Association Between Age-Related Decline in Brain Dopamine Activity and Impairment in Frontal and Cingulate Metabolism
Abstract
OBJECTIVE: Despite the well-documented loss of brain dopamine activity with age, little is known about its functional consequences in healthy individuals. This study investigates the relationship between measures of brain dopamine D2 receptors (molecules that transmit dopamine signals) and regional brain glucose metabolism (a marker of brain function) in healthy individuals. METHOD: Thirty-seven healthy volunteers aged 24–86 years underwent positron emission tomography scans after injection of [11C]raclopride to assess dopamine D2 receptors and [18]fluorodeoxyglucose to assess regional brain glucose metabolism. Two methods used to assess the correlations between metabolism and dopamine D2 receptors—pixel-by-pixel correlations and correlations in preselected regions of interest—were then compared. RESULTS: D2 receptors as well as frontal and cingulate metabolism declined with age. Regardless of the method used, significant correlations between metabolism and D2 receptors were found in the frontal cortex (Brodmann’s areas 6, 7, 8, 9, 10, 11, 44, 45, 47), anterior cingulate gyrus (areas 24, 32), temporal cortex (area 21), and caudate. These correlations remained significant after removing age effects (partial correlation). CONCLUSIONS: These results provide the first link between age-related declines in brain dopamine activity and frontal and cingulate metabolism, which supports the need to investigate the therapeutic utility of interventions that enhance dopamine function in the elderly. The fact that correlations remained significant after removing age effects suggests that dopamine may influence frontal, cingulate, and temporal metabolism regardless of age.
The continuing increase in human life expectancy places a sense of urgency on understanding the causes of disability in the elderly so that therapeutic interventions can be developed to improve their health and quality of life (1). Among the major causes of disability in the elderly are those pertaining to changes in brain function (2). The brain dopamine system, which plays a pivotal role in motor activity, motivation, and reward, is particularly vulnerable to age (3). In the human brain, significant losses over a normal lifespan have been reported for dopamine D2 receptors (4), dopamine D1 receptors (5), and dopamine transporters (6). Studies of dopamine metabolism have been less consistent, showing decreases as well as no changes with aging (7). However, very little is known about the functional consequences of the age-related dopamine decline. Part of the difficulty is that most of the studies in humans have been done postmortem, and thus there is limited information on the functional state of the brain of these subjects.
With imaging techniques such as positron emission tomography (PET), it is now possible to measure dopamine-specific parameters and parameters that reflect brain function (e.g., regional brain glucose metabolism) in living human subjects (8). For instance, through PET we recently documented that the age-related decline in dopamine D2 receptors was associated not only with impaired motor performance but also with impaired performance in neurocognitive tasks that involve the frontal cortex (9). We interpreted these findings as suggesting that the neurocognitive deficits were due to a dysregulation of the frontal regions from the loss in dopamine brain activity. Although multiple studies have revealed a significant decline in frontal and cingulate metabolism with age (10), no study has evaluated directly whether the changes in dopamine brain activity are, in fact, associated with the changes in frontal and cingulate metabolism.
Dopamine could modulate frontal activity indirectly through the striatal-pallidal-thalamic circuit (11) or directly through projections from mesencephalic dopamine cells (12). The importance of dopamine in frontal activity has been suggested by imaging studies, which have shown that dopamine-enhancing agents increase frontal activation in response to task stimulation (13, 14).
The purpose of this study was to map the effects of dopamine receptor availability on regional cerebral metabolism and investigate the association between age-related decrements in D2 receptors and regional brain glucose metabolism.
METHOD
Participants
Thirty-seven healthy volunteers (32 men, five women) were selected for the study. The mean age of the subjects was 49.0 years (SD=18.0, range=24–86). Subjects were recruited by advertisement in local newspapers and through word of mouth among employees and students. Participants had an average of 15 years (SD=3) of education, and their mean verbal IQ was 116 (SD=16). Participants were excluded if they had a past or present history of cardiovascular, psychiatric, neurological, metabolic, or endocrinological disease or history of dependence on any substance other than nicotine or caffeine. As part of the evaluation, all participants received a complete medical, neurological, psychiatric, and neurocognitive evaluation to ensure that they had no medical or neurocognitive impairment. No participants were taking medications at the time of the study, and pre-scan urine tests were done to ensure the absence of psychoactive drug use. After complete description of the study to the participants, written informed consent was obtained.
