normalized optical density, SD=0.34) and bipolar disorder (mean=0.94, SD=0.46) did not produce a group effect by ANOVA (F=1.01, df=3, 59, p=0.40).
Postmortem Stability of Bax and Caspase-3
The Western blots of rat cortex revealed high postmortem stability of the Bax and caspase-3 proteins over 24 hours. The mean Bax level in the rat cortex 24 hours postmortem (mean=0.20 optical density, SD=0.02) was not significantly different from that at 0 hours postmortem (mean=0.22, SD=0.02) (t=1.79, df=6, p=0.13). Likewise, the mean value for the caspase-3 20-kDa subunit at 24 hours postmortem (mean=0.22 optical density, SD=0.01) was unchanged from that at 0 hours (mean=0.22, SD=0.00) (t=1.10, df=5, p=0.33).
Haloperidol Effects on Bax, Bcl-2, and Caspase-3
The mean Bax/Bcl-2 ratio in the frontal cortex of the haloperidol-treated rats (mean=0.88, SD=0.12) was not significantly different from that of the rats given the saline control (mean=0.99, SD=0.18) (t=1.47, df=11, p=0.17). There was also no significant difference in the mean Bax level between haloperidol (mean=0.99 normalized optical density, SD=0.10) and saline (mean=1.10, SD=0.23) (t=1.14, df=11, p=0.28) or in the mean Bcl-2 level between haloperidol (mean=0.89, SD=0.06) and saline (mean=0.86, SD=0.06) (t=1.01, df=11, p=0.34). The mean level for the 20-kDa caspase-3 subunit also did not differ between haloperidol (mean=1.01 normalized optical density, SD=0.28) and saline (mean=1.04, SD=0.31) (t=0.21, df=11, p=0.85).
Discussion
In this study, the mean Bax/Bcl-2 ratio in Brodmann’s area 21 was 50% higher in patients with schizophrenia than in nonpsychiatric comparison subjects. This finding provides new evidence that apoptotic proteins contribute to the underlying pathophysiology of schizophrenia. A high Bax/Bcl-2 ratio has been found to increase the vulnerability of neurons to apoptosis (12), and a high ratio occurs in several classic neurodegenerative disorders characterized by progressive neuronal loss (15, 16). In schizophrenia, this finding may be particularly relevant to the evidence for progressive gray matter loss, as seen most consistently in early stages of the disorder (22–24, 29, 30).
The individual mean levels of Bax and Bcl-2 in the schizophrenia patients did not differ from those of the comparison subjects. Using ELISA, we previously detected 25% less Bcl-2 in schizophrenia patients than comparison subjects in Brodmann’s area 21 (8). Numerically, the Bcl-2 level was 21% lower in the schizophrenia patients in the current study, but the lack of significance probably reflects the lower sensitivity of Western blot compared to ELISA. It was necessary to reanalyze Bcl-2 with a different method—Western blot, in triplicate—in the current study in order to derive the Bax/Bcl-2 ratio since a Bax ELISA was unavailable. Furthermore, Western blot has the advantage of quantification and protein identification within one technique, while ELISA does not allow direct visualization of protein bands.
In contrast to the high Bax/Bcl-2 ratio, neither the activated caspase-3 nor procaspase-3 level was statistically different from that of the nonpsychiatric comparison subjects, although they both were modestly lower. This result may indicate that apoptosis is not active in Brodmann’s area 21 in chronic schizophrenia. However, this interpretation is qualified by several considerations. First, apoptosis may be active only during distinct phases of illness. For example, if apoptotic activity is limited to prodromal and new-onset schizophrenia, when progressive gray matter loss has been identified, then the current study using tissue from patients with chronic illness would miss such activity. Second, a high Bax/Bcl-2 ratio may reduce neuronal viability in the absence of caspase-3 activity and/or involve other effector caspases. It is interesting that the somewhat lower caspase-3 levels may have relevance to a recent study demonstrating a subset of neurons with fewer single-stranded DNA breaks in the cingulate cortex of patients with schizophrenia (31). In contrast, consistently higher procaspase-3 and activated caspase-3 levels in Alzheimer’s and Parkinson’s diseases (4, 5) suggest that the pathophysiology of schizophrenia is distinct from classic neurodegeneration.
