Dopamine Transporter Occupancies in the Human Brain Induced by Therapeutic Doses of Oral Methylphenidate
Abstract
Objective:The therapeutic effects of methylphenidate in the treatment of attention deficit disorder have been attributed to its ability to increase the synaptic concentration of dopamine by blocking the dopamine transporters. However, the levels of dopamine transporter blockade achieved by therapeutic doses of methylphenidate are not known. This study measured, for the first time, dopamine transporter occupancy by orally administered methylphenidate in the human brain and its rate of uptake in the brain. Method:Positron emission tomography (PET) and [11C]cocaine were used to estimate dopamine transporter occupancies after different doses of oral methylphenidate in seven normal subjects (mean age=24 years, SD=7). In addition, the pharmacokinetics of oral methylphenidate were measured in the baboon brain through use of PET and [11C]methylphenidate administered through an orogastric tube.Results:At 120 minutes after administration, oral methylphenidate produced a dose-dependent blockade of dopamine transporter; means=12% (SD=4%) for 5 mg, 40% (SD=12%) for 10 mg, 54% (SD=5%) for 20 mg, 72% (SD=3%) for 40 mg, and 74% (SD=2%) for 60 mg. The estimated dose of oral methylphenidate required to block 50% of the dopamine transporter corresponded to 0.25 mg/kg. Oral methylphenidate did not reach peak concentration in brain until 60 minutes after its administration.Conclusions:Oral methylphenidate is very effective in blocking dopamine transporters, and at the weight-adjusted doses used therapeutically (0.3 to 0.6 mg/kg), it is likely to occupy more than 50% of the dopamine transporters. The time to reach peak brain uptake for oral methylphenidate in brain corresponds well with the reported time course to reach peak behavioral effects. Am J Psychiatry 1998; 155: 1325-1331
Attention deficit hyperactivity disorder (ADHD) is the most commonly diagnosed behavioral disorder of childhood (1). The prevalence of the disorder has been recently estimated to be 5%–10% of the general population (1). The increase in diagnosis of ADHD seen over the past decade appears to reflect an increase in recognition of the disorder and has led to a dramatic increase in the prescription of methylphenidate (2), the drug of choice in the treatment of ADHD (3).
The therapeutic effects of methylphenidate are believed to be due to its ability to increase the synaptic concentration of dopamine (4), which it does by blocking the dopamine transporters (5). However, despite the widespread therapeutic use of methylphenidate, the levels of dopamine transporter blockade achieved at the doses used therapeutically in the treatment of ADHD are not known. In addition, while the pharmacokinetics of oral methylphenidate in blood have been widely investigated, there have been no studies of the pharmacokinetics of oral methylphenidate in the primate brain.
An evaluation of the levels of dopamine transporter blockade by oral methylphenidate is also of relevance vis à vis the abuse liability of methylphenidate (6), since dopamine transporters are believed to be the target of cocaine’s reinforcing effects (7). Dopamine transporter-blocking drugs, such as cocaine and methylphenidate, raise the extracellular concentration of dopamine in various brain regions including the nucleus accumbens, which is one of the brain structures associated with the reinforcing effects of drugs of abuse (8).
With positron emission tomography (PET) and appropriate radiotracers, it is now possible to measure the levels of dopamine transporter occupancy achieved by drugs that block the dopamine transporter in human subjects reliably (9) and also to evaluate drug pharmacokinetics directly in brain (9). Using this strategy, we have shown a significant correlation between the magnitude of cocaine-induced dopamine transporter blockade and the self-reports for the “high” as well as a good temporal correspondence between the pharmacokinetics of cocaine at the dopamine transporter and the temporal course for the duration of the “high” (9). Because we have shown that for intravenous cocaine to consistently induce a “high,” it has to block at least 60% of the dopamine transporters, we questioned whether methylphenidate, when given orally and at the doses used therapeutically, wouldachieves these levels of blockade.
