Effect of Schizophrenia on Frontotemporal Activity During Word Encoding and Recognition: A PET Cerebral Blood Flow Study
Abstract
OBJECTIVE: Neuropsychological studies have shown that deficits in verbal episodic memory in schizophrenia occur primarily during encoding and retrieval stages of information processing. The current study used positron emission tomography to examine the effect of schizophrenia on change in cerebral blood flow (CBF) during these memory stages. METHOD: CBF was measured in 23 healthy comparison subjects and 23 patients with schizophrenia during four conditions: resting baseline, motor baseline, word encoding, and word recognition. The motor baseline was used as a reference that was subtracted from encoding and recognition conditions by using statistical parametric mapping. RESULTS: Patients’ performance was similar to that of healthy comparison subjects. During word encoding, patients showed reduced activation of left prefrontal and superior temporal regions. Reduced left prefrontal activation in patients was also seen during word recognition, and additional differences were found in the left anterior cingulate, left mesial temporal lobe, and right thalamus. Although patients’ performance was similar to that of healthy comparison subjects, left inferior prefrontal activation was associated with better performance only in the comparison subjects. CONCLUSIONS: Left frontotemporal activation during episodic encoding and retrieval, which is associated with better recognition in healthy people, is disrupted in schizophrenia despite relatively intact recognition performance and right prefrontal function. This may reflect impaired strategic use of semantic information to organize encoding and facilitate retrieval.
Neuropsychological studies show that deficits in verbal episodic memory (1) in schizophrenia occur primarily during encoding and retrieval, with relative sparing of long-term storage (2, 3). Functional imaging of healthy subjects documents regional differences in activity during encoding and retrieval. A general finding, summarized in the hemispheric encoding/retrieval asymmetry model (4), is that episodic encoding and semantic retrieval activate the left prefrontal cortex, whereas episodic retrieval activates right prefrontal regions. Subsequent studies supported a distinct role for the right prefrontal cortex during episodic retrieval but found less evidence of hemispheric and task specificity for left prefrontal regions (5–8). Mesial temporal lobe distinctions have also been made on the basis of positron emission tomography (PET) (9–11): the rostral portion has been implicated in encoding, and the caudal portion has been implicated in retrieval. Functional magnetic resonance imaging has given different results (12), and some have argued that the mesial temporal lobe is continuously engaged (13) and that location and degree of mesial temporal lobe activity is related more to success (14, 15) or stimulus novelty (16–19) than to stage of information processing. The purpose of the current study is to examine changes in cerebral blood flow (CBF) during word encoding and retrieval to assess the impact of schizophrenia on frontotemporal function.
Most imaging studies of episodic memory in schizophrenia have examined episodic retrieval and found frontotemporal dysfunction (20–22). Disrupted left medial and superior temporal lobe function and atypical middle temporal lobe asymmetries were documented in xenon-133 studies of word and face recognition (23, 24). A series of PET studies of prose recall demonstrated prefrontal, thalamic, and cerebellar dysfunction (25–27). Frontotemporal abnormalities were found in free recall studies of supraspan word lists (28) and recognition studies of visual paired-associate learning (29). The mesial temporal lobe was implicated in studies of word-stem completion following shallow and deep encoding (30, 31). These studies found hippocampal dysfunction across patients and left prefrontal dysfunction primarily in patients with deficit symptoms.
Studies of episodic encoding are more recent. A single photon emission computed tomography study (32) measured CBF while patients and comparison subjects learned word lists of increasing semantic relatedness. The patients did not use semantic information to organize encoding, and their frontal and anterior cingulate regions were not activated. Degree of left inferior prefrontal activation was associated with patients’ use of semantic information to cluster words. Use of a semantic clustering strategy was also examined in a PET study of glucose metabolism that used a modification of the California Verbal Learning Test (33). Patients used a serial order rather than semantic clustering strategy and had lower metabolism in frontal and temporal regions. Use of the serial order strategy correlated with reduced frontal and increased temporal metabolism. Finally, CBF was measured while patients learned and recalled lists ranging from one to 12 items in a word-stem completion task (34). Increased memory demands led to prefrontal increases only in healthy comparison subjects, leading to the conclusion that hypofrontality is found only under conditions of cognitive stress.
To our knowledge, functional imaging studies of episodic memory in schizophrenia have not yet examined encoding and retrieval in the same experiment. This would be particularly informative given evidence that, although encoding deficits are prominent (35), recognition may be relatively well preserved (36), particularly for nonrelational recognition tasks requiring simple discrimination between new and old items (37). In our previous study of word encoding and recognition in healthy subjects using the current paradigm, we found bilateral prefrontal effects, with greater right prefrontal and anterior cingulate activation during recognition (38). Given these previous findings, we hypothesized that the recognition performance of patients with schizophrenia is relatively intact and that frontotemporal abnormalities are more prominent during word encoding.
Method
Participants
Participants were 23 patients (18 men and five women) and 23 healthy comparison subjects (12 men and 11 women) from the Regional Brain Function in Schizophrenia Center at the University of Pennsylvania. Comparison subjects were selected from a previous study group (38) and were chosen to match patients in age (comparison subjects’ mean age=30.7 years, SD=10.0; patients’ mean age=35.2, SD=9.9), parental education (comparison subjects’ mean level=14.1 years, SD=3.0; patients’ mean level=12.9 years, SD=2.8), and handedness (there were three left-handers in each group). As expected, the patients had fewer years of education (mean=13.8, SD=2.8, versus mean=15.9, SD=2.2) (t=2.8, df=42, p<0.01). Because illness disrupts educational attainment, participants were not matched in education. To do so would have created a matching fallacy in which patients were compared with undereducated healthy subjects (39).
Patients had a DSM-IV diagnosis of schizophrenia established by medical, neurological, and psychiatric evaluations (40, 41). Comparison subjects also underwent standard medical, neurological, and psychiatric evaluations (42, 43). Participants had no history of substance abuse or other medical, psychiatric, or neurological disorder that might affect brain function.
All patients were outpatients and ranged between mildly and moderately ill according to their scores on the Scale for the Assessment of Negative Symptoms (SANS) (44) (mean score=26.4, SD=19.6, range=0–69), Scale for the Assessment of Positive Symptoms (SAPS) (45) (mean score=16.5, SD=13.0, range=0–39) and Brief Psychiatric Rating Scale (BPRS) (46) (mean score=31.8, SD=10.1, range=21–63). Clinical scales and PET scans were administered within the same month (median=21 days). Patients were reassessed on the day of the study to determine that no change in clinical status had occurred. One patient had been rated clinically a year before the study, but this patient had stable symptoms.
Patients had received a mean dose of 204.9 mg/day in chlorpromazine equivalents (SD=391.8, range=0–1479.5) of typical neuroleptics and 119.0 mg in chlorpromazine equivalents (SD=267.6, range=0–739.8) of atypical neuroleptics over their lifetime. Their mean age at onset of schizophrenia was 23.5 (SD=7.4), and the mean duration of illness was 12.2 years (SD=9.2). Ten patients were neuroleptic naive, and 13 were receiving medication; six were receiving typical neuroleptics, four atypical, and three both typical and atypical.
