Cerebral Glucose Metabolism in Obsessive-Compulsive Hoarding
Abstract
OBJECTIVE: Compulsive hoarding and saving symptoms, found in many patients with obsessive-compulsive disorder (OCD), are part of a discrete clinical syndrome that includes indecisiveness, disorganization, perfectionism, procrastination, and avoidance and has been associated with poor response to medications and cognitive behavior therapy. The authors sought to identify cerebral metabolic patterns specifically associated with the compulsive hoarding syndrome using positron emission tomography (PET). METHOD: [18F]Fluorodeoxyglucose PET scans were obtained for 45 adult subjects who met DSM-IV criteria for OCD (12 of whom had compulsive hoarding as their most prominent OCD symptom factor) and 17 normal comparison subjects. All subjects had been free of psychotropic medication for at least 4 weeks. Regional cerebral glucose metabolism was compared between the groups. RESULTS: In relation to the comparison subjects, the patients with compulsive hoarding syndrome had significantly lower glucose metabolism in the posterior cingulate gyrus and cuneus, whereas the nonhoarding OCD patients had significantly higher glucose metabolism in the bilateral thalamus and caudate. In relation to nonhoarding OCD patients, compulsive hoarders had significantly lower metabolism in the dorsal anterior cingulate gyrus. Across all OCD patients, hoarding severity was negatively correlated with glucose metabolism in the dorsal anterior cingulate gyrus. CONCLUSIONS: OCD patients with the compulsive hoarding syndrome had a different pattern of cerebral glucose metabolism than nonhoarding OCD patients and comparison subjects. Obsessive-compulsive hoarding may be a neurobiologically distinct subgroup or variant of OCD whose symptoms and poor response to anti-obsessional treatment are mediated by lower activity in the cingulate cortex.
Although standard diagnostic classifications consider obsessive-compulsive disorder (OCD) to be a single entity, it has become clear that several different symptom dimensions of OCD exist. Large-scale factor and cluster analytic studies of OCD have identified four principal symptom factors: 1) aggressive, sexual, and religious obsessions with checking compulsions; 2) symmetry obsessions with ordering, arranging, and repeating compulsions; 3) contamination obsessions with washing and cleaning compulsions; and 4) hoarding, saving, and collecting symptoms (1–3). These symptom dimensions appear to be relatively stable over time (4). Different symptom factors show different patterns of genetic inheritance (5) and comorbidity (6). Perhaps most important, the symptom factors differ in their response to treatment (7–11). Thus, OCD appears to be a multidimensional and etiologically heterogeneous disorder. Defining the neurobiological mediation of OCD symptom factors is important for establishing more specific phenotypes for research studies and may be critical in the development of more effective treatments.
Hoarding is defined as the acquisition of, and inability to discard, worthless items even though they appear (to others) to have no value (12). Hoarding and saving behavior has been observed in several neuropsychiatric disorders, including schizophrenia, dementia, eating disorders, autism, and mental retardation (13), as well as in nonclinical populations (14), but it is most commonly found in patients with OCD. Approximately 18%–42% of patients with OCD have hoarding and saving compulsions (6, 14–16), and about 10%–20% of all OCD patients are thought to have the compulsive hoarding syndrome as their most prominent and distressing type of OCD.
Frost and colleagues (14) have argued persuasively that hoarding and saving symptoms are part of a discrete clinical syndrome that also includes indecisiveness, perfectionism, procrastination, difficulty organizing tasks, and avoidance. Compulsive hoarding is most commonly driven by obsessional fears of losing important items that the patient believes will be needed later (13), distorted beliefs about the importance of possessions, and excessive emotional attachments to possessions (12). Hoarders usually fear making “wrong decisions” about what to discard and what to keep, so they acquire and save items to prepare for every imaginable contingency. The most commonly saved items include newspapers, magazines, old clothing, bags, books, mail, notes, and lists (8, 12). Living spaces become sufficiently cluttered to preclude the activities for which they were designed, causing significant impairment in social and/or occupational functioning (17, 18). A survey of elderly hoarders found that hoarding constituted a physical health threat to 81% of identified patients (19), including the threat of fire hazard, falling, unsanitary conditions, and an inability to prepare food. Hoarders often have less insight into their symptoms than do nonhoarding patients with OCD (20), making them less likely to seek treatment.