To assess the level of dopamine transporter occupancy achieved by different doses of oral methylphenidate in human brain, we selected doses that covered the range used therapeutically for ADHD. The most frequent weight-adjusted doses for methylphenidate correspond to 0.3 to 0.6 mg/kg (10). The behavioral and cardiovascular responses to methylphenidate were monitored during the PET studies. Parallel studies to measure the rate of uptake of oral methylphenidate in brain were performed in a baboon rather than in a human subject in consideration of the radiation dose to the oral mucosa. In addition, because of dosimetry considerations, we conducted the studies in adults rather than in children, for whom methylphenidate is most frequently prescribed.
METHOD
Human Studies
Subjects
Seven healthy subjects (three men and four women) with a mean age of 24 years (SD=7) were studied. Written informed consent was obtained from all subjects after complete description of the study and according to the guidelines set by the Institutional Review Board at Brookhaven National Laboratory.
Scans
PET studies were carried out with a CTI 931 tomograph (reconstructed resolution 6×6×6.5 mm full width at half maximum, 15 slices) by using [11C]cocaine as a dopamine transporter ligand (11). Methods for positioning subjects, catheterizations, transmission scans, and blood sampling and analysis have been published (11). Briefly, emission scans were started immediately after injection of 4–8 mCi of [11C]cocaine (specific activity >0.2 Ci/mol at time of injection). A series of 20 emission scans was obtained from time of injection up to 54 minutes. Arterial sampling was used to quantitate total carbon-11 and unchanged [11C]cocaine in plasma (11).
Subjects were scanned four times with [11C]cocaine over a 2-day period (except for two subjects who were scanned only twice); the first scan on each day was done with oral placebo (calcium carbonate) administered 120 minutes before injection of [11C]cocaine and was used as baseline; the second scan was done 3 hours after the first one and 120 minutes after oral administration of methylphenidate (5 mg, 10 mg, 20 mg, 40 mg, or 60 mg). Table 1 summarizes the total and weight-adjusted doses of oral methylphenidate received by the subjects. The design of the study assumed that the intra- and intersubject variabilities in the dopamine transporter occupancy measures were equivalent. Venous blood was drawn for quantification of plasma concentration of the two enantiomers of methylphenidate (d-threo- and l-threo-methylphenidate) before and at 1, 2, and 3 hours after administration of oral methylphenidate, through use of capillary gas chromatography-mass spectrometry (12).
Behavioral measures
In order to simulate an analog rating scale, participants were instructed to orally respond to each descriptor by using a whole number between 1 and 10 for the self-report of the “high,” “rush,” “restlessness,” and “anxiety” measures. “High” was defined as euphoria, “rush” as the sensation of the drug in the body, and “restlessness” as desire to move. Ratings were obtained every minute for the first 20 minutes and then at 10-minute intervals for the next 40 minutes, as previously described (13). Heart rate and blood pressure were monitored continuously.
Analyses
Regions of interest in striatum and cerebellum were drawn directly on an averaged emission image (images obtained from 10 to 54 minutes), as previously described (11). These regions were then projected into the dynamic images to generate time activity curves for striatum and for cerebellum. These time activity curves for tissue concentration, along with the time activity curves for unchanged tracer in plasma, were used to calculate the distribution volume in striatum and cerebellum through use of a graphical analysis technique for reversible systems (14). We used the ratio of the distribution volume in striatum to that in cerebellum (DVSTR/DVCB), which corresponds to Bmax/Kd +1 and is insensitive to changes in cerebral blood flow (CBF), as model parameter for dopamine transporter availability (15). Dopamine transporter occupancies were calculated as ([Bmax/Kdplacebo – Bmax/Kdmethylphenidate]/Bmax/Kdplacebo) × 100.
