Selective Relationship Between Prefrontal N-Acetylaspartate Measures and Negative Symptoms in Schizophrenia
Abstract
OBJECTIVE: Certain cognitive, behavioral, and emotional deficits (so-called negative symptoms) in patients with schizophrenia have often been attributed to prefrontal cortical pathology, but direct evidence for a relationship between prefrontal neuronal pathology and negative symptoms has been lacking. The authors hypothesized that an in vivo measure of prefrontal neuronal pathology (N-acetylaspartate [NAA] levels) in patients with schizophrenia would predict negative symptoms. METHOD: Proton magnetic resonance spectroscopic imaging (1H-MRSI) and rating scales for negative and positive symptoms were used to study 36 patients with schizophrenia. Magnetic resonance spectra were analyzed as metabolite ratios, and parametric correlations were performed. RESULTS: A regionally selective negative correlation was found between prefrontal NAA-creatine ratio and negative symptom ratings in this group of patients with schizophrenia. CONCLUSIONS: Lower prefrontal NAA—and by inference greater neuronal pathology—predicted more severe negative symptoms in patients with schizophrenia. These data demonstrate a relationship between an intraneuronal measure of dorsolateral prefrontal cortex integrity and negative symptoms in vivo and represent further evidence for the involvement of the dorsolateral prefrontal cortex in negative symptoms associated with schizophrenia.
The early course of schizophrenia is often dominated by florid psychotic symptoms such as hallucinations or delusions (positive symptoms), but persistent difficulties with emotional expression (flattened affect), social communication (alogia), and motivation (avolition) often become the most debilitating aspects of schizophrenia as time passes (1–3). These latter, so-called negative symptoms are often most prominent in patients with schizophrenia who have poorer premorbid adjustment, earlier onset, and greater illness chronicity (4). Negative symptoms remain relatively unresponsive to most antipsychotic medications (perhaps even to atypical drugs such as clozapine) (5). Furthermore, negative symptoms interfere with psychosocial rehabilitation efforts and often persist unchanged (if not worsening) throughout the course of the illness (6).
The persistent, invariant quality of negative symptoms might lead one to predict that their etiopathogenesis might be easier to identify than positive symptoms, which often vary unpredictably in quality, duration, and amount. Because the negative symptoms in patients with schizophrenia are phenomenologically similar to the disruptions of affect, cognition, and motivation in neurological patients with frontal lobe lesions (7, 8), it has been speculated that these symptoms arise from dysfunction of the frontal lobes, particularly the dorsolateral prefrontal cortex (9–11).
Previous attempts to correlate negative symptoms with indirect measures of cortical neuronal pathology—including structural imaging, neuropsychological tests of dorsolateral prefrontal cortex function, regional cerebral blood flow (rCBF), and evoked electrophysiological responses—have had some success (e.g., reference 12), but replication has been inconsistent. For example, frontal lobe structural measurements have been found to predict negative symptoms in some studies (9, 13–16), but not others (17–21).
Patients with schizophrenia show greater impairments on tests of cognition thought to rely on intact dorsolateral prefrontal cortex function, such as the Wisconsin Card Sorting Test (22–24) and working memory tasks (25), and these deficits have been associated with negative symptoms (26–30). Moreover, in some reports, reduced dorsolateral prefrontal cortex blood flow at rest also predicted greater negative symptoms (31�35). Neurophysiological measures of dorsolateral prefrontal cortex function have also been reported as abnormal in schizophrenia, including abnormal eye tracking (36), abnormal EEG patterns (37), and disruption of the normal coherence between the dorsolateral prefrontal cortex and other brain regions (38). A sizable functional neuroimaging literature, although at times contradictory (39), has largely reported reduced physiological capacity of the dorsolateral prefrontal cortex in response to cognitive challenge (e.g., references 40–44).
None of these indirect measures, however, speaks to the integrity of prefrontal neurons per se; the different findings might also reflect activity in prefrontal inputs. For example, Sabri et al. (35) found that although negative symptoms correlated with bilateral frontal rCBF, negative symptoms also correlated with bilateral temporal, cingulate, left parietal, basal ganglia, and thalamic rCBF at rest.
In contrast to the modest in vivo evidence for a relationship between negative symptoms and frontal lobe dysfunction, evidence of dorsolateral prefrontal cortex neuronal pathology is compelling. Postmortem neuropathological human studies have found prefrontal gray matter abnormalities, including reduced neuropil volume with increased neuronal density in the dorsolateral prefrontal cortex (45), decreased inhibitory input from prefrontal chandelier cells onto dorsolateral prefrontal cortex pyramidal neurons (46), and decreased abundance and activity of interneurons (47, 48). In both medicated and untreated patients with schizophrenia, studies using proton magnetic resonance spectroscopy (1H-MRS and 1H-MRSI) have repeatedly found evidence of dorsolateral prefrontal cortex neuronal pathology reflected in reduced concentrations of the intraneuronal chemical N-acetylaspartate (NAA) (49).