PET Scans
PET scans were done with a CTI 931 tomograph (6.5×5.9×5.9 mm full width at half maximum, 15 slices). One 20-minute emission scan was started 35 minutes after intravenous injection of 4–6 mCi of [18F]fluorodeoxyglucose (FDG). Sequential emission scans were obtained immediately after intravenous injection of 4–10 mCi of [11C]raclopride (specific activity 0.5–1.5 Ci/µmol at the time of injection) for a total of 60 minutes as previously described (16). Details on synthesis of FDG and [11C]raclopride, subject positioning, transmission and emission scans, blood sampling procedures, [11C]raclopride quantification and metabolite analyses, and procedures for calculation of metabolic rates have been described (16, 17). Scans were carried out under resting conditions (supine, eyes open, ears unplugged), noise was kept to a minimum, and the room was dimly illuminated.
Image and Data Analysis
To assess the estimates of D2 receptor availability from the [11C]raclopride images, we obtained regions in the striatum and in the cerebellum as previously described (16). The time-activity curves for [11C]raclopride in the striatum and cerebellum and the time-activity curves for unchanged tracer in plasma were used to obtain distribution volumes (18). Our measure of D2 receptor availability was the ratio of the distribution volume in the striatum to that in the cerebellum, which corresponds to (Bmax/Kd)+1 and is insensitive to changes in cerebral blood flow (19).
The correlation analysis for striatal dopamine D2 receptors (Bmax/Kd) was carried out on the metabolic images on a pixel-by-pixel basis. For this purpose the subjects’ metabolic images were normalized to their maximal value (average metabolic activity for all pixels within 5% of the highest value) and were registered to the Talairach and Tournoux atlas (20) by using the software package for Statistical Parametric Mapping SPM95 (21). The original 15 slices from the CTI PET camera were interpolated into 31 slices that corresponded to the atlas. Because of some differences in positioning, not all participants contributed to every slice. A cutoff of 20% of the global maximum value of metabolic rate was used for each subject to determine which pixels were to be included in the correlation analysis. Vectors of Bmax/Kd and the corresponding subject metabolic rate for a given pixel were used in the analysis if at least 35 of the participants contributed to the metabolic rate vector. Pearson’s product-moment correlation was calculated for each contributing pixel that satisfied the above conditions. Images of the correlation coefficient and of the corresponding p value were created using the C program “pearsn” and related programs from Numerical Recipes in C (22). Programs written in PVWave were used to select out pixels that satisfied specified correlational criteria (r>0.52, df=36, p<0.001). The pixels with significant correlations were projected onto the Talairach and Tournoux atlas (19) for identification.
To corroborate the results from the pixel-by-pixel analysis, we independently assessed the pattern of correlation in metabolic measures obtained in preselected regions of interest. For this purpose we used a template to initially locate 114 regions of interest in the metabolic images, which were then individually fitted to each subject’s images as previously described (17). Weighted averages of the regions of interest from different slices that corresponded to the same anatomical areas were computed to obtain metabolic values in 24 “composite” brain regions. A measure of “whole brain” metabolism was computed by averaging metabolism in the 15 brain slices and was used to normalize the regional measures (regions of interest/whole brain). Pearson product-moment correlation analyses were performed between the normalized regional measures and Bmax/Kd. Because a positive correlation between regional metabolism and D2 receptors (Bmax/Kd) could reflect the effects of age on these two variables, it was important to measure the correlation with the effects of age removed. For this purpose we measured the partial correlations (partialing out for age effects) on the correlations that were significant. Pearson product-moment correlation analyses were also performed between the normalized metabolic measures and age. To correct for multiple comparisons in the regions of interest analysis, we set the level of significance at p<0.01.