Pearson product moment correlation analyses were calculated for the estimates of dopamine transporter occupancy and the concentration of methylphenidate in plasma. Because of the limited number of subjects for each dose, the effects of methylphenidate on the “high” and of the cardiovascular measures were not separately assessed for each dose but were grouped together. Differences between placebo and methylphenidate were compared with paired t tests (two tailed). For the comparison of the cardiovascular variables we averaged the measures obtained between 60 and 115 minutes after placebo or methylphenidate, since this was the time period when most of methylphenidate’s effects were seen. For the comparisons of the “high” measures we chose the peak reported effects. To obtain the estimated dose of methylphenidate required to occupy 50% of dopamine transporter (ED50), the percent occupancy was linearized by plotting the logarithm of P divided by (100 minus P) versus the logarithm of the dose (mg/kg) where P is the percent dopamine transporter occupancy; the linear regression permitted the determination of the ED50, which corresponds to the 0 value on the y axis (16). An equivalent plot was obtained to calculate the plasma concentration of d-threo-methylphenidate required to occupy 50% of the dopamine transporters.
Baboon Studies
To evaluate the rate of uptake of oral methylphenidate in brain we carried out PET studies in one anesthetized (ketamine/isoflurane) female baboon and used [11C]methylphenidate as the radiotracer. Scan procedures, animal preparation and anesthesia (17), and radiotracer synthesis (18) and plasma tracer analysis (19) have been published. In order to administer the radiotracer orally, at the time of endotracheal intubation, we installed an orogastric tube (16–18-inch French gastric tube); positioning was checked by insufflating air and monitoring gastric noise. [11C]Methylphenidate was administered into the orogastric tube as a 10-cc volume, followed by 10-cc flush of water to the gastric tube to clean off any remaining activity. Scanning was started immediately after administration of 20 mCi of [11C]methylphenidate, and dynamic scans were obtained for a total of 2 hours (6×10 minutes; 4×15 minutes). Protocols followed the guidelines of the Brookhaven National Laboratory Institutional Animal Care And Use Committee. Regions of interest in striatum, cerebellum, and whole brain were obtained as previously described (20). Time activity curves for the concentration of the radiotracer in these brain regions were used to estimate the time for the radiotracer to reach peak uptake in brain. Levels of Dopamine Transporter Occupancy as a Function of Plasma Concentration of d-threo-Methylphenidate 120 Minutes After Methylphenidate Administration (A), and Linearized Plots Used to Calculate Plasma Concentration of d-threo-Methylphenidate Associated With 50% Dopamine Transporter Occupancy (plasma ED50) (B) in Seven Normal Adults
RESULTS
Oral methylphenidate produced a dose-dependent blockade of dopamine transporters: means=12% (SD=4%) for 5 mg, 40% (SD=12%) for 10 mg, 54% (SD=5%) for 20 mg, 72% (SD=3%) for 40 mg, and 74% (SD=2%) for 60 mg. Figure 1A shows the levels of dopamine transporter occupancy for the weight-adjusted doses. The estimated dose required to block 50% of the dopamine transporter (ED50 ) was 0.25 mg/kg (figure 1B).
The plasma concentrations for d-threo-methylphenidate are shown in table 2; l-threo-methylphenidate was not detectable. Dopamine transporter occupancies were significantly correlated with the plasma concentration of d-threo-methylphenidate at 120 minutes, which was the time when dopamine transporter measurements were made (r=0.80, df=11, p<0.002). The levels of dopamine transporter occupancy as a function of the concentration of d-threo-methylphenidate at 120 minutes are shown in figure 2A. The plasma concentration of d-threo-methylphenidate, measured 2 hours after administration, that was associated with 50% blockade of the dopamine transporters was estimated to be 6 ng/ml (figure 2B).
Only one of the subjects reported a moderate “high” for one of the methylphenidate doses, whereas the others reported no or minimal “high” (table 1). Thus, even subjects who showed more than 60% dopamine transporter blockade did not report a “high” (figure 3). Methylphenidate induced mild but significant increases in heart rate (placebo: mean=64%, SD=10%; methylphenidate: mean=71%, SD=7%) (t=3.2, df=11, p<0.008) and in systolic (placebo: mean=117, SD=14; methylphenidate: mean=120, SD=12) (t=2.3, df=11, p<0.04) and diastolic (placebo: mean=69, SD=5; methylphenidate: mean=73, SD=4) (t=2.9, df=11, p<0.02) blood pressure (table 1). Group sizes were too small to assess dose effects.