The few studies that have directly compared patients with the compulsive hoarding syndrome to nonhoarding patients with OCD found that compared to nonhoarding OCD patients, hoarders had more severe family and social disability, anxiety, depression, and personality disorder symptoms (17); lower global functioning (18); and higher rates of hoarding and tics in their first-degree relatives (6). Compulsive hoarding also has a different pattern of genetic inheritance than other OCD symptom factors. The hoarding/saving symptom factor has a recessive inheritance pattern, whereas the aggressive/checking and symmetry/order symptom factors show a dominant pattern (5). A genome-wide scan conducted in sibling pairs with Gilles de la Tourette’s syndrome found that the hoarding phenotype was significantly associated with genetic markers on chromosomes 4q34-35, 5q35.2-35.3, and 17q25 (21). Taken together, these studies suggest that compulsive hoarding syndrome is a genetically distinct subgroup or variant of OCD (7) with a characteristic pattern of associated symptoms and functional disability.
Compulsive hoarding syndrome has often proven refractory to standard treatments for OCD. In several studies of OCD treatment, hoarding and saving compulsions have been strongly associated with poor response to pharmacotherapy with selective serotonin reuptake inhibitors (7–9) as well as cognitive behavior therapy (10, 11). Thus, the hoarding phenotype is a clear, reliable predictor of poor treatment response in OCD. In order to develop more effective treatments for this syndrome, it is crucial to elucidate its pathophysiology.
Animal studies suggest that hoarding may be mediated by the ventromedial striatum, the globus pallidus, and the medial dorsal thalamus (22), structures that are also implicated in human OCD (23). Electrical stimulation and lesion studies have also implicated the anterior cingulate gyrus (24), the hypothalamus (25), the hippocampus, and the septum (26) in the mediation of food hoarding behavior in rodents. Food hoarding is also stimulated by dopamine agonists (27) but is reduced by lesions of dopaminergic pathways (28, 29), as well as by administration of serotonin agonists (30). Gonadal steroids (31), benzodiazepines (32), and opiates (33) may also play roles in modulating hoarding behavior in animals. It is unclear whether food hoarding in rodents is a useful model for compulsive hoarding in OCD. However, despite its common occurrence and clinical significance, to our knowledge, no study to date has investigated the neurobiology of compulsive hoarding syndrome in human patients.
Functional brain imaging research has led to a greater understanding of the neurobiological mediation of OCD. Various positron emission tomography (PET) studies of OCD have found elevated glucose metabolic rates in the orbitofrontal cortex, the anterior cingulate gyrus, the caudate nuclei, and the thalamus (34–40) that normalize with response to treatment (39, 41–46). Interventions that provoke OCD symptoms have been found to increase activity in these same brain regions (47–50). These and other findings have led to the theory that the symptomatic expression of OCD is mediated by hyperactivity along specific frontal-subcortical circuits (51) connecting the orbitofrontal cortex, the ventromedial caudate, the globus pallidus, and the medial dorsal nucleus of the thalamus (52–55; see reference 56 for a review).
Although functional neuroimaging studies in OCD have yielded more consistent results than for other psychiatric disorders, there is heterogeneity in their findings that might be accounted for by phenotypical variations between their subject pools. Virtually all prior neurobiological and treatment studies of OCD have grouped patients with diverse symptom patterns together. Only one neuroimaging study to date has examined the neural correlates of symptom dimensions in patients with OCD (57), finding that the severity of factor 1 symptoms correlated with regional cerebral blood flow (rCBF) in the striatum, while factor 2 symptoms had a less-than-significant negative correlation with rCBF in the right striatum. The severity of factor 3 symptoms correlated with rCBF in the left orbitofrontal cortex, the bilateral anterior cingulate gyrus, and other cortical areas. Although these were preliminary results, they suggested that different OCD symptom clusters were mediated by quite different patterns of brain activity.