Based on 1H-MRSI and postmortem evidence of dorsolateral prefrontal cortex neuronal pathology in schizophrenia, we hypothesized that if negative symptoms arise from such pathology, NAA measures should correlate with negative symptom ratings. In particular, larger reductions in dorsolateral prefrontal cortex NAA, and by inference greater neuronal pathology, should predict more severe negative symptoms. Since it is possible with 1H-MRSI to obtain NAA from many regions of brain, one would not predict similar regional correlations between NAA and negative symptoms if the cellular basis of the symptoms were regionally selective.
Method
We studied 36 patients with schizophrenia diagnosed according to DSM-IV criteria. There were six women (five were right-handed) and 30 men (26 were right-handed) with a mean age of 34 years (SD=8). Patients were recruited from the inpatient wards of the National Institute of Mental Health (NIMH) at St. Elizabeths Neuropsychiatric Research Hospital, Washington, D.C., and the Warren Magnuson Clinical Center, Bethesda, Md. In addition, patients were studied as part of the NIMH Clinical Brain Disorders Branch Sibling Study of Schizophrenia, Bethesda, Md.
The majority of patients (30 of the 36) were receiving a stable dose of antipsychotic medication at the time of scanning. The six medication-free subjects had been off all medicines for a minimum of 14 days. The remaining, medicated patients were evenly divided between typical (N=15) and atypical (N=15) antipsychotic treatment, with no acute change in dose for at least 14 days before scanning. The patients studied were chosen from a larger group of patients with schizophrenia on the basis of the presence of dorsolateral prefrontal cortex regional NAA measures that were of high quality as well as ratings that were obtained immediately after scanning.
Before participating, all subjects underwent a diagnostic interview with the Structured Clinical Interview for DSM-IV (50). In addition, patients completed a screening questionnaire regarding past substance abuse and neurological illnesses followed by a full neurological examination and a structural magnetic resonance imaging (MRI) clinical examination. Exclusion criteria included history of active or recent (less than 6 months) substance abuse, history of clinically significant neurological illness, or abnormalities on MRI examination. After discussion of the risks and benefits of the MRI procedure and questioning to determine assent and understanding of the MRI procedure, all subjects gave written informed consent. The MRI protocol (91-M-0124) was approved by the institutional review board of the NIMH Intramural Program.
For a comparison group, we used our database of healthy subjects of similar age and sex. The comparison subjects were 28 women (27 were right-handed) and 45 men (34 were right-handed) men with a mean age of 32.2 (SD=8.1). The same inclusion and exclusion criteria were applied to all healthy comparison subjects.
Metabolite spectra from multiple brain regions were acquired by using 1H-MRSI as previously described (51). A 1.5-tesla GE Signa scanner (GE Medical Systems, Milwaukee) was used. The technique acquires four 15-mm-thick oblique axial slices (TR=2200 msec, TE=272 msec, voxel size=7.5 × 7.5 × 15 mm). All subjects included in the analysis were free from substantial movement artifact. Spectra were analyzed by using in-house software (A. Barnett) and then converted to ratios for further analysis. Regions of interest were then drawn by using T1-weighted scans acquired immediately after the 1H-MRSI acquisition with the group identity blinded as described previously (49). Region of interest analysis was used to quantify NAA, creatine (creatine plus phosphocreatine), and choline-containing compounds.
In addition to the dorsolateral prefrontal cortex, metabolite ratios were obtained from the orbitofrontal cortex, hippocampal area (inclusive of the amygdala), thalamus, putamen, anterior and posterior cingulate, superior temporal gyrus, prefrontal white matter, occipital cortex, and centrum semiovale for both patients and healthy comparison subjects. Due to the oblique angle of acquisition chosen to maximize coverage of the hippocampal area, the parietal cortex was not imaged. Furthermore, where metabolite ratios could not reliably be determined, they were not entered into the statistical analyses (thus, the numbers are reported separately for all correlations in addition to the p values).
Ratios of NAA to creatine, NAA to choline-containing compounds, and choline-containing compounds to creatine were used for the correlation analysis. To determine if there were regional abnormalities in these ratios, the patients were compared with the normal subjects (N=73) by using a three-way repeated measures analysis of variance (ANOVA) with region of interest and hemisphere as the repeated measures followed by post hoc comparisons.