There were no differences in education, parental education, age at onset, duration of illness, or BPRS symptoms between medication-naive and medicated patients (all t values <1). However, medication-naive patients had more severe symptoms on the SANS (mean score=40.1, SD=18.5, versus mean score=18.3, SD=15.2) (t=2.9, df=18, p<0.05). Medication-naive patients had nonsignificantly more severe SAPS ratings than medicated patients (mean=22.9, SD=13.9, versus mean=12.4, SD=11.4) (t=1.9, df=19, p=0.07) and were slightly younger (mean=30.6, SD=5.4, versus mean=38.0, SD=11.1) (t=–1.7, df=19, p=0.09). After complete description of the study, written informed consent was obtained from all participants.
Tasks
Images were acquired during four conditions: resting baseline, sequential finger movement, word encoding, and word recognition. Stimuli were visually presented by means of a computer and Power Laboratory software (47) on a monitor mounted from the ceiling at an angle perpendicular to the subjects’ line of sight. Words were centered on the screen as white block letters against a blue background. Words were presented for 5 seconds with a 2-second interstimulus interval during which a blank blue screen appeared. Word encoding preceded word recognition. The order of resting and motor baselines was variable.
Participants held a computer game pad in their right hand and made a button press every time a word was presented during the encoding condition and every time a word was recognized during the recognition condition. Button presses were visually monitored during encoding to ensure that participants were engaged and responding. Button presses and reaction times were automatically recorded during recognition to permit calculation of performance indexes. Lights were dimmed, and background noise was kept to a minimum.
Each condition was started 30 seconds before injection of 15O-labeled water to assure that participants were fully engaged. Conditions were separated by approximately 15 minutes to permit infused radioactivity to decay before the next infusion, resulting in a total study time of approximately 1 hour and 20 minutes.
The resting baseline was a standard condition (48) (eyes open, ears unoccluded) during which participants were instructed to “relax with your eyes open, and refrain from talking or movement.” The motor baseline was developed as a reference task to control for motor activation during the acquisition and recognition conditions (38). During the motor condition, participants sequentially touched their right thumb to the fingers on their right hand using large-amplitude finger movements at a rate of about 60 finger/thumb oppositions per minute.
Encoding and recognition conditions used the Penn Word Recognition Test (24, 38, 49, 50). Briefly, the imaging version of the Penn Word Recognition Test consists of real words, 20 targets and 20 foils, presented at a rate of 5 seconds each. Targets and foils were selected from a standard word list (51) and were balanced in frequency, concreteness, and imageability. During the encoding condition participants were instructed, “You will see 20 words, one at a time. When you see a word press the button. Look at the word the whole time it is on the screen and try to remember it. After you see the words once, there will be a blank screen, and then you will see the same words a second time. Continue to look at the words the second time and try to remember them.” Participants were presented with two repetitions of the 20 target items in a fixed order. During recognition, participants were instructed, “Now we are going to show you more words. This time, some of the words will be exactly the same as the words that you just saw, and some of the words will be different. If the word is the same as one you were previously shown push the button as quickly as you can. If the word is not one that you previously saw, do not press the button. If you are unsure, please guess. You will have 5 seconds to respond to each item.”
Forty trials were presented; half of the trials contained targets and half contained foils. Targets and foils were randomly assigned to each trial. Participants had no difficulty understanding task instructions. Practice was given on the computer game pad to ensure that participants were pressing the correct buttons before scanning.
Participants’ responses during recognition were recorded as true positive, false positive, true negative, and false negative. Two primary measures were examined. Discriminability was calculated as a measure of performance success, and true positive reaction time was calculated as a measure of performance effort. A response bias index was also calculated to assess response strategy. Discriminability and response bias index were calculated following the two-high threshold theory (52), which generates statistically independent signal detection indexes.
Discriminability was calculated by subtracting the false alarm rate ([false positives+0.5]/[number of foils+1.0]) from the hit rate ([true positives+0.5]/[number of targets+1.0]). Values of 0.5 and 1.0 were added to the numerators and denominators to avoid division by zero. Discriminability reflects the proportion of time that an individual is either certain that an item is a target or certain that it is a foil and is an index of memory accuracy.
Response bias was calculated as follows: response bias index=false alarm rate/(1–discriminability). This represents the proportion of items on which individuals say yes when they are uncertain. A value of 0.5 reflects a neutral bias in which there is a 50/50 chance of saying yes or no when uncertain. Response bias index values greater than 0.5 indicate a liberal response bias, and values below 0.5 reveal a conservative response bias.
Reaction time was measured by calculating the median reaction time (in milliseconds) for true positive responses.
PET Data Acquisition
Images were acquired by using the UGM 240H volume imaging scanner (53) composed of 6 NaI(Tl) crystals in a hexagonal arrangement. The camera has a 12.8-cm axial field of view and produces 64 image slices with an interslice distance of 2 mm. Spatial resolution was 5.5 mm in all directions (UGM Medical Systems [54]). Participants’ heads were aligned along the orbitomeatal line, and manual head holder restraints reduced movement.
Participants received four intravenous injections of 15O-labeled water according to a modified bolus ramped-infusion technique (55, 56). Intravenous lines were started approximately 10–15 minutes before scanning. This allowed time for activity to arrive from the cyclotron and time for participants to get used to the intravenous lines. A programmed infusion of 45 mCi of 15O-labeled water was delivered over 3.3 minutes. This results in rapid elevation of tissue activity to the peak of the count rate capability of the camera. The measuring period continues over 4.21 minutes, and dynamic scans were obtained continuously. Tissue activity concentrations peak over the first 1.5 minutes of the measurement period and then leveled because of increased washout (56). Resulting images were corrected for attenuation, scatter, and dead time and used to obtain regional activity concentration of 15O-labeled water.
Image Analysis
Analysis of PET images was accomplished with SPM 96 statistical parametric mapping software (57, 58). Images were realigned to the first image to help correct for head movement. Realigned images were normalized into a common space (59) by using the standard PET template. A customized bounding box (–78:78, –112:76, –40:64) was used to account for a restricted field of view in the z direction. Images were smoothed by using a 15-mm full width at half maximum Gaussian kernel. Proportional scaling was used to adjust values in each voxel by the global mean for each scan. Scaled images were set to a common grand mean of 50 ml/100 g per minute.
Separate SPM 96 paired t test analyses of patient and comparison subject data were used to detect task-related differences within groups. Unpaired SPM 96 t test analyses examined between-group differences These analyses provided an index of task-related activation and yielded a t statistic for the difference at each voxel. Only voxels with a positive signal change were analyzed. These within-group t statistics were expressed as standardized z scores in final projection maps and thresholded at p<0.005, uncorrected, requiring 50 contiguous voxels, corresponding to a minimum t value of 2.8. As noted in previous imaging studies of memory in schizophrenia (34), a lowered threshold helps avoid type II error and is justified given the large body of research documenting prefrontal dysfunction in schizophrenia. However, results for nonprefrontal regions should be interpreted with caution.
Output coordinates of activated voxels were expressed in Talairach space (x, y, z coordinates) (59) for identifying functional Brodmann’s areas. Pearson product moment correlations were used to examine the relationship between task performance (discriminability) and differences between task performance and the motor baseline in scaled pixel intensity. Correlation analyses were performed by using MEDx software (60).