RESULTS
Global absolute metabolism ranged between 26 and 41 µmol/100g per minute and was not correlated with age or with D2 receptor availability. However, the regional measures for metabolism declined significantly with age in several frontal brain regions and in the anterior cingulate as well as in the right supramarginal gyrus (table 1). Age was also associated with a significant decline in striatal dopamine D2 receptors (r=0.55, df=36, p<0.0001). We consequently used partial correlations to remove the effects of age from all subsequent analyses, which were found to be robust to this procedure. Therefore, the raw, uncorrected results will be reported.
The correlations between regional glucose metabolism and dopamine D2 receptor availability were significant mostly for cortical brain regions. figure 1 shows (superimposed on the Talairach-Tournoux atlas) the pixels with significant (r<0.52, df=36, p<0.001) correlations between D2 receptors and metabolism. Most of the significant correlations were located in the anterior cingulate gyrus and the frontal cortex, including areas in the prefrontal, motor, and orbitofrontal cortex (table 2). Significant correlations were also located in association areas in the temporal cortex and in the caudate.
This pattern of correlations was independently corroborated when the analysis was done by using the relative metabolic measures obtained in preselected regions of interest (table 1, figure 2). Because the variance from relative measures can result either from changes in the region or in the global measure, we also corroborated whether the significant correlations were also observed for the absolute measures. The correlations with the absolute measures were significant for the cingulate gyrus (r=0.47, df=36, p<0.005) and left caudate (r=0.53, df=36, p<0.001) and showed a trend for significance in the frontal and superior temporal gyrus (r>0.33, df=36, 0.01<p>0.05).
DISCUSSION
In this group of healthy participants, glucose metabolism was found to decrease with age in frontal brain regions and anterior cingulate gyrus. Reductions in frontal and anterior cingulate metabolism with age have been consistently reported by imaging studies of the aging human brain (10). The present study indicates that these age-linked metabolic reductions were coupled with the decline in dopamine D2 receptors. We documented a similar association in a group of cocaine abusers in whom the reductions in D2 receptors were associated with metabolic decrements in the prefrontal cortex and in the anterior cingulate gyrus (23). However, this is the first demonstration in healthy adults of a direct association between the levels of D2 receptors in brain and cerebral metabolic activity.
It is likely that dopamine dysfunction underlies motor impairment in the elderly (dyskinesias and rigidity), but the present results suggest that it may also underlie some of the cognitive changes seen in older individuals. Note that the cognitive deficits associated with age involve frontal lobe function (24). Moreover, in healthy elderly people the decline in D2 receptors is associated with disrupted performance in tasks related to frontal lobe function, such as executive function (Wisconsin Card Sorting Test) and response inhibition (Stroop Interference) (9). The significant association between D2 receptors and metabolism in prefrontal and anterior cingulate cortices provides an explanation of how decline in dopamine activity would result in impairment in these tasks, since these brain regions are involved in their execution (25, 26)). Although there was a significant association between striatal D2 receptors and metabolism in caudate, the correlation in putamen was not significant. The reason for this differential correlation is unclear.
It is noteworthy that some brain regions showing correlations between metabolism and D2 receptors did not decline with age (i.e., caudate), while metabolism that declined with age in some areas was not correlated with D2 receptors (supramarginal gyrus). This most likely reflects age-related changes in multiple neurotransmitter systems, which, in turn, affect the activity of brain regions they modulate. Moreover, the function of a given brain region is likely to be regulated by more than one neurotransmitter. Thus, it is unlikely that complete correspondence would be found between degeneration of the dopamine system and the function of a given brain region.
For this study the relationship between D2 receptors and metabolism was obtained during baseline conditions (no active stimulation). This would imply that dopamine’s modulation of projection regions under nonstimulation conditions, and hence presumably for tonic dopamine release (27), could be, in part, dependent on the availability of D2 receptors. In fact, increases in dopamine concentration induced by methylphenidate, a drug that blocks dopamine reuptake into the terminal, changed regional brain metabolism in proportion to the availability of D2 receptors (28). Although this study supports the role of dopamine in regulating frontal activity through its interaction with D2 receptors, a different pattern of interactions may emerge if the analysis is done for other dopamine receptors such as D1.