The baboon study showed that [11C]methylphenidate did not reach peak concentration in brain until after 60 minutes of its administration (figure 4). The short half-life of carbon-11 precluded an estimation of the clearance rate of [11C]methylphenidate from brain.
DISCUSSION
This study shows that oral methylphenidate is very effective in blocking dopamine transporters. The ED50 for dopamine transporter blockade by oral methylphenidate was estimated to be 0.25 mg/kg, which indicates that at the therapeutic doses used for ADHD (weight-adjusted doses of 0.3 to 0.6 mg/kg), methylphenidate is likely to occupy more than 50% of the dopamine transporters. This study also shows that the time to reach peak uptake of oral methylphenidate in brain was 60 minutes. This value corresponds well with the pharmacokinetics of methylphenidate reported in plasma, as well as with the time course for observing peak behavioral effects after therapeutic doses of oral methylphenidate (21, 22). Unfortunately, because of the short half-life of carbon-11, we were unable to monitor the rate of clearance of methylphenidate in brain; such monitoring would have allowed us todetermine whether there was a correspondence between the duration of its behavioral effects and its presence in brain.
This study shows a correlation between plasma concentrations and dopamine transporter occupancy and suggests that plasma concentration of d-threo-methylphenidate (active enantiomer) is a good indicator for monitoring methylphenidate delivery to brain. It also shows that plasma concentrations of 6 ng/ml of d-threo-methylphenidate, for the measurements taken at 2 hours of drug administration, are associated with 50% blockade of the dopamine transporters. Since plasma levels in excess of 6 ng/ml at 2 hours of drug administration are reported in children with ADHD who receive methylphenidate doses that are clinically effective (23), this suggests than greater than 50% dopamine transporter blockade may be required for therapeutic efficacy. A 0.25-mg/kg dose was estimated to correspond on average to a concentration of 6 ng/ml of d-threo-methylphenidate in plasma, taken 2 hours after drug administration, which is consistent with plasma concentrations reported in adult subjects treated with these doses (24). The variability in plasma methylphenidate concentrations among subjects, which is not unique to this study (23), could reflect differences in drug absorption or metabolism or both among subjects as well as the small groups studied.
It had been postulated that oral methylphenidate has low reinforcing effects because of its rapid metabolism into ritalinic acid, a compound with low psychostimulant actions (25). We had also hypothesized that oral methylphenidate, at the doses used therapeutically, would not induce a “high” because it would not achieve sufficient levels of dopamine transporter blockade. The results from this study do not support these hypotheses and suggest that variables other than poor efficacy at the dopamine transporter are responsible for the low reinforcing effects of oral methylphenidate. An important consideration in analyzing the reinforcing effects of drugs of abuse is the pharmacokinetics of these drugs, since the shorter the interval between intake and perceived effects of the drug, the greater the reinforcing effects of the drug (26, 27). Of particular relevance is the manner of administration of these drugs, since it will affect their brain pharmacokinetics (28, 29). In this study we showed that in the baboon brain, oral methylphenidate did not reach peak plasma concentrations until 60 minutes after its administration. This finding contrasts markedly with the fast rate of uptake seen in the baboon brain after intravenous administration of methylphenidate (8–10 minutes) (30) or cocaine (4–6 minutes) (14). In this respect it is worthwhile to note that while it has been shown that methylphenidate is abused by humans, such abuse occurs predominantly with intravenous rather than with oral administration (6). Similarly, studies in nonhuman primates that have shown comparable levels of self-administration for methylphenidate and cocaine have used intravenous administration (31, 32).
The dependency of the reinforcing effects of drugs on their rate of brain uptake could provide an explanation of why the subjects in this study did not report a “high” even when oral methylphenidate was given at doses that achieved levels of dopamine transporter blockade comparable to those of intravenous doses of cocaine (9) or of intravenous doses of methylphenidate (33), which induced a “high” under similar experimental conditions. These findings, in light of previous results showing that it is the rapid onset of blockade of the dopamine transporter, rather than continuous blockade, that is associated with the “high” induced by intravenous methylphenidate (34), suggest that oral methylphenidate’s uptake in brain may be too slow to induce a “high.” In contrast to our results, others have reported mild reinforcing effects with similar doses of oral methylphenidate (35). This may reflect differences in experimental conditions or the questionnaires used or both. In addition, the group sizes in the present study may have been too small to detect behavioral effects.