Results
Performance
Recognition levels were high for both groups (comparison subjects’ mean=85.6% correct, SD=9.7%; patients’ mean=79.3% correct, SD=10.9%). Because of software problems, responses were not recorded for three comparison subjects and four patients. Reaction time data were also missing for four comparison subjects and four patients. Given evidence of gender differences (61), gender and diagnosis were examined by entering the three performance indexes in a repeated measures multivariate analysis of variance (MANOVA) with two grouping factors (gender and diagnosis) and one repeated factor (performance index). Alpha level was set at 0.05, two-tailed, for all analyses. There was no main effect of gender or any gender interactions. However, there was an effect of index (F=47.0, df=2, 26, p<0.0001) and a significant diagnosis-by-index interaction (F=4.0, df=2, 26, p<0.05). Therefore, data were collapsed across gender.
T tests revealed no difference in reaction time for correct responses between patients (reaction time mean=1.3 seconds, SD=0.5) and comparison subjects (reaction time mean=1.1 seconds, SD=0.3) (t=–1.5, df=29, p>0.10), suggesting that groups were exerting similar levels of mental effort. However, patients had nonsignificantly less accurate recognition performance (discriminability mean=0.56, SD=0.20) than comparison subjects (discriminability mean=0.68, SD=0.18) (t=1.9, df=37, p=0.06). Patients also identified items as targets when unsure nonsignificantly more frequently—a “liberal response bias” (patients’ response bias index mean=0.35, SD=0.20; comparison subjects’ response bias index mean=0.24, SD=0.17) (t=1.8, df=37, p=0.07).
Medication effects were examined by performing the MANOVA with medication status (naive versus previously treated) as a group factor. This analysis revealed a main effect of index (F=54.3, df=2, 14, p<0.0001) but did not reveal any main effects of group or any group-by-index interactions. These medication results remained unchanged when the SANS was added as a covariate to control for clinical differences.
Regional Cerebral Blood Flow Change
Motor activation
Before we examined results on the Penn Word Recognition Test, we inspected differences between motor and resting baselines. Analysis of motor effects, which should be intact in psychiatric disorders (62–64), provides an “internal standard” to ensure that group differences in cognitive effects are not artifactual (65). When the resting baseline was subtracted from the motor condition, both groups produced maximal activation in the left sensorimotor cortex (patients: z score=5.8, N=23, p<0.0001; x, y, z=–33, –25, 44; comparison subjects: z score=5.4, N=23, p<0.0001; x, y, z=–33, –27, 44; Brodmann’s areas 1, 2, 3, and 4). A between-group comparison (healthy subjects and patients with schizophrenia) failed to reveal any above-threshold differences in this sensorimotor activation. Likewise, there were no between-group differences in sensorimotor effects between medicated and unmedicated patients. Thus, there did not appear to be any systematic artifacts affecting our ability to detect robust sensorimotor activation.
Activation during word encoding
Regional activation during word encoding in healthy volunteers has been described elsewhere (38) for 25 healthy subjects; 23 of these subjects participated in the current study. The comparison subjects in the current study produced the same maximal activation in the left inferior prefrontal cortex (Brodmann’s areas 45 and 46) (Table 1) as those in the earlier study. Effects were restricted to these areas in the previous study. However, the lower threshold in the current study revealed additional bilateral effects in the inferior temporal gyrus (Brodmann’s areas 20 and 37) and left hemisphere effects in the supplementary motor area (Brodmann’s area 6). Additional right hemisphere effects were found in the insula (Brodmann’s area 47), the occipital cortex (Brodmann’s area 18), and the cerebellum. When patients’ CBF values during the baseline motor task were subtracted from those during word encoding, activation was found in bilateral superior frontal regions (Brodmann’s area 10), the left superior temporal gyrus (Brodmann’s area 38), left cerebellum, and right thalamus.
Differences in task-related activation were examined by subtracting the CBF values of patients from those of healthy subjects (Figure 1, Table 1). This comparison revealed that healthy subjects had relatively greater left inferior prefrontal activation (Brodmann’s area 45). They also showed greater left hemisphere activation in the middle and superior frontal gyrus (Brodmann’s areas 8 and 9) and in the superior temporal gyrus (Brodmann’s area 38).
To assess the impact of medication, the CBF values of medicated patients were subtracted from those of the neuroleptic-naive patients (Table 1). This analysis showed that unmedicated patients had greater left hemisphere activation in the cerebellum and primary motor cortex (Brodmann’s area 4).
Activation during word recognition
Word recognition results for healthy volunteers were also described in our earlier study (38). Like the subjects in the earlier study, the comparison subjects in the current study showed bilateral prefrontal activation (Brodmann’s areas 45 and 46) (Table 1). Effects were also seen in the left anterior cingulate gyrus (Brodmann’s area 32), left middle frontal gyrus (Brodmann’s area 6), left superior frontal gyrus (Brodmann’s area 8), left inferior temporal gyrus (Brodmann’s area 20), and bilateral fusiform gyrus (Brodmann’s areas 18 and 20). In patients, activation was detected in the left middle frontal gyrus (Brodmann’s area 9), right superior frontal gyrus (Brodmann’s area 6), left middle temporal gyrus (Brodmann’s area 20), bilateral inferior temporal gyrus (Brodmann’s area 37), left cingulate gyrus (Brodmann’s area 24), left middle occipital gyrus (Brodmann’s area 19), and bilateral inferior occipital regions (Brodmann’s areas 18 and 19).
Results of the comparison of healthy subjects and patients with schizophrenia are presented in Figure 2 and Table 1. Comparison subjects had greater prefrontal activation in the left hemisphere (Brodmann’s area 46) and no difference in right prefrontal regions. Comparison subjects also showed relatively greater activation in the left anterior cingulate gyrus (Brodmann’s area 32), left middle frontal gyrus (Brodmann’s areas 8 and 9), left mesial temporal lobe, and right thalamus. The between-group comparison of values for neuroleptic-naive and medicated patients revealed a difference in the right occipital cortex (Brodmann’s area 18).
Correlations with performance
To assess the relationship between task-related activation and performance, recognition discriminability was correlated with changes in scaled pixel intensity for the 20 comparison subjects and 19 patients with performance data. Correlational results for the difference in CBF between word encoding and the baseline motor task are illustrated in Figure 3. The largest correlation for comparison subjects was in the left inferior frontal gyrus (x, y, z=–33, 34, 12; Brodmann’s area 46) (r=0.81, df=19, p=0.0002). In patients, the largest correlations were in the left inferior temporal gyrus (x, y, z=–52, –53, –12; Brodmann’s area 37) (r=0.73, df=18, p=0.002), left inferior parietal gyrus (x, y, z=–41, –52, 29; Brodmann’s area 40) (r=0.69, df=18, p=0.003), left superior frontal gyrus (x, y, z=–22, 14, 57; Brodmann’s area 6) (r=0.74, df=18, p=0.002), and right middle frontal gyrus (x, y, z=31, 4, 35; Brodmann’s area 9) (r=0.73, df=18, p=0.002).
Performance correlations for the recognition condition are illustrated in Figure 4. Again, comparison subjects produced the largest correlation in the left inferior frontal gyrus (x, y, z=–31, 34, 16; Brodmann’s area 46) (r=0.78, df=19, p=0.0004). The largest correlations in patients were in the left hemisphere motor area (x, y, z=–22, –25, 41; Brodmann’s area 4) (r=0.77, df=18, p=0.0009) and supplementary motor area (x, y, z=–12, 22, 54; Brodmann’s area 6) (r=0.71, df=18, p=0.002).