Following the MRI scanning session, subjects were rated with the Scale for the Assessment of Negative Symptoms (SANS) (52) and the Psychiatric Symptom Assessment Scale (53) by a psychiatrist (J.H.C.) who was blind to the 1H-MRSI data. Because the ANOVA did not identify a significant interaction of diagnosis by region of interest by side, product moment correlations (r) were computed between mean right and left hemisphere NAA-creatine ratio, NAA-choline ratio, and choline-creatine ratio in each region of interest and summary scores for the total SANS and the positive and negative subscales of the Psychiatric Symptom Assessment Scale. On the basis of our previous hypotheses of selective reduction in the dorsolateral prefrontal cortex (49), all analyses in other regions were corrected by the method of Bonferroni to avoid type I error (corrected p=0.0005 [0.05÷99] [three metabolite ratios × 11 regions of interest × three rating scales]). Within the text, statistical significance for regions outside of the dorsolateral prefrontal cortex will be reported as a corrected p.
Results
The patients with schizophrenia had significant bilateral reductions in NAA/creatine (main effect for diagnosis: F=5.7, df=1, 98, p=0.02; diagnosis by region: F=2.3, df=10, 908, p=0.01; diagnosis by region by hemisphere: F=0.6, df=10, 908, p=0.80). Overall, post hoc comparisons revealed significant reductions only in the dorsolateral prefrontal cortex (F=4.7, df=1, 132, p=0.03) and hippocampal area (F=13.6, df=1, 126, p<0.001), as found previously (54–57). There were no other statistically significant regional differences found. On average, the patients were moderately affected in terms of negative symptoms (SANS mean score=17.6, SD=13.7, and Psychiatric Symptom Assessment Scale negative subscale mean score=4.5, SD=3.1), and overall pathology (Psychiatric Symptom Assessment Scale total mean score=22.2, SD=11.1). These two rating instruments were significantly correlated with each other (r=0.70, N=36, p≤0.001). On the remaining items of the Psychiatric Symptom Assessment Scale (i.e., positive symptoms), the patients were mildly to moderately ill (mean=16.2, SD=9.2).
There was a significant relationship between dorsolateral prefrontal cortex NAA measures and negative symptoms. A negative correlation was found between dorsolateral prefrontal cortex NAA/creatine and SANS total scores (Table 1 and Figure 1) and the negative symptoms subscale of the Psychiatric Symptom Assessment Scale (r=–0.43, N=36, p=0.01). Neither dorsolateral prefrontal cortex NAA/choline nor the dorsolateral prefrontal cortex choline/creatine correlated with negative symptom measures.
Measures of positive symptoms did not correlate with any regional measure of NAA/creatine, NAA/choline, or choline/creatine. In spite of a between-group difference in NAA/creatine in the hippocampal area, there were no significant relationships between hippocampal area NAA/creatine and negative symptoms. However, there were negative correlations between SANS score and NAA/creatine in regions outside of the dorsolateral prefrontal cortex that share dense interconnectivity with the dorsolateral prefrontal cortex, including the thalamus and anterior cingulate (Table 1). Although these regional correlations did not survive statistical correction for multiple comparisons, future studies aimed specifically at these regions should be pursued.
Discussion
We found a regionally selective relationship between evidence of dorsolateral prefrontal cortex neuronal pathology (as reflected in NAA measures) and negative symptoms in patients with schizophrenia. No regional measures predicted positive symptoms in this group of patients. These patients showed significantly lower NAA in only two regions—the dorsolateral prefrontal cortex and hippocampal region—when compared with normal subjects. Although hippocampal NAA measures were not found to predict negative symptoms, we did find evidence of involvement of a larger network in the production of negative symptoms. Specifically, the thalamus and anterior cingulate were implicated by negative correlations between NAA/creatine and negative symptom ratings (Table 1).
Because these regions did not differ significantly between patients and healthy comparison subjects in this study, we cannot rule out the possibility that these represent spurious or secondary correlations. Nonetheless, their relationship to negative symptoms may arise from their dense interconnectivity with the dorsolateral prefrontal cortex. As a post hoc test of this supposition, we performed a forward stepwise multiple regression that included the dorsolateral prefrontal cortex, thalamus, and anterior cingulate NAA/creatine. Partial correlations revealed that neither the thalamus (partial correlation=–0.30, p=0.10) nor the anterior cingulate (partial correlation=–0.21, p=0.20) predicted negative symptoms (according to SANS scores) once the relationship between dorsolateral prefrontal cortex NAA/creatine and SANS scores was factored out. Nonetheless, these data suggest that multiple regions interact to produce negative symptoms.