In a subsequent study using OCD symptom provocation in patients with factor 3 (contamination/washing) symptoms, the same research group replicated the correlation between the severity of factor 3 symptoms and rCBF in the orbitofrontal cortex and the anterior cingulate gyrus (58). Of note, factor 4 symptoms (hoarding and saving) were not included in either of these studies. Thus, it is unclear whether the patients with the hoarding/saving subtype of OCD have the same abnormalities in brain activity as seen in other OCD patients. A recent study found that provocation of hoarding-related anxiety in normal volunteers activated ventral prefrontal regions and the left amygdala (59), but it remains unknown whether these regions mediate the characteristic symptoms and deficits that comprise the compulsive hoarding syndrome in patients.
We sought to determine whether the compulsive hoarding syndrome is associated with unique abnormalities of brain function that are different from those seen in other OCD patients by using PET to identify cerebral metabolic patterns specifically associated with compulsive hoarding syndrome.
Method
Subjects
This study was carried out under guidelines established by the institutional review board of the University of California at Los Angeles. Subjects were recruited from the Los Angeles area between 1994 and 1999 with approved advertisements in flyers, newspapers, and web sites. Sixty-two subjects were studied: 45 patients with OCD and 17 comparison subjects with no history of neuropsychiatric disorders. Diagnostic classifications were made by clinical interview with DSM-IV criteria and confirmed with the Schedule for Affective Disorders and Schizophrenia—Lifetime Version (60). Symptom severity and level of functioning were rated with the Yale-Brown Obsessive Compulsive Scale (61) and checklist, the 17-item Hamilton Depression Rating Scale (62), the Hamilton Anxiety Rating Scale (63), and the Global Assessment Scale (GAS) (64). The presence of all OCD symptoms, including hoarding/saving symptoms, was assessed prospectively in all subjects at study entry with the Yale-Brown Obsessive Compulsive Scale and its symptom checklist. To be enrolled in the study, the patients had to meet DSM-IV criteria for OCD and have a pre-treatment Yale-Brown Obsessive Compulsive Scale score of at least 16. These criteria were chosen based on prior use in several studies of OCD (65–67). All diagnostic assessments and rating scales were performed by a study psychiatrist with training in standardized assessment (S.S. or A.L.B.).
For each subject, the severity of the hoarding/saving symptoms was retrospectively rated on a 0–4 scale (0=none, 1=mild, 2=moderate, 3=severe, 4=extreme) with the method of Rauch et al. (58) for rating the severity of OCD symptom factors. OCD patients were classified retrospectively as having the compulsive hoarding syndrome if
1. | Compulsive hoarding/saving was their most prominent, distressing, and impairing OCD symptom factor, as determined by clinical interview and the Yale-Brown Obsessive Compulsive Scale checklist. | ||||
2. | They met the clinical criteria for compulsive hoarding developed by Frost et al. (14), requiring clutter that precludes use of living spaces and significant functional impairment due to hoarding. | ||||
3. | They had a hoarding severity score of at least 3. |
Of the 45 OCD patients, 12 met those criteria and were diagnosed with compulsive hoarding syndrome.
All subjects were in good physical health. Of the 45 patients with OCD, 16 had comorbid major depression, including three of the 12 compulsive hoarders (25%) and 13 of the 33 nonhoarding OCD patients (39%). Five patients with OCD had comorbid tic disorders. Subjects with other concurrent axis I diagnoses, including bipolar disorder, psychotic disorders, other anxiety disorders, substance abuse, or concurrent medical conditions affecting brain function (i.e., Parkinson’s disease, diabetes mellitus, etc.) were excluded. All subjects had been free of psychoactive medications for at least 4 weeks before entering the study and from fluoxetine for at least 5 weeks. Only four subjects had received any psychotropic medication within 12 weeks of entering the study. A total of 15 of the 45 OCD patients had never before been treated with psychotropic medications. After complete description of the study to the subjects, written informed consent was obtained.