In interpreting the findings from this study, it is important to comment on the model used in the study to assess dopamine transporter occupancy (Bmax/Kd=DVSTR/DVCB–1). The distribution volume is an “equilibrium” measure representing the ratio of tissue radioactivity (metabolite corrected) to plasma radioactivity. Since it is an equilibrium property, it does not depend on blood flow. The distribution volume calculated for [11C]cocaine with the graphical method has been shown to be the same as that obtained from a compartmental model approach and to be a stable measure that does not change with time (14). Furthermore, estimates of dopamine transporter occupancy have been shown to be reproducible (9) and to lead to similar measures whether one uses [11C]cocaine or [11C]d-threo-methylphenidate as the dopamine transporter radioligand (36). In interpreting the findings from this study, it is also important to realize that while the self-report of “high” has been shown to be a reliable and accurate predictor for drug self-administration in humans, it is not the only variable accounting for drug-seeking behavior (37).
Limitations of this study include the fact that the studies were done in adults and not in children with ADHD and that the group size was small, which precluded an accurate analysis of methylphenidate’s behavioral effects. Furthermore, dopamine transporter measurements were made at 120 minutes and not at 60 minutes, when peak brain uptake was observed, so that peak occupancies may have been underestimated. In addition, while this study showed the levels of dopamine transporter occupancy obtained after various doses of methylphenidate, future studies are required to determine the levels of dopamine transporter blockade associated with methylphenidate’s therapeutic efficacy in ADHD.
This study measures for the first time the levels of dopamine transporter blockade achieved after therapeutic doses of oral methylphenidate. It shows that oral methylphenidate is very effective in blocking dopamine transporters and that at the doses used therapeutically for ADHD, it may block more than 50% of the dopamine transporters. It also shows that oral methylphenidate did not reach peak brain concentrations until 60 minutes after its administration. The slow rate of brain uptake of oral methylphenidate may explain the failure to induce significant self-reports of “high.” These results highlight the significant role that manner of administration has on the ability of methylphenidate to elicit a “high” and indicate that the “level,” as well as the “rate,” of dopamine transporter blockade contributes to the “high.” Further studies to elucidate the mechanism or mechanisms responsible for the “rate” dependency should be encouraged.
Received Jan. 23, 1998; revision received April 14, 1998; accepted June 19, 1998. From the Medical and Chemistry Departments, Brookhaven National Laboratory; and the Department of Psychiatry, State University of New York at Stony Brook, Stony Brook, N.Y.. Address reprint requests to Dr. Volkow, Medical Department, Brookhaven National Laboratory, Upton, NY 11973; [email protected] (e-mail). Supported by U.S. Department of Energy Office of Health and Environmental Research contract DE-ACO2-76CH-00016 and by National Institute on Drug Abuse grant DA-09490.The authors thank D. Schlyer for Cyclotron operations; A. Levy and D. Warner for PET operations; C. Wong for data management; R. Ferrieri, K Shea, R. MacGregor, and P. King for radiotracer preparation and analysis; P. Cerveny and N. Netusil for patient care; and T. Cooper for plasma methylphenidate analyses.