The shared variance between dorsolateral prefrontal cortex pathology and negative symptoms was not large—approximately 23%. A number of factors may have contributed to this modest predictive value. Both 1H-MRSI and psychiatric rating scales are inherently imprecise measures (49, 52). In addition, 1H-MRSI has a relatively low spatial resolution (about 1.4 cc/voxel with our method). Finally, although NAA is found almost exclusively within neurons (58), the exact functional implications of reduced NAA levels remain unclear. NAA is found in highest concentration in pyramidal neurons in the dorsolateral prefrontal cortex (59). In addition to serving as a storage form of aspartate, NAA is in the metabolic pathway for glutamate and, although not thought to be a neurotransmitter per se (60), is capable of inducing calcium influx by means of N-methyl-d-aspartic acid receptors in vitro (61). Thus, the measurement of NAA concentration in vivo is likely to reflect a number of potentially variable factors.
NAA reductions are clearly linked to a number of neurological disorders involving neuronal pathology (60) and may be reversed by treatment in some instances (62–64). Thus, schizophrenia and its negative symptoms are not likely caused by NAA reductions per se, but, rather, NAA reductions are a marker for some other dorsolateral prefrontal cortex neuronal abnormality. NAA reductions are also not likely the result of a gross loss of neurons in the dorsolateral prefrontal cortex, given the wealth of postmortem human data suggesting that neuronal dropout is not characteristic (65). To the extent that NAA concentrations likely correlate with the overall volume of neuronal soma and processes, the findings of reduced NAA measures are consistent with postmortem evidence of decreased neuropil and soma size (45, 66).
Reduced NAA measures in the dorsolateral prefrontal cortex seem to predict a variety of phenomena associated with manifest illness. In addition to the present findings, dorsolateral prefrontal cortex NAA measures have been found to predict activation within the distributed neural network subserving working memory for patients with schizophrenia (67), to predict baseline and amphetamine-induced striatal dopamine levels (68, 69), and to predict the loss of dorsolateral prefrontal cortex neuronal efficiency during a parametric working memory task (70). This particular predictive power of dorsolateral prefrontal cortex NAA measures is both striking and puzzling. However, given the widespread connectivity of dorsolateral prefrontal cortex neurons throughout the brain, these data may collectively suggest that certain dorsolateral prefrontal cortex neurons represent a population of effector neurons that act within larger cortical networks to determine many of the manifest state variables of schizophrenia.
In our patients, correlations between dorsolateral prefrontal cortex NAA measures and those in the thalamus and anterior cingulate support the supposition of a larger network, but this finding remains to be tested in future data samples. If greater dorsolateral prefrontal cortex neuronal pathology (lower NAA) can be used to predict greater negative symptoms, then these data not only will lend credence to the supposition that negative symptoms arise from dorsolateral prefrontal cortex neuronal pathology but also may provide an additional clinical tool for the assessment of neuropsychiatric patients.
![]() |
Presented in preliminary form at the Fourth International Conference on Functional Mapping of the Human Brain, June 7–12, 1998, Montreal. Received Oct. 12, 1999; revision received April 19, 2000; accepted April 20, 2000. From the Clinical Brain Disorders Branch, Intramural Research Program, NIMH, National Institutes of Health. Address reprint requests to Dr. Callicott, Clinical Brain Disorders Branch, Intramural Research Program, NIMH, National Institutes of Health, 10 Center Dr., Rm. 4D-20, MSC 1389, Bethesda, MD 20892-1389; [email protected] (e-mail).The authors thank Joseph Frank, M.D., Jeff Duyn, Ph.D., and Alan Barnett, Ph.D., for providing expertise, equipment, and software for the analysis of 1H-MRSI data. They also thank the patients and staff of the NIMH inpatient units and the NIMH Clinical Brain Disorders Branch Sibling Study of Schizophrenia. Rebecca Rakow, B.S., Karin Weidenhammer, B.S., and Philip Weissbrod, B.S., provided invaluable assistance in data collection and analysis.
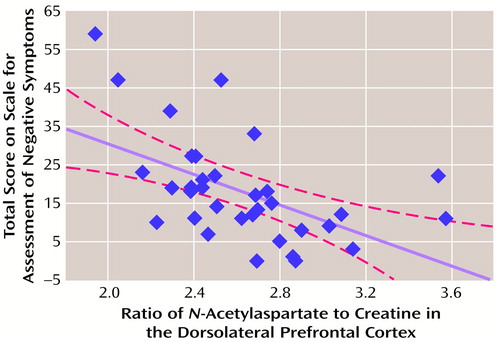
Figure 1. Relationship Between the Dorsolateral Prefrontal Cortex NAA-Creatine Ratio and Negative Symptoms in 36 Patients With Schizophreniaa
aThe ratio is the mean of the right and left hemispheres. The dotted lines indicate 95% confidence intervals (Table 1). Dorsolateral prefrontal cortex NAA was inversely related to negative symptoms (r=–0.68, N=30, p<0.001). Some patients had overlapping values.