Discussion
The current study found evidence of reduced left hemisphere frontotemporal activation in patients with schizophrenia during episodic memory encoding and retrieval. Differences between patients and healthy subjects in CBF during encoding were centered in the left inferior prefrontal gyrus and extended to the left middle frontal gyrus, superior frontal gyrus, and left superior temporal cortex. Smaller activations in the left inferior and middle frontal gyrus of patients were also seen during recognition, with additional activation impairments found in the left anterior cingulate, left mesial temporal lobe, and right thalamus. There were no group differences during recognition in a right prefrontal region believed to mediate episodic retrieval (66). Greater left inferior prefrontal activation during encoding and recognition was correlated with better task performance only in comparison subjects. These group differences did not appear to be due to medication status or to reduced power to detect activation effects in the patient group.
These results underscore the importance of the dorsolateral portion of the left inferior frontal gyrus (Brodmann’s areas 45 and 46) to episodic memory and to the pathophysiology of schizophrenia (26, 67–69). As in our previous study (38), this region was activated during word encoding and recognition in healthy comparison subjects. This finding is consistent with modifications (5–8) of the hemispheric encoding/retrieval asymmetry model (4), clarifying that, although the right prefrontal cortex appears specific to episodic retrieval, left prefrontal regions are involved in both stages of episodic memory processing. Activation of this region during multiple stages has led to the suggestion that the region is responsible for maintaining a verbal representation of word stimuli to permit encoding and subsequent retrieval (5, 6).
Differences between patients with schizophrenia and healthy subjects in left inferior prefrontal activation might, therefore, reflect patients’ difficulties with active maintenance and manipulation of verbal representations caused by impaired working memory (70–72). This explanation gains support from findings of patient abnormalities in left prefrontal activation during “n-back” working memory tasks despite normal levels of task performance (65, 73). Statistical control of working memory deficits has also been shown to eliminate free recall deficits in patients with schizophrenia (74). However, the ability of patients to perform the recognition task suggests that their working memory abilities were sufficient to maintain the words long enough to permit encoding.
An alternative explanation is that patients processed words on a more superficial level (75) and did not use semantic information to organize encoding and retrieval. Evidence of normal left prefrontal activation in patients during semantic decision tasks (76) suggests that semantic processing at the lexical level was intact and that prefrontal abnormalities more likely reflected impaired strategic processes caused by executive difficulties (69, 77–79). This conclusion is supported by the finding that although patients tend to encode words superficially, when provided with cues (2, 3), they can successfully use semantic information to improve performance (36, 79, 80). Imaging data have also shown that left prefrontal activation is maximized when healthy subjects process word associations semantically (17) and that the degree of left prefrontal activation and metabolism in patients is related to their use of semantic clustering versus serial order strategies (32, 33). Because encoding strategies were not systematically controlled or measured in the current study, further testing of this hypothesis will require future studies employing depth-of-processing (81) imaging paradigms (82, 83).
Contrary to our hypothesis, prefrontal dysfunction was also prominent during recognition and extended to the left anterior cingulate, left mesial temporal lobe, and right thalamus. This is potentially an incongruous finding, given that we found that patient recognition performance was relatively intact, as has been found in previous studies (36). However, Danion et al. (37) demonstrated how recognition tasks can be performed despite a shallow level of processing. In a study of recognition paradigms, these investigators proposed that nonrelational tasks such as the current forced-choice paradigm can be successfully performed on the basis of feelings of familiarity (noetic awareness) rather than conscious recollection (autonoetic awareness). If the task is altered to require conscious recollection (e.g., probe of source memory), then frontal brain potentials increase (84) and patient recognition performance becomes impaired (37, 85).
The current correlational findings support the hypothesis that patients and comparison subjects were engaged in different levels of information processing and memory awareness. The strongest correlation in comparison subjects was in the left inferior prefrontal gyrus, consistent with high-level organizational processing during encoding and autonoetic awareness during recognition. In contrast, correlations in patients were more posterior and included a basal temporal area involved in linguistic processing (86). The patient correlations might reflect operation of a compensatory network used to facilitate language processing (76).
Finally, lack of group differences in right prefrontal function suggest that retrieval mechanisms were intact, permitting successful performance regardless of level of memory awareness. Future imaging studies contrasting free recall and recognition paradigms with and without source memory probes would help to test these speculative hypotheses.
Reduced patient activation of the anterior cingulate, mesial temporal lobe, and thalamic regions during the Penn Word Recognition Test should be viewed with caution given the relatively low thresholds used for between-group analyses and the fact that comparison subjects did not show mesial temporal lobe and thalamic activation during the within-group analysis. Nevertheless, patient deficits in anterior cingulate activity have been documented in a variety of imaging paradigms (25, 78, 87–90) and attributed to problems in attention (27) or performance monitoring (91). Patient deficits in mesial temporal lobe function have been reported during episodic retrieval (30, 31) and attributed to impaired conscious recollection. Abnormal thalamic function has been documented (25, 27, 92) and discussed in relation to information processing abnormalities (93). These explanations might all be applicable to the current results. However, there are insufficient data to test these multiple hypotheses, and a more parsimonious explanation may be that an abnormality in the functional connectivity of the prefrontal cortex with temporal-limbic regions accounted for the multifocal abnormalities (26, 94).
The current study suffered from several limitations. In measuring task effects we chose to contrast encoding and retrieval with a low-level motor baseline task rather than performing serial subtractions of increasingly complex reference conditions (95). Because of this, we failed to control for linguistic processes and other nonmemory functions that might complicate interpretation. The choice of appropriate reference tasks is a problematic issue in the field, and different arguments have been raised in favor of active versus passive reference conditions (96). The rationale for the current design was twofold. First, performing serial subtractions of increasingly complex reference tasks has the potential limitation of confounding the interpretation of activation effects if interactions occur between reference tasks at each stage of the serial subtraction procedure (97). Second, the combination of relatively long scan times (4.2-minute scans) and long (15-minute) decay periods limited the number of scans because of participant fatigue. These time constraints made use of serial subtraction procedures less feasible. Therefore, in the motor task we followed the recommended procedure of establishing similar levels of sensorimotor activation between groups to provide an internal standard to help ensure that subsequent group differences in cognitive effects were not artifactual (65).
A second limitation is that more than half of the patients were receiving medication at the time of testing. All patients were stable outpatients, and the neuroleptic-naive patients were tested during their first episode. For the stable patients who were receiving medication, discontinuing that medication would have exacerbated symptoms and hindered performance. The clinical benefit of medication was reflected in the fact that patients receiving medication had less severe symptoms. However, there is no evidence that long-term neuroleptic exposure in schizophrenia affects CBF in the prefrontal or mesial temporal lobe regions (98, 99). Current results support this: there were few regional CBF differences between medicated and unmedicated patients, and no differences occurred in hypothesized regions. There was also no effect of medication on task performance, notwithstanding recent reports that atypical neuroleptics improve memory performance (100). However, relatively few patients were receiving atypical compounds, and a larger-scale investigation of atypical medication effects on performance and blood flow is warranted.