A limitation for this study was that the metabolic measures were not corrected for brain atrophy. However, it is unlikely that the correlations were driven by atrophy, since atrophy accounts for only a small percent of the variance in the regional metabolic measures seen with age (29). Another limitation is the concern of chance findings when performing multiple correlations. However, the fact that the two methods of analysis used to corroborate the findings (pixel by pixel and preselected regions of interest) gave an almost identical pattern of correlations suggests that the findings are not due to chance. Even though the correlation between frontal metabolism and D2 receptors remained significant after removing age effects, future studies in which this relationship is assessed in elderly subjects would serve to solidify this finding. Finally, although regional brain glucose metabolism, as assessed with PET, serves as a good marker of brain function, it does not allow one to determine the cellular mechanisms underlying the changes in metabolic activity. Studies in laboratory animals are required to determine which molecular targets are responsible for the association between the decline in dopamine receptors and the reduction in frontal metabolism.
This study provides direct evidence in humans that age-related losses in D2 receptors are functionally significant and predominantly affect the frontal and cingulate brain regions. Understanding the role that the degeneration of the dopamine system has on the function of the human brain in the elderly is a crucial step in the development of interventions targeted toward ameliorating these changes and retarding their presentation.
Received March 26, 1999; revision received July 29, 1999; accepted Aug. 2, 1999. From the Medical and Chemistry Departments, Brookhaven National Laboratory; the Department of Psychiatry, State University of New York at Stony Brook; and the Brain-Behavior Laboratory, Department of Psychiatry, University of Pennsylvania School of Medicine, Philadelphia. Address reprint requests to Dr. Volkow, Brookhaven National Laboratory, Upton, NY 11973; [email protected] (e-mail). Supported in part by the U.S. Department of Energy under contract DE-ACO2-76CH00016 and grant DA-06891 from the National Institute on Drug Abuse. The authors thank David Schlyer for cyclotron operations; Alex Levy and Donald Warner for PET operations; Colleen Shea, Richard Ferrieri, Robert MacGregor, and Payton King for radiotracer preparation; Noelwah Netusil for care of subjects; and Carol Redvanly for organization.
![]() |
![]() |
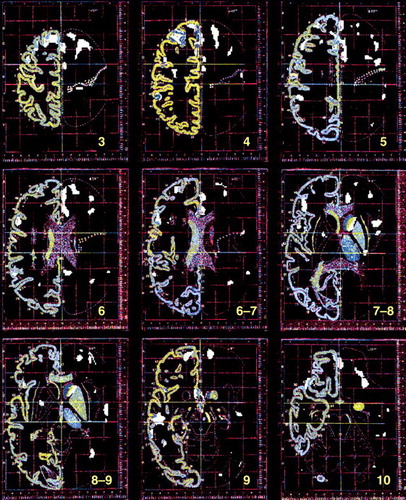
FIGURE 1. Brain Images Depicting Pixel-by-Pixel Correlations Between Regional Glucose Metabolism and Striatal Dopamine D2 Receptors for 37 Healthy Subjects 24–86 Years Olda
aThe images, obtained from the Talairach-Tournoux Atlas (19), correspond to planes located +50 mm, +40 mm, +32 mm, +24 mm, +16 mm, +8 mm, –1 mm, –8 mm, and –16 mm from the intercommissural line. Pixels with significant correlations (r>0.52, df=36, p<0.001) are marked in white. Significant correlations were located in the cingulate gyrus, frontal cortex (prefrontal, motor, and orbitofrontal cortex), temporal cortex (Brodmann’s area 21 and temporal insula), parietal cortex (Brodmann’s area 40), and caudate.