The current findings raise several other considerations for the pathophysiology of schizophrenia. First, schizophrenia is increasingly conceptualized as a disorder of the synapse, given converging lines of evidence from microarray, neuropathological, and neuroimaging techniques (32, 33). Furthermore, several studies suggest that synaptic remodeling and elimination may be influenced by apoptotic protein activity in a process termed “synaptic apoptosis” (34, 35). Although this process is speculative, if synaptic loss in schizophrenia is mediated by apoptotic protein activity and is episodic rather than continuous (e.g., during adolescent synaptic pruning or in response to discrete episodes of excitotoxic stress), then the caspase-3 level may be increased only transiently and then return to baseline. Second, the data do not rule out an early neurodevelopmental role for apoptosis in schizophrenia. A fetal or perinatal insult could transiently alter normal developmental apoptosis to yield enduring cytoarchitectural deficits. It is interesting that multiple proapoptotic stimuli, such as ischemia, hypoxia, and pro-inflammatory cytokines (36, 37), have also been implicated as insults during early life that increase the risk of developing schizophrenia (38–40). Studies of the normal developmental pattern of apoptotic protein expression in the human frontal cortex have revealed that infancy is the period of highest Bax levels and lowest Bcl-2 levels across the lifespan (27, 41). This suggests that neuronal apoptosis during the perinatal period may be especially vulnerable to dysregulation from neurodevelopmental insults.
All of the subjects in this study were group matched for age, gender, postmortem interval, side of brain, and brain pH, limiting the potential confounding effects of these variables. Although chronic antipsychotic treatment may alter the expression of apoptotic proteins, one week of haloperidol treatment in animals did not produce changes. Furthermore, cumulative antipsychotic medication exposure did not correlate with the Bax/Bcl-2 ratio or caspase-3 level. Postmortem interval could affect results if apoptotic proteins demonstrated poor postmortem stability. However, the study of postmortem stability showed no change in Bax, procaspase-3, or activated caspase-3 (20-kDa subunit) over 24 hours. Similarly, high postmortem stability for Bcl-2 was previously determined (27). Finally, patient-related factors, including diagnostic heterogeneity, substance abuse, and medical illness, could introduce unintended variability, but efforts were made to limit these factors during subject selection (26).
This study revealed limited associations with other psychiatric diagnoses. The Bax/Bcl-2 ratio and caspase-3 level were essentially no different in the patients with bipolar disorder or major depression from those in the nonpsychiatric comparison subjects. Since substantially lower cortical volume and neuronal and glial density have been documented in affective disorders (42, 43), this finding suggests that divergent pathophysiological mechanisms may underlie the neuropathology of schizophrenia and primary affective disorders.
In conclusion, this study indicates that apoptotic proteins may reduce neuronal viability in adult schizophrenia without inducing large-scale apoptosis. An intriguing possibility that merits further inquiry is the extent to which apoptotic proteins influence synaptic elimination and thereby contribute to neuropil loss in schizophrenia. The differential regulation of the expression of Bax, Bcl-2, and caspase-3 proteins demonstrates that the pathophysiology of schizophrenia is distinct from that of classic neurodegenerative disorders. Ultimately, if clinically relevant roles for apoptotic proteins can be demonstrated, their pharmacological manipulation may permit novel approaches to schizophrenia treatment.