1.. Andreasen NC: Improvement of negative symptoms: concepts, definition and assessment. Int Clin Psychopharmacol 1997; 12(suppl 2):S7–S10Google Scholar
2.. McGlashan TH: The profiles of clinical deterioration in schizophrenia. J Schizophr Res 1998; 32:133–141Google Scholar
3.. Sheitman BB, Lieberman JA: The natural history and pathophysiology of treatment resistant schizophrenia. J Schizophr Res 1998; 32:143–150Google Scholar
4.. Gupta S, Rajaprabhakaran R, Arndt S, Flaum M, Andreasen NC: Premorbid adjustment as a predictor of phenomenological and neurobiological indices in schizophrenia. Schizophr Res 1995; 16:189–197Crossref, Medline, Google Scholar
5.. Rosenheck R, Dunn L, Peszke M, Cramer J, Xu W, Thomas J, Charney D (Department of Veterans Affairs Cooperative Study Group on Clozapine in Refractory Schizophrenia): Impact of clozapine on negative symptoms and on the deficit syndrome in refractory schizophrenia. Am J Psychiatry 1999; 156:88–93Link, Google Scholar
6.. Goldberg TE, Weinberger DR: Effects of neuroleptic medications on the cognition of patients with schizophrenia: a review of recent studies. J Clin Psychiatry 1996; 57:62–65Medline, Google Scholar
7.. Piercy M: The effects of cerebral lesions on intellectual function: a review of current research trends. Br J Psychiatry 1964; 110:310–352Crossref, Medline, Google Scholar
8.. Weddell RA, Trevarthen C, Miller JD: Reactions of patients with focal cerebral lesions to success or failure. Neuropsychologia 1988; 26:373–385Crossref, Medline, Google Scholar
9.. Andreasen NC, Nasrallah HA, Dunn V, Olson SC, Grove WM, Ehrhardt JC, Coffman JA, Crossett JH: Structural abnormalities in the frontal system in schizophrenia: a magnetic resonance imaging study. Arch Gen Psychiatry 1986; 43:136–144Crossref, Medline, Google Scholar
10.. Weinberger DR: Implications of normal brain development for the pathogenesis of schizophrenia. Arch Gen Psychiatry 1987; 44:660–669Crossref, Medline, Google Scholar
11.. Goldman-Rakic PS, Selemon LD: Functional and anatomical aspects of prefrontal pathology in schizophrenia. Schizophr Bull 1997; 23:437–458Crossref, Medline, Google Scholar
12.. Andreasen NC, Rezai K, Alliger R, Swayze VW II, Flaum M, Kirchner P, Cohen G, O’Leary DS: Hypofrontality in neuroleptic-naive patients and in patients with chronic schizophrenia: assessment with Xenon 133 single-photon emission computed tomography and the Tower of London. Arch Gen Psychiatry 1992; 49:943–958Crossref, Medline, Google Scholar
13.. Andreasen NC, Ehrhardt JC, Swayze VW II, Alliger RJ, Yuh WT, Cohen G, Ziebell S: Magnetic resonance imaging of the brain in schizophrenia: the pathophysiologic significance of structural abnormalities. Arch Gen Psychiatry 1990; 47:35–44Crossref, Medline, Google Scholar
14.. Chua SE, Wright IC, Poline JB, Liddle PF, Murray RM, Frackowiak RS, Friston KJ, McGuire PK: Grey matter correlates of syndromes in schizophrenia: a semi-automated analysis of structural magnetic resonance images. Br J Psychiatry 1997; 170:406–410Crossref, Medline, Google Scholar
15.. Gur RE, Mozley PD, Shtasel DL, Cannon TD, Gallacher F, Turetsky B, Grossman R, Gur RC: Clinical subtypes of schizophrenia: differences in brain and CSF volume. Am J Psychiatry 1994; 151:343–350Link, Google Scholar
16.. Gur RE, Cowell P, Turetsky BI, Gallacher F, Cannon T, Bilker W, Gur RC: A follow-up magnetic resonance imaging study of schizophrenia: relationship of neuroanatomical changes to clinical and neurobehavioral measures. Arch Gen Psychiatry 1998; 55:145–152Crossref, Medline, Google Scholar
17.. Uematsu M, Kaiya H: Midsagittal cortical pathomorphology of schizophrenia: a magnetic resonance imaging study. Psychiatry Res 1989; 30:11–20Crossref, Medline, Google Scholar
18.. Buchanan RW, Breier A, Kirkpatrick B, Elkashef A, Munson RC, Gellad F, Carpenter WT Jr: Structural abnormalities in deficit and nondeficit schizophrenia. Am J Psychiatry 1993; 150:59–65Link, Google Scholar