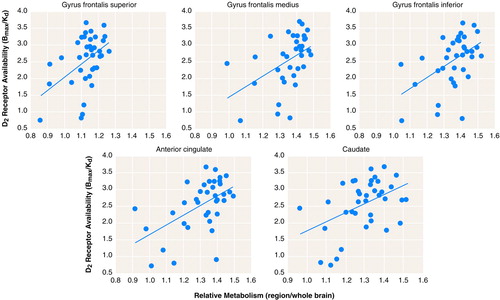
FIGURE 2. Regression Slopes Depicting Correlations Between Striatal Dopamine D2 Receptors (B max/K d) and Relative Glucose Metabolism in Preselected Brain Regions in 37 Healthy Subjects 24–86 Years Old
1. Schneider EL: Aging in the third millennium. Science 1999; 283:796–797Crossref, Medline, Google Scholar
2. Phillips P: European neurologists focus on aging. JAMA 1998; 280:1973–1974Google Scholar
3. Joseph JA, Roth GS, Strong R: The striatum, a microcosm for the examination of age-related alterations in the CNS: a selected review. Rev Biol Res Aging 1990; 4:181–199Google Scholar
4. Volkow ND, Wang G-J, Fowler JS, Logan J, Gatley SJ, MacGregor RR, Schlyer D, Hitzemann R, Wolf AP: Measuring age-related changes in DA D2 receptors with [11C]raclopride and with [18F]N-methylspiroperidol. Psychiatry Res 1996; 67:11–16Crossref, Medline, Google Scholar
5. Suhara T, Fukuda H, Inoue O, Itoh T, Suzuki K, Yamasaki T, Tateno Y: Age-related changes in human D1 dopamine receptors measured by positron emission tomography. Psychopharmacology (Berl) 1991; 103:41–45Crossref, Medline, Google Scholar
6. Volkow ND, Ding Y-S, Fowler JS, Wang G-J, Logan J, Gatley SJ, Hitzemann R, Smith G, Fields F, Gur R, Wolf AP: Dopamine transporters decrease with age in healthy subjects. J Nucl Med 1996; 37:554–558Medline, Google Scholar
7. Eidelberg D, Takikawa S, Dhawan V, Chaly T, Robeson W, Dahl R, Margouleff D, Moeller JR, Patlak CS, Fahn S: Striatal 18F-DOPA uptake: absence of an aging effect. J Cereb Blood Flow Metab 1993; 13:881–888Crossref, Medline, Google Scholar
8. Volkow ND, Fowler JS, Gatley JS, Logan J, Wang G-J, Ding YS, Dewey SL: PET evaluation of the dopamine system of the human brain. J Nucl Med 1996; 37:1242–1256Google Scholar
9. Volkow ND, Gur RC, Wang G-J, Fowler JS, Moberg PJ, Ding Y-S, Hitzemann R, Smith G, Logan J: Association between decline in brain dopamine activity with age and cognitive and motor impairment in healthy individuals. Am J Psychiatry 1998; 155:344–349Link, Google Scholar
10. Moeller JR, Ishikawa T, Dhawan V, Spetsieris P, Mandel F, Alexander GE, Grady C, Pietrini P, Eidelberg D: The metabolic topography of normal aging. J Cereb Blood Flow Metab 1996; 16:385–398Crossref, Medline, Google Scholar
11. Heimer L, Alheid GF, Zaborzky L: The basal ganglia, in The Rat Nervous System. Edited by Paxinos G. New York, Academic Press, 1985, pp 37–74Google Scholar
12. Williams SM, Goldman-Rakic PS: Widespread origin of the primate mesofrontal dopamine system. Cereb Cortex 1998; 8:321–345Crossref, Medline, Google Scholar
13. Daniel DG, Weinberger DR, Jones DW, Zigun JR, Coppola R, Handel S, Bigelow LB, Goldberg TE, Berman KF, Kleinman JE: The effect of amphetamine on regional cerebral blood flow during cognitive activation in schizophrenia. J Neurosci 1991; 11:1907–1917Google Scholar
14. Dolan RJ, Fletcher P, Frith CD, Friston KJ, Frackowiak RSJ, Grasby PM: Dopaminergic modulation of impaired cognitiveactivation in the anterior cingulate cortex in schizophrenia. Nature 1995; 378:180–182Crossref, Medline, Google Scholar