![]() |
Presented at the 8th International Congress of Schizophrenia Research, Whistler, B.C., Canada, April 28 to May 2, 2001. Received July 3, 2002; revision received April 10, 2003; accepted May 14, 2003. From the Schizophrenia Research Center, Department of Psychiatry, University of North Carolina School of Medicine. Address reprint requests to Dr. Jarskog, Department of Psychiatry, University of North Carolina School of Medicine, C.B. 7160, Chapel Hill, NC 27599-7160; [email protected] (e-mail). Supported by NIMH grant MH-01752 and National Alliance for Research on Schizophrenia and Depression Young Investigator Award to Dr. Jarskog, NIMH grant MH-33127 to Dr. Lieberman, and NIMH grant MH-60352 to Dr. Gilmore. The authors thank the Stanley Medical Research Institute and Drs. Michael Knable, Serge Weis, Fuller Torrey, Maree Webster, and Robert Yolken for the postmortem brains.
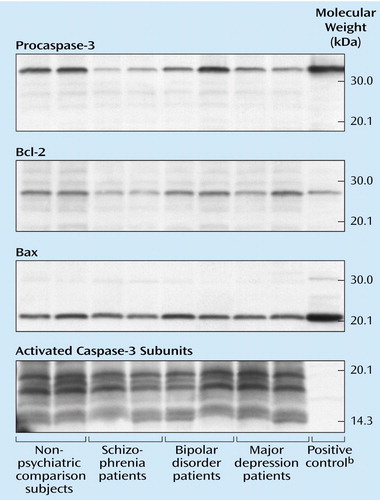
Figure 1. Representative Western Blots of Procaspase-3, Bcl-2, Bax, and Activated Caspase-3 Subunit Proteins in Postmortem Brain Tissue From Brodmann’s Area 21 in Two Nonpsychiatric Comparison Subjects and Two Patients Each With Schizophrenia, Bipolar Disorder, and Major Depressiona
aEqual amounts of total protein (20 μg for procaspase-3, 10 μg for Bax, 30 μg for Bcl-2, 90 μg for activated caspase-3) were resolved on 12% (Bcl-2, procaspase-3), 14% (Bax), and 16% (activated caspase-3) Tris-glycine polyacrylamide gels and transferred to polyvinylidene difluoride membranes, which were incubated with the appropriate primary antihuman antibodies and then developed by using chemiluminescence on radiographic film. The bands migrated to 32 kDa (procaspase-3), 26 kDa (Bcl-2), 21 kDa (Bax), and 20, 17, and 12–14 kDa (activated caspase-3 isoforms) according to molecular weight markers applied to all gels.
bPositive controls were applied to the gels to further ascertain correct band identification, as follows: Jurkat cell lysate for Bcl-2 (26 kDa) and procaspase-3 (32 kDa), HeLa cell lysate for Bax (21 kDa).
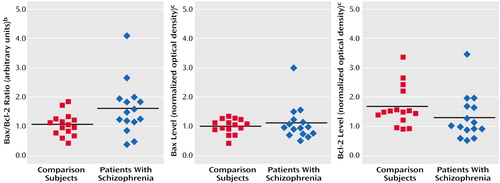
Figure 2. Bax/Bcl-2 Ratio and Bax and Bcl-2 Levels in Postmortem Brain Tissue From Brodmann’s Area 21 in Patients With Schizophrenia (N=15) and Nonpsychiatric Comparison Subjects (N=15)a
aLevels were measured by semiquantitative Western blot. Black horizontal lines are mean values.
bDerived from normalizing individual Bax and Bcl-2 optical densities to the mean optical densities of the comparison group for Bax and Bcl-2, respectively, then calculating a Bax/Bcl-2 ratio for each individual. The mean ratio was significantly higher in the schizophrenia group than in the comparison group (t=2.19, df=28, p=0.04, two-tailed test).
cDetermined by optical densitometry of triplicate protein bands and normalization of each band to a pooled control sample applied to each gel.