Image Acquisition
PET methods were as detailed in previous reports (40, 45). In brief, each subject received 5–10 mCi of [18F]fluorodeoxyglucose (FDG) while in a supine position with eyes and ears open. The subjects were closely monitored to make sure that they remained awake and lying still without moving or talking during the 40-minute FDG-uptake period. No cognitive task was given. PET scanning was performed on Siemens-CTI PET tomographs (CTI, Knoxville, Tenn.): the ECAT III 831 (15 transverse sections spaced 6.75 mm apart with 6 mm of in-plane spatial resolution, acquired at an angle parallel to the canthomeatal plane) for the first 24 subjects and the EXACT HR1 961 (47 transverse sections spaced 4.0 mm apart with 3.6 mm of in-plane spatial resolution) for the next 38 subjects.
Each subject also received a magnetic resonance imaging (MRI) scan of the brain with a double-echo sequence (proton density and T2 images: TR=2000 to 2500 msec, TE=25 to 30 msec and 90 to 110 msec, 24 cm field of view, 3 mm slices with 0 mm separation). All MRI scans were reviewed by a neuroradiologist. Any prospective subject with MRI evidence of structural CNS lesions was excluded from the study.
Image Analysis
Two methods of image analysis were employed: 1) MRI-based region-of-interest analysis and 2) statistical parametric mapping (SPM) (68, 69). The region-of-interest analysis allowed for comparisons of glucose metabolism in brain regions chosen a priori, based on previous findings in OCD. SPM was used for two reasons. First, the drawn regions of interest were relatively large, and SPM allowed examination of smaller areas within the regions of interest that might have significant findings but might not be detected in the region-of-interest analysis because adjacent areas did not. Second, SPM could perform an exploratory, whole-brain search of the rest of the brain for significant findings outside of our a priori hypothesized regions of interest. Results from the two methods were compared, given the limitations of each (69, 70).
MRI-based region-of-interest analysis involved coregistering each subject’s FDG PET scans with his or her MRI scan (71) then outlining regions of interest on horizontal planes of the MRI scan, as previously described (40, 45). CSF and white matter were excluded from the outlines of all gray matter regions of interest. Regions of interest were drawn by technicians who were blind to subject identity and diagnosis and were reviewed by S.S. and A.L.B to ensure interrater reliability. Nine bilateral regions of interest were selected a priori based on previous associations with OCD symptoms, depressive symptoms, or response to treatment: the dorsolateral prefrontal cortex, ventrolateral prefrontal cortex, orbitofrontal cortex, dorsal anterior cingulate gyrus, ventral anterior cingulate gyrus, caudate, thalamus, amygdala, and hippocampus. Boundaries for these regions were defined with standard atlases (72, 73). The dorsolateral prefrontal cortex consisted of the dorsal half of the middle frontal gyrus, and the ventrolateral prefrontal cortex consisted of its ventral half (74). The orbitofrontal cortex region of interest included the medial and lateral orbital gyri, the orbital part of the inferior frontal gyrus, and the most inferior part of the frontal pole, but it excluded the gyrus rectus. The anterior cingulate gyrus was divided evenly into dorsal and ventral portions. The superior boundary of the dorsal anterior cingulate gyrus was the base of the body of the cingulate gyrus, while the inferior boundary was parallel to the middle of the body of the caudate. The caudate region of interest included the entire head but excluded the body and tail of the caudate nucleus. Amygdala and hippocampal regions of interest excluded the mesial temporal cortex and the parahippocampal gyrus. Both supratentorial hemispheres were also drawn.
Regions of interest drawn on each subject’s MRI were transferred onto his or her coregistered PET scans. Mean activity in each region-of-interest volume and ratios of each region of interest normalized to ipsilateral hemispheric glucose metabolism (region of interest/hemisphere) were calculated as previously described (40, 45). Absolute glucose metabolic rates could not be calculated accurately or reliably for many PET scans in this study because of errors in gamma counter calibration and blood glucose measurement. Therefore, only regional metabolic data normalized to each subject’s ipsilateral hemisphere were used for the MRI-based region-of-interest analysis. This also made the region-of-interest and SPM analyses more congruent since SPM data were also normalized and proportionally scaled to group means.