19.. Turetsky B, Cowell PE, Gur RC, Grossman RI, Shtasel DL, Gur RE: Frontal and temporal lobe brain volumes in schizophrenia: relationship to symptoms and clinical subtype. Arch Gen Psychiatry 1995; 52:1061–1070Google Scholar
20.. Wright IC, McGuire PK, Poline JB, Travere JM, Murray RM, Frith CD, Frackowiak RS, Friston KJ: A voxel-based method for the statistical analysis of gray and white matter density applied to schizophrenia. Neuroimage 1995; 2:244–252Crossref, Medline, Google Scholar
21.. Cowell PE, Kostianovsky DJ, Gur RC, Turetsky BI, Gur RE: Sex differences in neuroanatomical and clinical correlations in schizophrenia. Am J Psychiatry 1996; 153:799–805Link, Google Scholar
22.. Hoffer A, Osmond H: A card sorting test helpful in establishing prognosis. Am J Psychiatry 1962; 118:840–841Link, Google Scholar
23.. Berman I, Viegner B, Merson A, Allan E, Pappas D, Green AI: Differential relationships between positive and negative symptoms and neuropsychological deficits in schizophrenia. Schizophr Res 1997; 25:1–10Crossref, Medline, Google Scholar
24.. Goldberg TE, Weinberger DR, Berman KF, Pliskin NH, Podd MH: Further evidence for dementia of the prefrontal type in schizophrenia? a controlled study of teaching the Wisconsin Card Sorting Test. Arch Gen Psychiatry 1987; 44:1008–1014Google Scholar
25.. Park S, Holzman PS: Schizophrenics show spatial working memory deficits. Arch Gen Psychiatry 1992; 49:975–982Crossref, Medline, Google Scholar
26.. Fleming K, Goldberg TE, Binks S, Randolph C, Gold JM, Weinberger DR: Visuospatial working memory in patients with schizophrenia. Biol Psychiatry 1997; 41:43–49Crossref, Medline, Google Scholar
27.. Goldman-Rakic PS: Working memory dysfunction in schizophrenia. J Neuropsychiatry Clin Neurosci 1994; 6:348–357Crossref, Medline, Google Scholar
28.. Wexler BE, Stevens AA, Bowers AA, Sernyak MJ, Goldman-Rakic PS: Word and tone working memory deficits in schizophrenia. Arch Gen Psychiatry 1998; 55:1093–1096Google Scholar
29.. Carter C, Robertson L, Nordhal T, Chaderjian M, Kraft L, O’Shora-Celaya L: Spatial working memory deficits and their relationship to negative symptoms in unmedicated schizophrenia patients. Biol Psychiatry 1996; 40:930–932Crossref, Medline, Google Scholar
30.. Keefe RS, Roitman SE, Harvey PD, Blum CS, DuPre RL, Prieto DM, Davidson M, Davis KL: A pen-and-paper human analogue of a monkey prefrontal cortex activation task: spatial working memory in patients with schizophrenia. Schizophr Res 1995; 17:25–33Crossref, Medline, Google Scholar
31.. Kawasaki Y, Suzuki M, Maeda Y, Urata K, Yamaguchi N, Matsuda H, Hisada K, Suzuki M, Takashima T: Regional cerebral blood flow in patients with schizophrenia: a preliminary report. Eur Arch Psychiatry Clin Neurosci 1992; 241:195–200Crossref, Medline, Google Scholar
32.. Liddle PF, Friston KJ, Frith CD, Hirsch SR, Jones T, Frackowiak RS: Patterns of cerebral blood flow in schizophrenia. Br J Psychiatry 1992; 160:179–186Crossref, Medline, Google Scholar
33.. Yuasa S, Kurachi M, Suzuki M, Kadono Y, Matsui M, Saitoh O, Seto H: Clinical symptoms and regional cerebral blood flow in schizophrenia. Eur Arch Psychiatry Clin Neurosci 1995; 246:7–12Crossref, Medline, Google Scholar
34.. Molina Rodriguez V, Montz Andree R, Perez Castejon MJ, Gutierrez Labrador R, Ferre Navarrete F, Carreas Delgado JL, Rubia Vila FJ: Cerebral perfusion correlates of negative symptomatology and parkinsonism in a sample of treatment-refractory schizophrenics: an exploratory 99mTc-HMPAO SPET study. Schizophr Res 1997; 25:11–20Crossref, Medline, Google Scholar
35.. Sabri O, Erkwoh R, Schreckenberger M, Cremerius U, Schulz G, Dickmann C, Kaiser HJ, Steinmeyer EM, Sass H, Buell U: Regional cerebral blood flow and negative/positive symptoms in 24 drug-naive schizophrenics. J Nucl Med 1997; 38:181–188Medline, Google Scholar
36.. Holzman PS, Proctor LR, Hughes DW: Eye-tracking patterns in schizophrenia. Science 1973; 181:179–181Crossref, Medline, Google Scholar