![]() |
Presented in part at the seventh International Congress on Schizophrenia Research, Santa Fe, N.Mex., April 15–17, 1999. Received April 4, 2000; revision received Jan. 23, 2001; accepted Feb. 1, 2001. From the Department of Psychiatry, University of Pennsylvania. Address reprint requests to Dr. Ragland, Department of Psychiatry, University of Pennsylvania, 10th Floor Gates Building/HUP, 3400 Spruce St., Philadelphia, PA 19104-4283; [email protected] (e-mail). Dr. Raz and Dr. Smith died in 2000.Supported by the EJLB Foundation and by NIMH grants MH-19112, MH-00586, MH-48539, MH-43880, and M01RR040. The authors thank the staff of the University of Pennsylvania Health System PET Center for assistance in data collection.
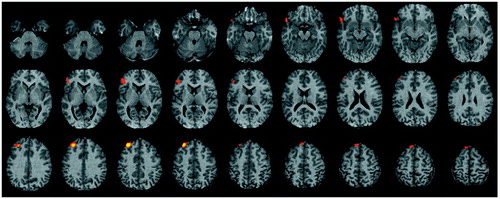
Figure 1. Brain Imagesa Showing Differencesb Between 23 Healthy Comparison Subjects and 23 Patients With Schizophrenia in Change in Regional Cerebral Blood Flow Between Word Encoding and Baseline Motor Task
aStatistical parametric maps are displayed on an axial 4-mm MRI standardized into Talairach space extending in the z direction from –28 to 56. Images are in neurological convention (left is left). Colored areas exceed an uncorrected p value of 0.005 with 50 or more contiguous voxels activated.
bValues for comparison subjects minus values for patients. Maxima of activation differences noted in left inferior frontal gyrus, middle frontal gyrus, superior frontal gyrus, and left inferior temporal gyrus.
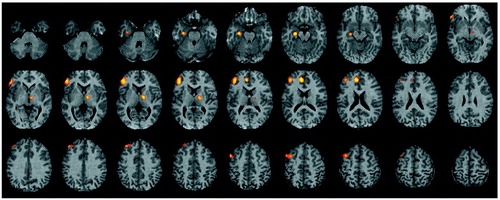
Figure 2. Brain Imagesa Showing Differencesb Between 23 Healthy Comparison Subjects and 23 Patients With Schizophrenia in Change in Regional Cerebral Blood Flow Between Word Recognition and Baseline Motor Task
aStatistical parametric maps are displayed as in Figure 1.
bValues for comparison subjects minus values for patients. Maxima of activation differences noted in left inferior frontal gyrus, middle frontal gyrus, left parahippocampal gyrus, left anterior cingulate, and right thalamus.
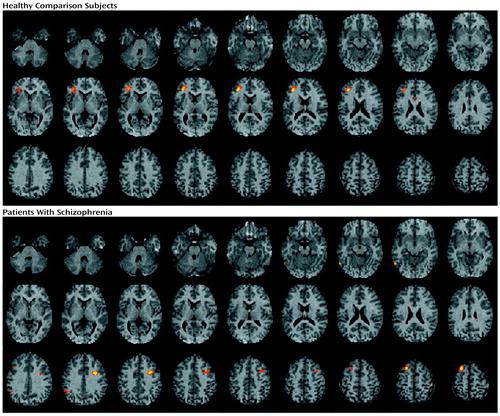
Figure 3. Brain Imagesa Showing Pearson Correlationsb Between Target Discriminability and Change in Regional Cerebral Blood Flow Between Word Encoding and Baseline Motor Task for 20 Healthy Comparison Subjects and 19 Patients With Schizophreniac
aStatistical parametric maps are displayed as in Figure 1.
bColored areas exceed an r value of 0.60.
cFor comparison subjects, the largest correlation is noted in the left inferior frontal gyrus; for patients, the largest correlations are noted in the left inferior temporal gyrus, left inferior parietal gyrus, left superior frontal gyrus, and right middle frontal gyrus.
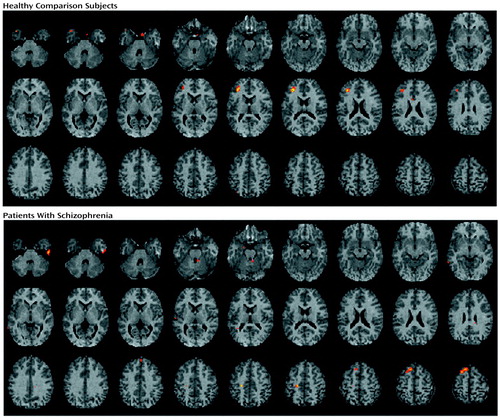
Figure 4. Brain Imagesa Showing Pearson Correlationsb Between Target Discriminability and Change in Regional Cerebral Blood Flow Between Word Recognition and Baseline Motor Task for 20 Healthy Comparison Subjects and 19 Patients With Schizophreniac
aStatistical parametric maps are displayed as in Figure 1.
bColored areas exceed an r value of 0.60.
cFor comparison subjects, the largest correlation is noted in the left inferior frontal gyrus; for patients, the largest correlations are noted in left hemisphere motor and supplementary motor cortices.
1. Tulving E: Elements of Episodic Memory. Oxford, UK, Oxford University Press, 1983Google Scholar
2. Heinrichs RW, Awad AG: Neurocognitive subtypes of chronic schizophrenia. Schizophr Res 1993; 9:49-58Crossref, Medline, Google Scholar
3. Paulsen JS, Heaton RK, Sadek JR, Perry W, Delis DC, Braff D, Kuck J, Zisook S, Jeste DV: The nature of learning and memory impairments in schizophrenia. J Int Neuropsychol Soc 1995; 1:88-99Crossref, Medline, Google Scholar
4. Tulving E, Kapur S, Craik FIM, Moscovitch M, Houle S: Hemispheric encoding/retrieval asymmetry in episodic memory: positron emission tomography findings. Proc Natl Acad Sci USA 1994; 91:2016-2020Google Scholar
5. Buckner RL: Beyond HERA: contributions of specific prefrontal brain areas to long-term memory retrieval. Psychon Bull Rev 1996; 3:149-158Crossref, Medline, Google Scholar
6. Buckner RL, Petersen SE: What does neuroimaging tell us about the role of prefrontal cortex in memory retrieval? Semin Neurosci 1996; 8:47-55Crossref, Google Scholar
7. Petrides M, Alivisatos B, Evans AC: Functional activation of the human ventrolateral frontal cortex during the mnemonic retrieval of verbal information. Proc Natl Acad Sci USA 1995; 92:5803-5807Google Scholar
8. Nyberg L: Mapping episodic memory. Behav Brain Res 1998; 90:107-114Crossref, Medline, Google Scholar
9. Gabrieli JD, Brewer JB, Desmond JE, Glover GH: Separate neural bases of two fundamental memory processes in the human medial temporal lobe. Science 1997; 276:264-266Crossref, Medline, Google Scholar
10. Lepage M, Habib R, Tulving E: Hippocampal PET activations of memory encoding and retrieval: the HIPER model. Hippocampus 1998; 8:313-322Crossref, Medline, Google Scholar
11. Roland PE, Gulyas B: Visual memory, visual imagery, and visual recognition of large field patterns by the human brain: functional anatomy by positron emission tomography. Cereb Cortex 1995; 1:79-93Crossref, Google Scholar
12. Schacter DL, Wagner AD: Medial temporal lobe activations in fMRI and PET studies of episodic encoding and retrieval. Hippocampus 1998; 9:7-24Crossref, Google Scholar
13. Martin A: Automatic activation of the medial temporal lobe during encoding: lateralized influences of meaning and novelty. Hippocampus 1999; 9:62-70Crossref, Medline, Google Scholar
14. Schacter DL, Alpert NM, Savage CR, Rauch SL, Albert MS: Conscious recollection and the human hippocampal formation: evidence from positron emission tomography. Proc Natl Acad Sci USA 1996; 93:321-325Crossref, Medline, Google Scholar