![]() |
![]() |
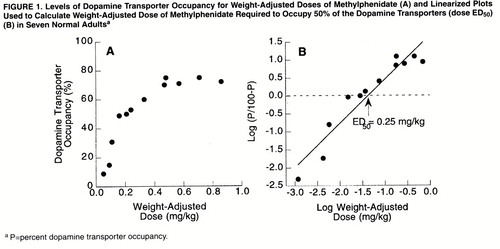

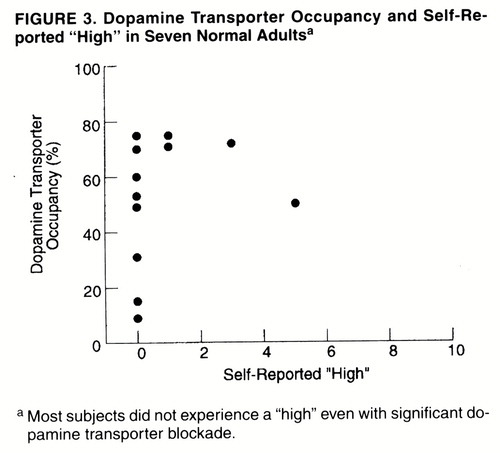
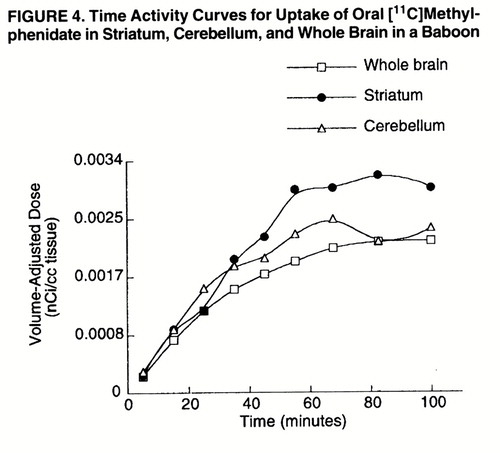
1. Swanson JM, Seargeant JA, Taylor E, Sonuga-Barke EJS, Jensen PS, Cantwell DP: Attention deficit disorder and hyperkinetic disorder. Lancet 1998; 351:429–433Crossref, Medline, Google Scholar
2. Swanson JM, Lerner M, Williams L: More frequent diagnosis of attention deficit-hyperactivity disorder (letter). N Engl J Med 1995; 333:944Crossref, Medline, Google Scholar
3. Carrey NJ, Wiggins DM, Milin RP: Pharmacological treatment of psychiatric disorders in children and adolescents: focus on guidelines for the primary care practitioner. Drugs 1996; 51:750–759Crossref, Medline, Google Scholar
4. Castellanos XF, Elia J, Kruesi MJ, Narsh WL, Gulotta CS, Potter WZ, Ritchie GF, Hamburger SD, Rapoport JL: Cerebrospinal fluid homovanillic acid predicts behavioral response to stimulants in 45 boys with attention/hyperactivity disorder. Neuropsychopharmacology 1996; 14:125–137Crossref, Medline, Google Scholar
5. Schweri MM, Skolnick P, Rafferty MF, Rice KC, Janowsky AJ, Paul SM: [3H]Threo-(+/–)-methylphenidate binding to 3,4-dihydroxyphenyl ethylamine uptake sites in corpus striatum: correlation with the stimulant properties of ritalinic acid esters. J Neurochem 1985; 45:1062–1070Crossref, Medline, Google Scholar
6. Parran TV, Jasinski DR: Intravenous methylphenidate abuse: prototype for prescription drug abuse. Arch Intern Med 1991; 151:781–783Crossref, Medline, Google Scholar
7. Ritz MC, Lamb RJ, Goldberg SR, Kuhar MJ: Cocaine receptors on dopamine transporters are related to self-administration of cocaine. Science 1987; 237:1219–1223Crossref, Medline, Google Scholar
8. Koob GF, Bloom FE: Cellular and molecular mechanism of drug dependence. Science 1988; 242:715–723Crossref, Medline, Google Scholar
9. Volkow ND, Wang GJ, Fischman MW, Foltin RW, Fowler JS, Abumrad NN, Vitkun S, Logan J, Gatley SJ, Pappas N, Hitzemann R, Shea K: Relationship between subjective effects of cocaine and dopamine transporter occupancy. Nature 1997; 386:827–830Crossref, Medline, Google Scholar
10. Greenhill LL, Abikoff HB, Arnold E, Cantwell DP, Conners CK, Elliot G, Hechtman L, Hinshaw SP, Hoza B, Jensen PS, March J, Newcorn J, Pelham WF, Severe JB, Swanson JM, Vitiello B, Wells K: Medication treatment strategies in the MTA study: relevance to clinicians and researchers. J Am Acad Child Adolesc Psychiatry 1996; 34:1–10Google Scholar
11. Fowler JS, Volkow ND, Wolf AP, Dewey SL, Schlyer DJ, MacGregor R, Hitzemann R, Logan J, Bendriem B, Gatley J, Christman D: Mapping cocaine-binding sites in human and baboon brain in vivo. Synapse 1989; 4:371–377Crossref, Medline, Google Scholar
12. Srinivas NR, Hubbard JW, Quinn D, Korchinski ED, Midha K: Extensive and enantioselective presystemic metabolism of dl-threo-methylphenidate in humans. Prog Neuropsychopharmacol Biol Psychiatry 1991; 15:213–220Crossref, Medline, Google Scholar