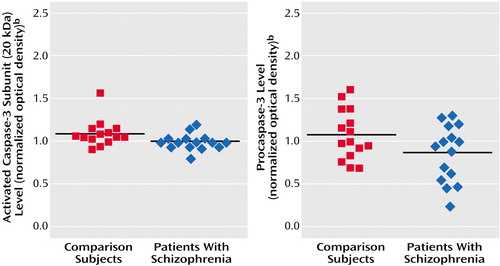
Figure 3. Levels of the 20-kDa Activated Caspase-3 Subunit and Procaspase-3 in Postmortem Brain Tissue From Brodmann’s Area 21 in Patients With Schizophrenia (N=15) and Nonpsychiatric Comparison Subjects (N=15)a
aLevels were measured by semiquantitative Western blot. The level of the activated caspase-3 subunit was missing for one comparison subject. The black horizontal lines are mean values.
bDetermined by optical densitometry of triplicate protein bands and normalization of each band to a pooled control sample applied to each gel.
1. Oppenheim RW: Cell death during development of the nervous system. Annu Rev Neurosci 1991; 14:453–501Crossref, Medline, Google Scholar
2. Bredesen DE: Neural apoptosis. Ann Neurol 1995; 38:839–851Crossref, Medline, Google Scholar
3. Su JH, Deng G, Cotman CW: Bax protein expression is increased in Alzheimer’s brain: correlations with DNA damage, Bcl-2 expression and brain pathology. J Neuropathol Exp Neurol 1997; 56:86–93Crossref, Medline, Google Scholar
4. Masliah E, Mallory M, Alford M, Tanaka S, Hansen L: Caspase dependent DNA fragmentation might be associated with excitotoxicity in Alzheimer’s disease. J Neuropathol Exp Neurol 1998; 57:1041–1052Crossref, Medline, Google Scholar
5. Hartmann A, Hunot S, Michel PP, Muriel MP, Vyas S, Faucheux BA, Mouatt-Prigent A, Turmel H, Srinivasan A, Ruberg M, Evan GI, Agid Y: Caspase-3: a vulnerability factor and final effector in apoptotic death and dopaminergic neurons in Parkinson’s disease. Proc Natl Acad Sci USA 2000; 97:2875–2880Crossref, Medline, Google Scholar
6. Margolis RL, Chuang DM, Post RM: Programmed cell death: implications for neuropsychiatric disorders. Biol Psychiatry 1994; 35:946–956Crossref, Medline, Google Scholar
7. Lieberman JA: Is schizophrenia a neurodegenerative disorder? a clinical and neurobiological perspective. Biol Psychiatry 1999; 46:729–739Crossref, Medline, Google Scholar
8. Jarskog LF, Gilmore JH, Selinger ES, Lieberman JA: Cortical Bcl-2 protein expression and apoptotic regulation in schizophrenia. Biol Psychiatry 2000; 48:641–650Crossref, Medline, Google Scholar
9. Job DE, Whalley HC, McConnell S, Glabus M, Johnstone EC, Lawrie SM: Structural gray matter differences between first-episode schizophrenics and normal control subjects using voxel-based morphometry. Neuroimage 2002; 17:880–889Crossref, Medline, Google Scholar
10. Shenton ME, Dickey CC, Frumin M, McCarley RW: A review of MRI findings in schizophrenia. Schizophr Res 2001; 49:1–52Crossref, Medline, Google Scholar
11. Hulshoff Pol HE, Schnack HG, Bertens MGBC, van Haren NEM, van der Tweel I, Staal WG, Baaré WFC, Kahn RS: Volume changes in gray matter in patients with schizophrenia. Am J Psychiatry 2002; 159:244–250Link, Google Scholar
12. Oltvai ZN, Milliman CL, Korsmeyer SJ: Bcl-2 heterodimerizes in vivo with a conserved homolog, Bax, that accelerates programmed cell death. Cell 1993; 74:609–619Crossref, Medline, Google Scholar
13. Vekrellis K, McCarthy MJ, Watson A, Whitfield J, Rubin LL, Ham J: Bax promotes neuronal cell death and is downregulated during the development of the nervous system. Development 1997; 124:1239–1249Medline, Google Scholar