SPM analysis of PET data employed the software package SPM 96 (75). PET images were coregistered and spatially normalized to the standardized coordinate system of Talairach and Tournoux (76). Normalization by proportional scaling was used. A 16-mm full width at half-maximum, three-dimensional Gaussian smoothing filter was applied to all images. Two OCD subjects had PET scans that could not be normalized properly to the standard template and so were excluded from the SPM analyses. To determine the location of the SPM findings, MRIs of all study subjects were transformed into Talairach space, and clusters with significant correlations were mapped onto a group-averaged MRI. Voxel coordinates were also located in the standard atlas (76).
Statistical Analyses
The data were first screened for distributional properties, outliers, and missing values. No variables were rejected by this process. Demographic variables were compared between the three groups: compulsive hoarders (N=12), nonhoarding patients with OCD (N=33), and normal comparison subjects (N=17). Age was compared between groups with a one-way analysis of variance (ANOVA) (SPSS version 6.1.2 for Macintosh, SPSS, Chicago), with post hoc least significant differences tests to determine which diagnostic group accounted for significant between-group differences (p<0.05), while the proportion of women in each group was compared with a chi-square test. Baseline symptom severity scores (Yale-Brown Obsessive Compulsive Scale, Hamilton depression scale, Hamilton anxiety scale, GAS, and hoarding severity) were compared between the two patient groups—compulsive hoarders (N=12) and nonhoarding OCD patients (N=33)—with t tests (two-tailed) for independent samples.
Both SPM and MRI-based region-of-interest analyses were used to identify significant differences in regional cerebral glucose metabolism between the three groups: compulsive hoarders, nonhoarding OCD patients, and normal comparison subjects. For the region-of-interest analysis, region of interest/hemisphere values were compared between the three groups with omnibus multivariate analysis of variance (MANOVA), using diagnostic group as a between-subject factor and normalized glucose metabolic rates in the selected regions of interest as the dependent variables, with age, gender, scanner type, and Hamilton depression scale score as covariates. Two omnibus MANOVAs were performed, one for all OCD-related regions of interest (the caudate, the thalamus, the orbitofrontal cortex, and the ventral anterior cingulate gyrus) and one for depression-related regions of interest (the dorsolateral prefrontal cortex, the ventrolateral prefrontal cortex, the dorsal anterior cingulate gyrus, the hippocampus, and the amygdala). Wilks’s lambda statistic was used to determine whether the diagnostic groups were significantly different across all comparisons of metabolic rates. Univariate ANOVAs were then performed, followed by post hoc pairwise comparisons for only the regions of interest found to have significant effects of diagnosis in the univariate ANOVAs, to determine which diagnostic groups accounted for significant between-group differences (p<0.05). This method reduced the likelihood of type II error from multiple comparisons.
For SPM analysis, cerebral metabolic differences between groups were assessed with the z statistic on a voxel-by-voxel basis to identify the profile of voxels that differed significantly between groups. Age, gender, scanner type, and Hamilton depression scale score were entered into the model for each between-group comparison as nuisance covariates. Only clusters larger than 200 voxels were reported as significant. A significance threshold of p<0.005 at the uncorrected voxel level was used. This threshold was similar to those used in other published studies of mood and anxiety disorders using SPM (44, 77). Results are presented with the voxel of peak significance.
To determine associations between the severity of compulsive hoarding/saving symptoms and baseline regional cerebral glucose metabolism, data from all 45 OCD patients were pooled and both analyses based on region of interest and whole-brain SPM were performed. For the MRI-based region-of-interest data analysis, partial correlation coefficients (two-tailed) were calculated between pretreatment region of interest/hemisphere values and hoarding severity score, covarying for age, gender, scanner type, and Hamilton depression scale score. For the SPM analysis, linear regression was performed with glucose metabolic rates as dependent variables and hoarding severity as an independent variable. Age, gender, scanner type, and Hamilton depression scale score were entered into the model as nuisance covariates. The SPM program performed a separate linear regression for every voxel in which the data were the voxel-normalized metabolic rates for every scan. In the regressions, the variance of the voxel-normalized metabolic rate was taken to be independent of scan and voxel. Voxels with linear relationships between normalized glucose metabolism and hoarding severity were considered significant at an uncorrected p<0.005.