15. Nyberg L, McIntosh AR, Houle S, Nilsson LG, Tulving E: Activation of medial temporal structures during episodic memory retrieval. Nature 1996; 380:715-717Crossref, Medline, Google Scholar
16. Tulving E, Markowitsch HJ, Craik FIM, Habib R, Houle S: Novelty and familiarity activations in PET studies of memory encoding and retrieval. Cereb Cortex 1996; 6:71-79Crossref, Medline, Google Scholar
17. Dolan RJ, Fletcher PC: Dissociating prefrontal and hippocampal function in episodic memory encoding. Nature 1997; 388:582-585Crossref, Medline, Google Scholar
18. Fujii T, Okuda J, Kawashima R, Yamadori A, Fukatsu R, Suzuki K, Ito M, Goto R, Fukuda H: Different roles of the left and right parahippocampal regions in verbal recognition: a PET study. Neuroreport 1997; 8:1113-1117Google Scholar
19. Martin A, Wiggs CL, Weisberg J: Modulation of human medial temporal lobe activity by form, meaning, and experience. Hippocampus 1997; 7:587-593Crossref, Medline, Google Scholar
20. Dolan RJ, Fletcher PC, McKenna P, Friston KJ, Frith CD: Abnormal neural integration related to cognition in schizophrenia. Acta Psychiatr Scand Suppl 1999; 395:58-67Crossref, Medline, Google Scholar
21. Gur RE: Functional brain-imaging studies in schizophrenia, in Psychopharmacology: The Fourth Generation of Progress. Edited by Bloom FE, Kupfer DJ. Philadelphia, Raven Press, 1995, pp 1185-1192Google Scholar
22. Liddle PF: Functional imaging: schizophrenia. Br Med Bull 1996; 52:486-494Crossref, Medline, Google Scholar
23. Wood FB, Flowers DL: Hypofrontal vs hypo-Sylvian blood flow in schizophrenia. Schizophr Bull 1990; 16:413-424Crossref, Medline, Google Scholar
24. Gur RE, Jaggi J, Shtasel D, Ragland JD, Gur RC: Cerebral blood flow in schizophrenia: effects of memory processing on regional activation. Biol Psychiatry 1994; 35:3-15Crossref, Medline, Google Scholar
25. Andreasen NC, O’Leary DS, Cizadlo T, Arndt S, Rezai K, Boles Ponto LL, Watkins GL, Hichwa RD: Schizophrenia and cognitive dysmetria: a positron-emission tomography study of dysfunctional prefrontal-thalamic-cerebellar circuitry. Proc Natl Acad Sci USA 1996; 93:9985-9990Google Scholar
26. Andreasen NC, O’Leary DS, Flaum M, Nopoulos P, Watkins GL, Boles Ponto LL, Hichwa RD: Hypofrontality in schizophrenia: distributed dysfunctional circuits in neuroleptic-naive patients. Lancet 1997; 349:1730-1734Google Scholar
27. Crespo-Facorro B, Paradiso S, Andreasen NC, O’Leary DS, Watkins GL, Boles Ponto LL, Hichwa RD: Recalling word lists reveals “cognitive dysmetria” in schizophrenia: a positron emission tomography study. Am J Psychiatry 1999; 156:386-392Abstract, Google Scholar
28. Ganguli R, Carter C, Mintun M, Brar J, Becke J, Sarma R, Nichols T, Bennington E: PET brain mapping study of auditory verbal supraspan memory versus visual fixation in schizophrenia. Biol Psychiatry 1997; 41:33-42Crossref, Medline, Google Scholar
29. Ragland JD, Gur RC, Glahn DC, Censits DM, Smith RJ, Lazarev MG, Alavi A, Gur RE: Fronto-temporal cerebral blood flow change during executive and declarative memory tasks in schizophrenia: a positron emission tomography study. Neuropsychology 1998; 12:399-413Crossref, Medline, Google Scholar
30. Heckers S, Rauch SL, Goff D, Savage CR, Schacter DL, Fischman AJ, Alpert NM: Impaired recruitment of the hippocampus during conscious recollection in schizophrenia. Nat Neurosci 1998; 1:318-323Crossref, Medline, Google Scholar
31. Heckers S, Goff D, Schacter DL, Savage CR, Fischman AJ, Alpert NM, Rauch SL: Functional imaging of memory retrieval in deficit vs nondeficit schizophrenia. Arch Gen Psychiatry 1999; 56:1117-1123Google Scholar
32. Nohara S, Suzuki M, Kurach M, Yamashita I, Matsui M, Seto H, Saitoh O: Neural correlates of memory organization deficits in schizophrenia: a single photon emission computed tomography study with 99mTc-ethyl-cysteinate dimer during a verbal learning task. Schizophr Res 2000; 42:209-222Crossref, Medline, Google Scholar
33. Hazlet EA, Buchsbaum MS, Jeu LA, Nenadic I, Fleischman MB, Shihabuddin L, Haznedar M, Harvey PD: Hypofrontality in unmedicated schizophrenia patients studied with PET during performance of a serial verbal learning task. Schizophr Res 2000; 43:33-46Crossref, Medline, Google Scholar
34. Fletcher PC, McKenna PJ, Frith CD, Grasby PM, Friston KJ, Dolan RJ: Brain activations in schizophrenia during a graded memory task studied with functional neuroimaging. Arch Gen Psychiatry 1998; 55:1001-1008Google Scholar
35. Gold JM, Randolf C, Carpenter CJ, Goldberg TE, Weinberger DR: Forms of memory failure in schizophrenia. J Abnorm Psychol 1992; 101:487-494Crossref, Medline, Google Scholar
36. Calev A: Recall and recognition in chronic non-demented schizophrenics: use of matched tasks. J Abnorm Psychol 1984; 93:172-177Crossref, Medline, Google Scholar
37. Danion J-M, Rizzo L, Bruant A: Functional mechanisms underlying impaired recognition memory and conscious awareness in patients with schizophrenia. Arch Gen Psychiatry 1999; 56:639-644Crossref, Medline, Google Scholar
38. Ragland JD, Gur RC, Lazarev MG, Smith RJ, Schroeder L, Raz J, Turetsky BI, Alavi A, Gur RE: Hemispheric activation of anterior and inferior prefrontal cortex during verbal encoding and recognition. Neuroimage 2000; 11:624-633Crossref, Medline, Google Scholar
39. Resnick SM: Matching for education in studies of schizophrenia (letter). Arch Gen Psychiatry 1992; 49:246Crossref, Medline, Google Scholar
40. First MB, Spitzer RL, Gibbon M, Williams JBW: Structured Clinical Interview for DSM-IV Axis I Disorders, Patient Edition (SCID-P), version 2. New York, New York State Psychiatric Institute, Biometrics Research, 1996Google Scholar
41. Gur RE, Mozley PD, Resnick SM, Levick S, Erwin R, Saykin AJ, Gur RC: Relations among clinical scales in schizophrenia. Am J Psychiatry 1991; 148:472-478Link, Google Scholar
42. First MB, Spitzer RL, Gibbon M, Williams JB: Structured Clinical Interview for DSM-IV Axis I Disorders—Non-Patient Edition (SCID-I/NP), version 2.0. New York, New York State Psychiatric Institute, Biometrics Research, 1996Google Scholar