14. Pollack M, Phaneuf S, Dirks A, Leeuwenburgh C: The role of apoptosis in the normal aging brain, skeletal muscle, and heart. Ann NY Acad Sci 2002; 959:93–107Crossref, Medline, Google Scholar
15. Sawa A, Oyama F, Cairns NJ, Amano N, Matsushita M: Aberrant expression of bcl-2 gene family in Down’s syndrome brains. Mol Brain Res 1997; 48:53–59Crossref, Medline, Google Scholar
16. Paradis E, Douillard H, Koutroumanis M, Goodyer C, LeBlanc A: Amyloid β peptide of Alzheimer’s disease downregulates Bcl-2 and upregulates Bax expression in human neurons. J Neurosci 1996; 16:7533–7539Crossref, Medline, Google Scholar
17. Thornberry NA, Lazebnik Y: Caspases: enemies within. Science 1998; 281:1312–1316Crossref, Medline, Google Scholar
18. Yuan J, Yanker BA: Apoptosis in the nervous system. Nature 2000; 407:802–809Crossref, Medline, Google Scholar
19. Krajewska M, Wang HG, Krajewski S, Zapata JM, Shabaik A, Gascoyne R, Reed JC: Immunohistochemical analysis of in vivo patterns of expression of CPP32 (Caspase-3), a cell death protease. Cancer Res 1997; 57:1605–1613Medline, Google Scholar
20. Shenton ME, Kikinis R, Jolesz FA, Pollak SD, LeMay M, Wible CG, Hokama H, Martin J, Metcalf D, Coleman M, McCarley RW: Abnormalities of the left temporal lobe and thought disorder in schizophrenia: a quantitative MRI study. N Engl J Med 1992; 327:604–612Crossref, Medline, Google Scholar
21. Suddath RL, Casanova MF, Goldberg TE, Daniel DG, Kelsoe JR Jr, Weinberger DR: Temporal lobe pathology in schizophrenia: a quantitative magnetic resonance imaging study. Am J Psychiatry 1989; 146:464–472Link, Google Scholar
22. Gur RE, Cowell P, Turetsky BI, Gallacher F, Cannon T, Bilker W, Gur RC: A follow-up magnetic resonance imaging study of schizophrenia: relationship of neuroanatomical changes to clinical and neurobehavioral measures. Arch Gen Psychiatry 1998; 55:145–152Crossref, Medline, Google Scholar
23. Rapoport JL, Giedd JN, Blumental J, Hamburger S, Jeffries N, Fernandez T, Nicolson R, Bedwell J, Lenane M, Zijdenbos A, Paus T, Evans A: Progressive cortical change during adolescence in childhood onset schizophrenia: a longitudinal MRI study. Arch Gen Psychiatry 1999; 56:649–654Crossref, Medline, Google Scholar
24. Kasai K, Shenton ME, Salisbury DF, Hirayasu Y, Lee C-U, Ciszewski AA, Yurgelun-Todd D, Kikinis R, Jolesz FA, McCarley RW: Progressive decrease of left superior temporal gyrus gray matter volume in patients with first-episode schizophrenia. Am J Psychiatry 2003; 160:156–164Link, Google Scholar
25. Woodruff PWR, Wright IC, Bullmore ET, Brammer M, Howard RJ, Williams SCR, Shapleske J, Rossell S, David AS, McGuire PK, Murray RM: Auditory hallucinations and the temporal cortical response to speech in schizophrenia: a functional magnetic resonance imaging study. Am J Psychiatry 1997; 154:1676–1682Link, Google Scholar
26. Torrey EF, Webster M, Knable M, Johnston N, Yolken RH: The Stanley Foundation brain collection and neuropathology consortium. Schizophr Res 2000; 44:151–155Crossref, Medline, Google Scholar
27. Jarskog LF, Gilmore JH: Developmental expression of Bcl-2 protein in human cortex. Dev Brain Res 2000; 119:225–230Crossref, Medline, Google Scholar
28. Fernandes-Alnemri T, Armstrong RC, Krebs J, Srinivasula SM, Wang L, Bullrich F, Fritz LC, Trapani JA, Tomaselli KJ, Litwack G, Alnemri ES: In vitro activation of CPP32 and Mch3 by Mch4, a novel human apoptotic cysteine protease containing two FADD-like domains. Proc Natl Acad Sci USA 1996; 93:7464–7469Crossref, Medline, Google Scholar