Results
Compulsive hoarders were significantly older than nonhoarding OCD patients and comparison subjects (ANOVA: F=15.0, df=2, 59, p<0.001; least significant differences p<0.05) (Table 1). There was a significantly lower proportion of women in the nonhoarding group with OCD than in the group of compulsive hoarders or the group of normal comparison subjects (χ2=11.65, df=2, p=0.003). Compulsive hoarders and nonhoarding patients with OCD did not significantly differ from one another in scores on the Yale-Brown Obsessive Compulsive Scale, the Hamilton depression scale, the Hamilton anxiety scale, or the GAS, but compulsive hoarders scored significantly higher than nonhoarders on the hoarding severity scale (t=16.2, df=43, p<0.001) (Table 1).
MRI-Based Region-of-Interest Results
A significant effect of diagnosis was found in the omnibus MANOVA comparing OCD-related region of interest/hemisphere values in compulsive hoarders, nonhoarding OCD patients, and comparison subjects (Wilks’s lambda=0.58; F=1.86, df=16, 96, p=0.03). Univariate ANOVAs revealed a significant effect of diagnostic group for the right thalamus (F=5.88, df=2, 55, p=0.005), the left thalamus (F=4.29, df=2, 55, p=0.02), and the right caudate (F=3.84, df=2, 55, p=0.03) (Table 2). Nonhoarding OCD patients had significantly higher right thalamic metabolism than both the comparison subjects (estimated marginal data: mean=1.10, SE=0.01, versus mean=1.02, SE=0.02; pairwise p=0.003) and the compulsive hoarders (mean=1.10, SE=0.01, versus mean=1.06, SE=0.02; pairwise p=0.05). Nonhoarding OCD patients also had significantly higher left thalamic metabolism than either the comparison subjects (mean=1.10, SE=0.01, versus mean=1.02, SE=0.02; pairwise p=0.02) or the compulsive hoarders (mean=1.10, SE=0.01, versus mean=1.04, SE=0.02; pairwise p=0.05). The nonhoarding OCD patients had significantly higher right caudate metabolism than the comparison subjects (mean=1.20, SE=0.01, versus mean=1.12, SE=0.02; pairwise p=0.008). No significant effect of diagnosis was found in the omnibus MANOVA comparing depression-related region of interest/hemisphere values in the compulsive hoarders, the nonhoarding OCD patients, and the comparison subjects (Wilks’s lambda=0.78; F=0.91, df=28, 84, p=0.89). No significant correlation was found between hoarding severity score and any region of interest/hemisphere ratio.
SPM Results
In relation to the comparison subjects, the compulsive hoarders had significantly lower cerebral glucose metabolism in the right posterior cingulate gyrus (Talairach coordinates: x=10, y=–50, z=36; z score=2.71, p=0.003) and the bilateral cuneus (Talairach coordinates: x=–2, y=–84, z=30; z score=2.82, p=0.002) (Figure 1), but they had higher metabolism in small regions within the left dorsolateral prefrontal cortex (Talairach coordinates: x=–50, y=30, z=30; z score=3.52, p<0.001) and the right dorsolateral prefrontal cortex (Talairach coordinates: x=44, y=26, z=26; z score=2.72, p=0.003) (Figure 2).
To further quantify the difference in cerebral glucose metabolism between the compulsive hoarders and the comparison subjects found in the SPM analysis, we drew the posterior cingulate gyrus on the MRI of each subject and compared normalized glucose metabolic ratios for left and right posterior cingulate in the two groups with a MANOVA after covarying for age, gender, scanner type, and Hamilton depression scale score. Metabolism in the right posterior cingulate was significantly lower in the compulsive hoarders than in the comparison subjects (estimated marginal data: mean=1.30, SE=0.03, versus mean=1.41, SE=0.02; F=5.23, df=2, 21, p=0.03).