16. Volkow ND, Fowler JS, Wang G-J, Dewey SL, Schlyer D, MacGregor R, Logan J, Alexoff D, Shea C, Hitzemann R, Angrist B, Wolf AP: Reproducibility of repeated measures of carbon-11-raclopride binding in the human brain. J Nucl Med 1993; 34:609–613Medline, Google Scholar
17. Wang G-J, Volkow ND, Roque CT, Cestaro VL, Hitzemann RJ, Cantos EL, Levy AV, Dhawan AP: Functional importance of ventricular enlargement and cortical atrophy in healthy subjects and alcoholics as assessed with PET, MR imaging, and neuropsychologic testing. Radiology 1992; 186:59–65Crossref, Google Scholar
18. Logan J, Fowler JS, Volkow ND, Wolf AP, Dewey SL, Schlyer D, MacGregor RR, Hitzemann R, Bendriem B, Gatley SJ, Christman DR: Graphical analysis of reversible radioligand binding from time-activity measurements applied to [N-11C-methyl]-(-)-cocaine PET studies in human subjects. J Cereb Blood Flow Metab 1990; 10:740–747Crossref, Medline, Google Scholar
19. Logan J, Volkow ND, Fowler JS, Wang G-J, Dewey SL, MacGregor R, Schlyer D, Gatley SJ, Pappas N, King P, Hitzemman R, Vitkun S: Effects of blood flow on [11C]raclopride binding in the brain: model simulations and kinetic analysis of PET data. J Cereb Blood Flow Metab 1994; 14:995–1010Google Scholar
20. Talairach J, Tournoux P: Co-Planar Stereotaxic Atlas of the Human Brain. New York, Thieme Medical, 1988Google Scholar
21. SPM95 Software. London, Hammersmith Hospital, MRC Cyclotron Unit, 1995Google Scholar
22. Flannery BP, Teukplsky SA, Vetterling WT: Numerical Recipes In C: The Art of Scientific Computing. Cambridge, UK, Cambridge University Press, 1988Google Scholar
23. Volkow ND, Fowler JS, Wang G-J, Hitzemann R, Logan J, Schlyer D, Dewey S, Wolf AP: Decreased dopamine D2 receptor availability is associated with reduced frontal metabolism in cocaine abusers. Synapse 1993; 14:169–177Crossref, Medline, Google Scholar
24. West RL: An application of prefrontal cortex function theory to cognitive aging. Psychol Bull 1996; 120:272–292Crossref, Medline, Google Scholar
25. Weinberger DR, Berman KF, Zec RF: Physiologic dysfunction of dorsolateral prefrontal cortex in schizophrenia, I: regional cerebral blood flow evidence. Arch Gen Psychiatry 1986; 43:114–124Crossref, Medline, Google Scholar
26. Pardo JV, Pardo PJ, Janer KW, Raichle ME: The anterior cingulate cortex mediates processing selection in the Stroop Attentional Conflict Paradigm. Proc Natl Acad Sci USA 1990; 87:256–259Crossref, Medline, Google Scholar
27. Grace AA: Phasic versus tonic dopamine release and the modulation of dopamine system responsivity: a hypothesis for the etiology of schizophrenia. Neuroscience 1991; 41:1–24Crossref, Medline, Google Scholar
28. Volkow ND, Wang G-J, Fowler JS, Logan J, Angrist B, Hitzemann R, Lieberman J, Pappas N: Effects of methylphenidate on regional brain glucose metabolism in humans: relationship to dopamine D2 receptors. Am J Psychiatry 1997; 154:50–55Link, Google Scholar
29. Schlageter NL, Horwitz B, Creasey H, Carson R, Duara R, Berg GW, Rapoport SI: Relation of measured brain glucose utilisation and cerebral atrophy in man. J Neurol Neurosurg Psychiatry 1987; 50:779–785Crossref, Medline, Google Scholar