43. Shtasel DL, Gur RE, Mozley PD, Richards J, Taleff MM, Heimberg C, Gallacher F, Gur RC: Volunteers for biomedical research: recruitment and screening of normal controls. Arch Gen Psychiatry 1991; 48:1022-1025Google Scholar
44. Andreasen NC: Modified Scale for the Assessment of Negative Symptoms (SANS). Iowa City, University of Iowa, 1984Google Scholar
45. Andreasen NC: Scale for the Assessment of Positive Symptoms (SAPS). Iowa City, University of Iowa, 1984Google Scholar
46. Overall JE, Gorham DR: The Brief Psychiatric Rating Scale. J Operational Psychiatry 1980; 11:46-64Google Scholar
47. Chute DL, Westall RF: Power Laboratory. Devon, Pa, MacLaboratory, 1997Google Scholar
48. Gur RC, Gur RE, Obrist WD, Hungerbuhler JP, Younkin D, Rosen AD, Skolnick BE, Reivich M: Sex and handedness differences in cerebral blood flow during rest and cognitive activity. Science 1982; 217:659-661Crossref, Medline, Google Scholar
49. Gur RC, Jaggi JL, Ragland JD, Resnick SM, Shtasel D, Muenz L, Gur RE: Effects of memory processing on regional brain activation: cerebral blood flow in normal subjects. Int J Neurosci 1993; 72:31-44Crossref, Medline, Google Scholar
50. Gur RC, Ragland JD, Mozley LH, Mozley PD, Smith R, Alavi A, Bilker W, Gur RE: Lateralized changes in regional cerebral blood flow during performance of verbal and facial recognition tasks: correlations with performance and “effort.” Brain Cogn 1997; 33:388-414Crossref, Medline, Google Scholar
51. Paivio A, Yuille JC, Madigan SA: Concreteness, imagery, and meaningfulness values for 925 nouns. J Exp Psychol Monograph Suppl 1968; 76:1-25Crossref, Medline, Google Scholar
52. Snodgrass JG, Corwin J: Pragmatics of measuring recognition memory: applications to dementia and amnesia. J Exp Psychol Gen 1988; 117:34-50Crossref, Medline, Google Scholar
53. Smith RJ, Karp JS, Muehllehner G: The countrate performance of the volume imaging PENN PET scanner. IEEE Trans Med Imaging 1994; 13:610-618Crossref, Medline, Google Scholar
54. Karp JS, Kinahan PE, Muehllehner G: Effect of increased axial field of view on the performance of a volume PET scanner. IEEE Trans Med Imaging 1993; 12:299-306Crossref, Medline, Google Scholar
55. Lammertsma AA, Frackowiak RSJ, Lenzi GL, Heather JD, Pozzilli C, Jones T: Accuracy of the oxygen-15 steady state technique for measuring rCBF and rCMR(O2): tracer modeling, statistics and spatial sampling. J Cereb Blood Flow Metab 1981; 1:S3-S4Google Scholar
56. Smith RJ, Shao L, Freifelder R, Karp JS, Ragland JD: Quantitative measurements of cerebral blood flow in volume imaging PET scanners. IEEE Transactions on Nuclear Sci 1995; 42:1018-1023Google Scholar
57. Friston KJ, Ashburner J, Frith CD, Poline J-B, Heather JD, Frackowiak RSJ: Spatial registration and normalization of images. Hum Brain Mapp 1995; 2:165-189Crossref, Google Scholar
58. Friston KJ, Holmes AP, Worsley KJ, Poline J-B, Frith CD, Frackowiak RSJ: Statistical parametric mapping in functional imaging: a general linear approach. Hum Brain Mapp 1995; 2:189-210Crossref, Google Scholar
59. Talairach J, Tournoux P: Co-Planar Stereotaxic Atlas of the Human Brain: Three-Dimensional Proportional System. New York, Thieme Medical, 1988Google Scholar
60. MEDx version 3.2: Multimodality Radiological Imaging Processing for UNIX Work Stations: Users Guide. Sterling, Va, Sensory Systems, 1998Google Scholar
61. Kramer JH, Delis DC, Daniel M: Sex differences in verbal learning. J Clin Psychol 1988; 44:907-915Crossref, Google Scholar
62. Bogerts BH: The neuropathology of schizophrenia. Schizophr Bull 1993; 19:68-75Crossref, Google Scholar
63. Buckley PF, Friedman L, Wu D, Lai S, Meltzer HY, Haacke EM, Miller D, Lewis JS: Functional magnetic resonance imaging in schizophrenia: initial methodology and evaluation of the motor cortex. Psychiatry Res Neuroimaging 1997; 74:13-23Crossref, Medline, Google Scholar
64. Mattay VS, Callicott JH, Bertolino A, Santha A, Tallent K, Frank J, Weinberger DR: Sensorimotor cortex lateralization is anomalous in patients with schizophrenia: a whole brain fMRI study (letter). Neuroimage 1997; 5(suppl):S23Google Scholar
65. Callicott JH, Ramsey NF, Tallent BA, Bertolino A, Knable MB, Coppola R, Goldberg T, van Gelderen P, Mattay VS, Frank JA, Moonen CTW, Weinberger DR: Functional magnetic resonance imaging brain mapping in psychiatry: methodological issues illustrated in a study of working memory in schizophrenia. Neuropsychopharmacology 1998; 18:186-196Crossref, Medline, Google Scholar
66. Desgranges B, Baron J, Eustache F: The functional neuroanatomy of episodic memory: the role of the frontal lobes, the hippocampal formation, and other areas. Neuroimage 1998; 8:198-213Crossref, Medline, Google Scholar
67. Goldberg TE, Weinberger DR, Berman KF, Pliskin NH, Podd MH: Further evidence for dementia of the prefrontal type in schizophrenia? a controlled study of teaching the Wisconsin Card Sorting Test. Arch Gen Psychiatry 1987; 44:1008-1014Google Scholar
68. Pearlson GD, Petty RG, Ross CA, Tien AY: Schizophrenia: a disease of heteromodal association cortex? Neuropsychopharmacology 1996; 14:1-7Crossref, Medline, Google Scholar
69. Weinberger DR, Berman KF, Zec RF: Physiologic dysfunction of dorsolateral prefrontal cortex in schizophrenia. Arch Gen Psychiatry 1986; 43:114-124Crossref, Medline, Google Scholar
70. Carter CS, Robertson LC, Nordahl TE, Kraft L, Chaderjian M, Oshora-Celaya L: Spatial working memory deficits and their relationship to negative symptoms in unmedicated schizophrenia patients. Biol Psychiatry 1996; 40:930-932Crossref, Medline, Google Scholar
71. Park S, Holzman PS: Schizophrenics show spatial working memory deficits. Arch Gen Psychiatry 1992; 49:975-982Crossref, Medline, Google Scholar