37.. Abrams R, Taylor MA: Differential EEG patterns in affective disorder and schizophrenia. Arch Gen Psychiatry 1979; 36:1355–1358Google Scholar
38.. Tauscher J, Fischer P, Neumeister A, Rappelsberger P, Kasper S: Low frontal electroencephalographic coherence in neuroleptic-free schizophrenic patients. Biol Psychiatry 1998; 44:438–447Crossref, Medline, Google Scholar
39.. Weinberger DR, Berman KF: Prefrontal function in schizophrenia: confounds and controversies. Phil Trans R Soc Med 1996; 351:1495–1503Google Scholar
40.. Yurgelun-Todd DA, Waternaux CM, Cohen BM, Gruber SA, English CD, Renshaw PF: Functional magnetic resonance imaging of schizophrenic patients and comparison subjects during word production. Am J Psychiatry 1996; 153:200–205Link, Google Scholar
41.. Volz HP, Gaser C, Hager F, Rzanny R, Mentzel HJ, Kreitschmann-Andermahr I, Kaiser WA, Sauer H: Brain activation during cognitive stimulation with the Wisconsin Card Sorting Test—a functional MRI study on healthy volunteers and schizophrenics. Psychiatry Res 1997; 75:145–157Crossref, Medline, Google Scholar
42.. Callicott JH, Ramsey NF, Tallent K, Bertolino A, Knable MB, Coppola R, Goldberg T, van Gelderen P, Mattay VS, Frank JA, Moonen CT, Weinberger DR: Functional magnetic resonance imaging brain mapping in psychiatry: methodological issues illustrated in a study of working memory in schizophrenia. Neuropsychopharmacology 1998; 18:186–196Crossref, Medline, Google Scholar
43.. Carter CS, Perlstein W, Ganguli R, Brar J, Mintun M, Cohen JD: Functional hypofrontality and working memory dysfunction in schizophrenia. Am J Psychiatry 1998; 155:1285–1287Google Scholar
44.. Stevens AA, Goldman-Rakic PS, Gore JC, Fulbright RK, Wexler BE: Cortical dysfunction in schizophrenia during auditory word and tone working memory demonstrated by functional magnetic resonance imaging. Arch Gen Psychiatry 1998; 55:1097–1103Google Scholar
45.. Selemon LD, Goldman-Rakic PS: The reduced neuropil hypothesis: a circuit based model of schizophrenia. Biol Psychiatry 1999; 45:17–25Crossref, Medline, Google Scholar
46.. Woo TU, Whitehead RE, Melchitzky DS, Lewis DA: A subclass of prefrontal gamma-aminobutyric acid axon terminals are selectively altered in schizophrenia. Proc Natl Acad Sci USA 1998; 95:5341–5346Google Scholar
47.. Benes FM, McSparren J, Bird ED, SanGiovanni JP, Vincent SL: Deficits in small interneurons in prefrontal and cingulate cortices of schizophrenic and schizoaffective patients. Arch Gen Psychiatry 1991; 48:996–1001Google Scholar
48.. Akbarian S, Bunney WE, Potkin SG, Wigal SB, Hagman JO, Sandman CA, Jones GE: Altered distribution of nicotinamide-adenine dinucleotide phosphate diaphorase cells in frontal lobe of schizophrenics implies disturbances of cortical development. Arch Gen Psychiatry 1993; 50:169–177Crossref, Medline, Google Scholar
49.. Bertolino A, Weinberger DR: Proton magnetic resonance spectroscopy in schizophrenia. Eur J Radiol 1999; 2:132–141Crossref, Google Scholar
50.. First MB, Spitzer RL, Gibbon M, Williams JBW: Structured Clinical Interview for DSM-IV Axis I Disorders, Patient Edition (SCID-P), version 2. New York, New York State Psychiatric Institute, Biometrics Research, 1995Google Scholar
51.. Duyn J, Gillen J, Sobering G, Van ZP, Moonen C: Multisection proton MR spectroscopic imaging of the brain. Radiology 1993; 188:277–282Crossref, Medline, Google Scholar
52.. Andreasen NC: The Scale for the Assessment of Negative Symptoms (SANS): conceptual and theoretical foundations. Br J Psychiatry Suppl 1989; 7:49–58Medline, Google Scholar
53.. Bigelow LB, Berthot BD: The Psychiatric Symptom Assessment Scale. Psychopharmacol Bull 1989; 25:168–173Medline, Google Scholar
54.. Bertolino A, Nawroz S, Mattay VS, Barnett AS, Duyn JH, Moonen CTW, Frank JA, Tedeschi G, Weinberger DR: Regionally specific pattern of neurochemical pathology in schizophrenia as assessed by multislice proton magnetic resonance spectroscopic imaging. Am J Psychiatry 1996; 153:1554–1563Google Scholar