13. Wang G-J, Volkow ND, Hitzemann RJ, Wong C, Angrist B, Burr G, Pascani K, Pappas N, Lu A, Cooper T, Lieberman JA: Behavioral and cardiovascular effects of intravenous methylphenidate in normal subjects and cocaine abusers. European Addiction Res 1997; 3:49–54Crossref, Google Scholar
14. Logan J, Fowler JS, Volkow ND, Wolf AP, Dewey SL, Schlyer D, MacGregor RR, Hitzemann R, Bendriem B, Gatley SJ, Christman DR: Graphical analysis of reversible radioligand binding from time activity measurements applied to [N-11C-methyl]-(-)cocaine PET studies in human subjects. J Cereb Blood Flow Metab 1990; 10:740–747Crossref, Medline, Google Scholar
15. Logan J, Volkow ND, Fowler JS, Wang G-J, Dewey SL, MacGregor R, Schlyer D, Gatley SJ, Pappas N, King P, Hitzemman R, Vitkun S: Effects of blood flow on [11C]raclopride binding in the brain: model simulations and kinetic analysis of PET data. J Cereb Blood Flow Metab 1994; 14:995–1010Crossref, Medline, Google Scholar
16. Keen M, MacDermot J: Analysis of receptors by radioligand binding, in Receptor Autoradiography: Principles and Practice. Edited by Wharton J, Polak JM. Oxford, England, Oxford University Press, 1993, pp 50–51Google Scholar
17. Dewey SL, Smith G, Logan J, Brodie JD, Wei YD, Ferrieri RA, King P, MacGregor R, Martin PT, Wolf AP, Volkow ND, Fowler JS: GABAergic inhibition of endogenous dopamine release measured in vivo with 11C-raclopride and positron emission tomography. J Neurosci 1992; 12:3773–3780Crossref, Medline, Google Scholar
18. Ding Y-S, Sugano Y, Fowler JS, Salata C: Synthesis of the racemate and individual enantiomers of [11C]methylphenidate for studying presynaptic dopaminergic neurons with positron emission tomography. J Labeled Compounds and Radiopharmacology 1994; 34:989–997Crossref, Google Scholar
19. Alexoff DL, Shea C, Fowler JS, King P, Gatley SJ, Schlyer D J, Wolf AP: Plasma input function determination for PET using a commercial laboratory robot. Nucl Med Biol 1995; 22:893–904Crossref, Medline, Google Scholar
20. Volkow ND, Fowler JS, Logan J, Gatley JS, Dewey SL, MacGregor RR, Schlyer DJ, Pappas N, King P, Wolf AP: Carbon-11-cocaine binding compared at sub-pharmacological and pharmacological doses: a PET study. J Nucl Med 1995; 36:1289–1297Medline, Google Scholar
21. Wargin W, Patrick K, Kilts PC, Gualtieri CT, Ellington K, Mueller RA, Kraemer G, Breese GR: Pharmacokinetics of methylphenidate in man, rat and monkey. J Pharmacol Exp Ther 1983; 226:382–386Medline, Google Scholar
22. Shaywitz SE, Hunt RD, Jatlow P, Cohen DJ, Young JG, Pierce RN, Anderson GM, Shaywitz BA: Psychopharmacology of attention deficit disorder: pharmacokinetic, neuroendocrine, and behavioral measures following acute and chronic treatment with methylphenidate. Pediatrics 1982; 69:688–694Medline, Google Scholar
23. Srinivas NR, Hubbard JW, Quinn D, Korchinski ED, Midha K: Enantioselective pharmacokinetics and pharmacodynamics of dl-threo-methylphenidate in children with attention deficit hyperactivity disorder. Clin Pharmacol Ther 1992; 52:561–568Crossref, Medline, Google Scholar