72. Stevens AA, Goldman-Rakic PS, Gore JC, Fulbright RK, Wexler BE: Cortical dysfunction in schizophrenia during auditory word and tone working memory demonstrated by functional magnetic resonance imaging. Arch Gen Psychiatry 1998; 55:1097-1103Google Scholar
73. Carter CS, Perlstein W, Ganguli R, Brar J, Mintun M, Cohen JD: Functional hypofrontality and working memory dysfunction in schizophrenia. Am J Psychiatry 1998; 155:1285-1287Google Scholar
74. Stone M, Gabrieli JDE, Stebbins GT, Sullivan EV: Working and strategic memory deficits in schizophrenia. Neuropsychology 1998; 12:278-288Crossref, Medline, Google Scholar
75. Brebion G, Amador X, Smith MJ, Gorman JM: Mechanisms underlying memory impairment in schizophrenia. Psychol Med 1997; 27:383-393Crossref, Medline, Google Scholar
76. Curtis VA, Bullmore ET, Morris RG, Brammer MJ, Williams SCR, Simmons A, Sharma T, Murray RM, McGuire PK: Attenuated frontal activation in schizophrenia may be task dependent. Schizophr Res 1999; 37:35-44Crossref, Medline, Google Scholar
77. Weinberger DR, Gallhofer B: Cognitive dysfunction in schizophrenia. Int Clin Psychopharmacol 1997; 12(suppl 4):S29-S36Google Scholar
78. Andreasen NC, Rezai K, Alliger R, Swayze VW II, Flaum M, Kirchner P, Cohen G, O’Leary DS: Hypofrontality in neuroleptic-naive patients and in patients with chronic schizophrenia: assessment with Xenon 133 single-photon emission computed tomography and the Tower of London. Arch Gen Psychiatry 1992; 49:943-958Crossref, Medline, Google Scholar
79. McClain L: Encoding and retrieval in schizophrenics’ free recall. J Nerv Ment Dis 1983; 171:471-479Crossref, Medline, Google Scholar
80. Iddon JL, McKenna PJ, Sahakian BJ, Robbins TW: Impaired generation and use of strategy in schizophrenia: evidence from visuospatial and verbal tasks. Psychol Med 1998; 28:1049-1062Google Scholar
81. Craik FIM, Lockhart RS: Levels of processing: a framework for memory research. J Verb Learn Verb Behav 1972; 11:671-684Crossref, Google Scholar
82. Buckner RL, Koutstaal W, Schacter DL, Wagner AD, Rosen BF: Functional-anatomic study of episodic retrieval using fMRI. Neuroimage 1998; 7:151-162Crossref, Medline, Google Scholar
83. Demb JB, Desmond JE, Wagner AD, Vaidya CJ, Glover GH, Gabrieli JDE: Semantic encoding and retrieval in the left inferior prefrontal cortex: a functional MRI study of task difficulty and process specificity. J Neurosci 1995; 15:5870-5878Google Scholar
84. Ranganath C, Paller KA: Neural correlates of memory retrieval and evaluation. Brain Res Cogn Brain Res 2000; 9:209-222Crossref, Medline, Google Scholar
85. Huron C, Danion JM, Giacomoni F, Grange D, Robert P, Rizzo L: Impairment of recognition memory with, but not without, conscious recollection in schizophrenia. Am J Psychiatry 1995; 152:1737-1742Google Scholar
86. Buchel C, Price C, Friston K: A multimodal language region in the ventral vision pathway. Nature 1998; 394:274-277Crossref, Medline, Google Scholar
87. Siegel BV Jr, Buchsbaum MS, Bunney WE Jr, Gottschalk LA, Haier RJ, Lohr JB, Lottenberg S, Najafi A, Nuechterlein KH, Potkin SG: Cortical-striatal-thalamic circuits and brain glucose metabolic activity in 70 unmedicated male schizophrenic patients. Am J Psychiatry 1993; 150:1325-1336Google Scholar
88. Tamminga CA, Thaker GK, Buchanan R, Kirkpatrick B, Alphs LD, Chase TN, Carpenter WT: Limbic system abnormalities identified in schizophrenia using positron emission tomography with fluorodeoxyglucose and neocortical alterations with deficit syndrome. Arch Gen Psychiatry 1992; 49:522-530Crossref, Medline, Google Scholar
89. Dolan RJ, Fletcher P, Frith CD, Friston KJ, Frackowiak RS, Grasby PM: Dopaminergic modulation of impaired cognitive activation in the anterior cingulate cortex in schizophrenia. Nature 1995; 378:180-182Crossref, Medline, Google Scholar
90. Haznedar MM, Buchsbaum MS, Luu C, Hazlett EA, Siegel BV Jr, Lohr J, Wu J, Haier RJ, Bunney WE Jr: Decreased anterior cingulate gyrus metabolic rate in schizophrenia. Am J Psychiatry 1997; 154:682-684Link, Google Scholar
91. Carter CS, Botvinick MM, Cohen JD: The role of the anterior cingulate cortex in executive processes of cognition. Rev Neurosci 1999; 10:49-57Crossref, Medline, Google Scholar
92. Buchsbaum MS, Someya T, Teng CY, Abel L, Chin S, Najafi A, Haier RJ, Wu J, Bunney WE Jr: PET and MRI of the thalamus in never-medicated patients with schizophrenia. Am J Psychiatry 1996; 153:191-199Link, Google Scholar
93. Braff DL: Information processing and attentional dysfunctions in schizophrenia. Schizophr Bull 1993; 19:233-259Crossref, Medline, Google Scholar
94. Frith CD: The Cognitive Neuropsychology of Schizophrenia. Hove, UK, Lawrence Erlbaum Associates, 1992Google Scholar
95. Posner MI, Petersen SE, Fox PT, Raichle ME: Localization of cognitive operations in the human brain. Science 1988; 240:1627-1631Google Scholar
96. Gur RC, Erwin RJ, Gur RE: Neurobehavioral probes for physiologic neuroimaging studies. Arch Gen Psychiatry 1992; 49:409-414Crossref, Medline, Google Scholar
97. Friston KJ, Price CJ, Fletcher P, Moore C, Frackowiak RSJ, Dolan RJ: The trouble with cognitive subtraction. Neuroimage 1996; 4:97-104Crossref, Medline, Google Scholar
98. Goldberg TE, Weinberger DR: Effects of neuroleptic medications on the cognition of patients with schizophrenia: a review of recent studies. J Clin Psychiatry 1996; 57(suppl 9):62-65Google Scholar
99. Miller DD, Rezai K, Alliger R, Andreasen NC: The effect of antipsychotic medication on relative cerebral blood perfusion in schizophrenia: assessment with technetium-99m hexamethyl-propyleneamine oxime single photon emission computed tomography. Biol Psychiatry 1997; 41:550-559Crossref, Medline, Google Scholar
100. Kern RS, Green MF, Marshall BD Jr, Wirshing WC, Wirshing D, McGurk SR, Marder SR, Mintz J: Risperidone versus haloperidol on secondary memory: can newer medications aid learning? Schizophr Bull 1999; 25:223-232Crossref, Medline, Google Scholar