55.. Bertolino A, Callicott JH, Elman I, Mattay VS, Tedeschi G, Frank JA, Breier A, Weinberger DR: Regionally specific neuronal pathology in untreated patients with schizophrenia: a proton magnetic resonance spectroscopic imaging study. Biol Psychiatry 1998; 43:641–648Crossref, Medline, Google Scholar
56.. Bertolino A, Kumra S, Callicott JH, Mattay VS, Lestz RM, Jacobsen L, Barnett IS, Duyn JH, Frank JA, Rapoport JL, Weinberger DR: Common pattern of cortical pathology in childhood-onset and adult-onset schizophrenia as identified by proton magnetic resonance spectroscopic imaging. Am J Psychiatry 1998; 155:1376–1383Google Scholar
57.. Bertolino A, Callicott JH, Nawroz S, Mattay VS, Duyn JH, Tedeschi G, Frank JA, Weinberger DR: Reproducibility of proton magnetic resonance spectroscopic imaging in patients with schizophrenia. Neuropsychopharmacology 1998; 18:1–9Crossref, Medline, Google Scholar
58.. Urenjak J, Williams S, Gadian D, Noble M: Proton nuclear magnetic resonance spectroscopy unambiguously identifies different neural cell types. J Neurosci 1993; 13:981–989Crossref, Medline, Google Scholar
59.. Moffett JR, Namboodiri MA: Differential distribution of N-acetylaspartylglutamate and N-acetylaspartate immunoreactivities in rat forebrain. J Neurocytol 1995; 24:409–433Crossref, Medline, Google Scholar
60.. Tsai C, Coyle JT: N-Acetylaspartate in neuropsychiatric disorders. Prog Neurobiol 1995; 46:531–540Crossref, Medline, Google Scholar
61.. Rubin Y, LaPlaca MC, Smith DH, Thibault LE, Lenkinski RE: The effect of N-acetylaspartate on the intracellular calcium concentration in NTera2-neurons. Neurosci Lett 1995; 198:209–212Crossref, Medline, Google Scholar
62.. Cendes F, Andermann F, Dubeau F, Matthews PM, Arnold DL: Normalization of neuronal metabolic dysfunction after surgery for temporal lobe epilepsy: evidence from proton MR spectroscopic imaging. Neurology 1997; 49:1525–1533Google Scholar
63.. De Stefano N, Matthews P, Antel JP, Preul M, Francis G, Arnold DL: Chemical pathology of acute demyelinating lesions and its correlations with disability. Ann Neurol 1995; 38:901–909Crossref, Medline, Google Scholar
64.. Vion-Dury J, Salvan AM, Confort-Gouny S, Dhiver C, Cozzone P: Reversal of brain metabolic alterations with zidovudine detected by proton localised magnetic resonance spectroscopy. Lancet 1995; 345:60–61Crossref, Medline, Google Scholar
65.. Selemon LD, Lidow MS, Goldman-Rakic PS: Increased volume and glial density in primate prefrontal cortex associated with chronic antipsychotic drug exposure. Biol Psychiatry 1999; 46:161–172Crossref, Medline, Google Scholar
66.. Rajkowska G, Selemon LD, Goldman-Rakic PS: Neuronal and glial somal size in the prefrontal cortex: a postmortem morphometric study of schizophrenia and Huntington disease. Arch Gen Psychiatry 1998; 55:215–224Crossref, Medline, Google Scholar
67.. Bertolino A, Esposito G, Callicott JH, Mattay VS, Van Horn JD, Frank JA, Berman KF, Weinberger DR: Specific relationship between prefrontal neuronal N-acetylaspartate and activation of the working memory cortical network in schizophrenia. Am J Psychiatry 2000; 157:26–33Link, Google Scholar
68.. Bertolino A, Knable MB, Saunders RC, Callicott JH, Kolachana B, Mattay VS, Bachevalier J, Frank JA, Egan M, Weinberger DR: The relationship between dorsolateral prefrontal neuronal N-acetylaspartate and evoked release of striatal dopamine in schizophrenia. Biol Psychiatry 1999; 45:660–667Crossref, Medline, Google Scholar
69.. Bertolino A, Breier A, Callicott JH, Adler C, Mattay VS, Shapiro M, Frank JA, Pickar D, Weinberger DR: The relationship between dorsolateral prefrontal neuronal N-acetylaspartate and evoked release of striatal dopamine in schizophrenia. Neuropsychopharmacology 2000; 22:125–132Crossref, Medline, Google Scholar
70.. Callicott JH, Bertolino A, Mattay VS, Langheim FJP, Duyn J, Coppola R, Goldberg TE, Weinberger DR: Physiological dysfunction of the dorsolateral prefrontal cortex in schizophrenia revisited. Cereb Cortex (in press)Google Scholar