29. DeLisi LE, Sakuma M, Tew W, Kushner M, Hoff AL, Grimson R: Schizophrenia as a chronic active brain process: a study of progressive brain structural change subsequent to the onset of schizophrenia. Psychiatry Res Neuroimaging 1997; 74:129–140Crossref, Medline, Google Scholar
30. Cahn W, Hulshoff Pol HE, Lems EBTE, van Haren NEM, Schnack HG, van der Linden JA, Schothorst PF, van Engeland H, Kahn RS: Brain volume changes in first-episode schizophrenia. Arch Gen Psychiatry 2002; 59:1002–1010Crossref, Medline, Google Scholar
31. Benes FM, Walsh J, Bhattacharyya S, Sheth A, Berretta S: DNA fragmentation is decreased in schizophrenia but not bipolar disorder. Arch Gen Psychiatry 2003; 60:359–364Crossref, Medline, Google Scholar
32. Lewis DA: Development of the prefrontal cortex during adolescence: insights into vulnerable neural circuits in schizophrenia. Neuropsychopharmacology 1997; 16:385–398Crossref, Medline, Google Scholar
33. Mirnics K, Middleton FA, Lewis DA, Levitt P: Analysis of complex brain disorders with gene expression microarrays: schizophrenia as a disease of the synapse. Trends Neurosci 2001; 24:479–486Crossref, Medline, Google Scholar
34. Mattson MP, Keller JN, Begley JG: Evidence for synaptic apoptosis. Exp Neurol 1998; 153:35–48Crossref, Medline, Google Scholar
35. Festoff BW, Suo Z, Citron BA: Plasticity and stabilization of neuromuscular and CNS synapses: interactions between thrombin protease signaling pathways and tissue transglutaminase. Int Rev Cytol 2001; 211:153–177Crossref, Medline, Google Scholar
36. Thompson CB: Apoptosis in the pathogenesis and treatment of disease. Science 1995; 267:1456–1462Crossref, Medline, Google Scholar
37. Charriaut-Marlangue C, Aggoun-Zouaoui D, Represa A, Ben-Ari Y: Apoptotic features of selective neuronal death in ischemia, epilepsy and gp120 toxicity. Trends Neurosci 1996; 19:109–114Crossref, Medline, Google Scholar
38. Gilmore JH, Jarskog LF: Exposure to infection and brain development: cytokines in the pathogenesis of schizophrenia (letter). Schizophr Res 1997; 24:365–367Crossref, Medline, Google Scholar
39. Geddes JR, Lawrie SM: Obstetric complications and schizophrenia: a meta-analysis. Br J Psychiatry 1995; 167:786–793Crossref, Medline, Google Scholar
40. Urakubo A, Jarskog LF, Lieberman JA, Gilmore JH: Prenatal exposure to maternal infection alters cytokine expression in the placenta, amniotic fluid, and fetal brain. Schizophr Res 2001; 47:27–36Crossref, Medline, Google Scholar
41. Jarskog LF, Selinger ES, Lieberman JA, Gilmore JH: Developmental downregulation of Bax protein in human frontal cortex. Abstracts of the Society for Neuroscience 2001, number 28.5Google Scholar
42. Drevets WC, Price JL, Simpson JR, Todd RD, Reich T, Vannier M, Raichle ME: Subgenual prefrontal cortex abnormalities in mood disorders. Nature 1997; 386:824–827Crossref, Medline, Google Scholar
43. Rajkowska G, Miguel-Hidalgo JJ, Wei J, Dilley G, Pittman SD, Meltzer HY, Overholser JC, Roth BL, Stockmeier CA: Morphometric evidence for neuronal and glial prefrontal cell pathology in major depression. Biol Psychiatry 1999; 45:1085–1098Crossref, Medline, Google Scholar