24. Aoyama T, Kotaki H, Sasaki T, Sawada Y, Honda Y, Iga T: Nonlinear kinetics of threo-methyphenidate enantiomers in a patient with narcolepsy and in healthy volunteers. Eur J Pharmacol 1993; 44:79–84Crossref, Google Scholar
25. Faraj GA, Israili JM, Perel ML, Jenjins ML, Holtzaman SG, Cucinell SA, Dayton PG: Metabolism and disposition of methylphenidate 14C: studies in man and animals. J Pharmacol Exp Ther 1974; 191:535–547Medline, Google Scholar
26. Oldendorf WH: Some relationships between addiction and drug delivery to the brain. NIDA Res Monogr 1992; 120:13–25Medline, Google Scholar
27. Balster RL, Schuster CR: Fixed-interval schedule of cocaine reinforcement: effects of dose and infusion duration. J Exp Anal Behav 1973; 20:119–129Crossref, Medline, Google Scholar
28. Verebey K, Gold MS: From coca leaves to crack: the effects of dose and routes of administration in abuse liability. Psychiatr Annals 1988; 18:513–520Crossref, Google Scholar
29. Perez-Reyes M, DiGuiseppi S, Ondrusek G, Jeffcoat AR, Cook CE: Free-base cocaine smoking. Clin Pharmacol Ther 1982; 32:459–465Crossref, Medline, Google Scholar
30. Ding Y-S, Fowler JS, Volkow ND, Gatley SJ, Logan J, Dewey S, Alexoff D, Wolf AP: Pharmacokinetics and in vivo specificity of [11C]dl-threo-methylphenidate for the presynaptic dopaminergic neuron. Synapse 1994; 18:152–160Crossref, Medline, Google Scholar
31. Johanson CE, Shuster CR: A choice procedure for drug reinforcers: cocaine and methylphenidate in the rhesus monkey. J Pharmacol Exp Ther 1975; 193:676–688Medline, Google Scholar
32. Bergman J, Madras B, Johnson SE, Spealman RD: Effects of cocaine and related drugs in nonhuman primates, III: self administration by squirrel monkeys. J Pharmacol Exp Ther 1989; 251:150–155Medline, Google Scholar
33. Volkow ND, Wang G-J, Fowler JS, Gatley SJ, Ding Y-S, Logan J, Dewey SL, Hitzemann R, Lieberman J: Relationship between psychostimulant induced high and dopamine transporter occupancy. Proc Natl Acad Sci USA 1996; 93:10388–10392Crossref, Medline, Google Scholar
34. Volkow ND, Ding Y-S, Fowler JS, Wang GJ, Logan J, Gatley JS, Dewey SL, Ashby C, Lieberman J R Hitzemann, Wolf AP: Is methylphenidate like cocaine? studies on their pharmacokinetics and distribution in human brain. Arch Gen Psychiatry 1995; 52:456–463Crossref, Medline, Google Scholar
35. Chait LD: Reinforcing and subjective effects of methylphenidate in humans. Behavioral Pharmacology 1994; 5:281–288Crossref, Medline, Google Scholar
36. Fowler JS, Volkow ND, Logan J, Gatley SJ, Pappas N, King P, Ding Y-S, Wang GJ: Comparison of [11C]cocaine and [11C]d-threo-methylphenidate for estimating dopamine transporter occupancy by cocaine in vivo. Synapse 1998; 28:111–116Crossref, Medline, Google Scholar
37. Fischman MW, Foltin RW: Utility of subjective-effects measurements in assessing abuse liability of drugs in humans. Br J Addict 1991; 86:1563–1570Crossref, Medline, Google Scholar