In relation to the comparison subjects, the nonhoarding OCD patients had significantly higher glucose metabolism in the left thalamus (Talairach coordinates: x=–16, y=–20, z=14; z score=3.85, p<0.001), the right thalamus (Talairach coordinates: x=12, y=–18, z=4; z score=2.97, p=0.001), the left caudate (Talairach coordinates: x=–6, y=8, z=4; z score=2.58, p=0.005), and the anterior midbrain (Talairach coordinates: x=4, y=–16, z=–18; z score=2.98, p=0.001) (Figure 3). The nonhoarding OCD patients had significantly lower metabolism than the comparison subjects in the right anterior temporal pole (Talairach coordinates: x=36, y=14, z=–26; z score=2.81, p=0.002), the left frontotemporal operculum (Talairach coordinates: x=–58, y=10, z=10; z score=2.77, p=0.003), and the left anterior temporal cortex (Talairach coordinates: x=–44, y=16, z=–22; z score=2.77, p=0.003).
Compared to nonhoarding OCD patients, compulsive hoarders had significantly lower glucose metabolism in the bilateral dorsal anterior cingulate gyrus (Talairach coordinates: x=2, y=22, z=20; z score=3.85, p<0.001) (Figure 4). Across all 45 OCD patients, hoarding severity was negatively correlated with glucose metabolism in this same area, extending into the corpus callosum (Talairach coordinates: x=0, y=20, z=14; z score=3.52, p<0.001).
The compulsive hoarders had a higher metabolism in a small locus in the right sensory motor cortex (Talairach coordinates: x=46, y=–24, z=26; z score=4.02, p<0.001) than the nonhoarding OCD patients, and hoarding severity was positively correlated with metabolism in that same region (Talairach coordinates: x=48, y=–24, z=26; z score=3.41, p<0.001) (Figure 5).
Conclusions
To our knowledge, this is the first study to investigate the functional neuroanatomy of obsessive-compulsive hoarding. The main finding of this study was that the patients with compulsive hoarding syndrome had a different pattern of cerebral glucose metabolism than both the nonhoarding OCD patients and the normal comparison subjects. Although the nonhoarding OCD patients showed the expected elevations in regional metabolism in the caudate and thalamus in relation to the comparison subjects, the compulsive hoarders did not. Instead, the hoarders showed a unique pattern of lower metabolism in the posterior cingulate cortex and the occipital cortex (cuneus) in relation to the comparison subjects. The hoarders and the nonhoarding OCD patients also differed from each other, with the hoarders having significantly lower metabolism in the dorsal anterior cingulate gyrus and the thalamus than the nonhoarding OCD patients. Our findings suggest that compulsive hoarding syndrome may be a neurobiologically distinct variant of OCD.
The symptoms of compulsive hoarding syndrome may be mediated by diminished activity in several parts of the cingulate cortex. Across all OCD patients studied, hoarding severity was significantly correlated with lower activity in the dorsal part of the anterior cingulate gyrus, its cognitive subdivision (78). Functions of the anterior cingulate cortex include motivation, executive control, focused attention, assigning emotional valence to stimuli, monitoring response to conflict, emotional self-control, problem solving, detecting errors, and selecting responses (78, 79). The anterior cingulate also plays a key role in decision making, especially in choosing between multiple conflicting options (80, 81). Compulsive hoarders had significantly lower activity in the right posterior cingulate cortex than the comparison subjects. The posterior cingulate cortex is involved in the monitoring of visual events, spatial orientation, episodic memory (82), and the processing of emotional stimuli (83). It modulates activity in several brain regions involved in the putative functional neurocircuitry of OCD, including the orbitofrontal cortex, the anterior cingulate cortex, and the striatum (84). Thus, lower activity in both the anterior and posterior parts of the cingulate gyrus may mediate the remarkable difficulty in making decisions, attentional problems, and other cognitive deficits seen in compulsive hoarders.
Compulsive hoarders were found to have lower glucose metabolism in brain regions specifically associated with response to treatment in previous studies. Lower pretreatment activity of the anterior cingulate gyrus has been strongly associated with poor response to antidepressant treatment (85–88), while lower pretreatment activity in the posterior cingulate gyrus has been found to correlate with worse response to fluvoxamine (59) and cingulotomy (84) in patients with OCD. Thus, our finding of low cingulate gyrus activity in patients with the compulsive hoarding syndrome is quite consistent with its poor response to standard treatments for OCD. Lower activity in the anterior and posterior cingulate cortex may not only mediate the symptoms of the compulsive hoarding syndrome but also its poor response to treatment.
The findings of low metabolism in the occipital cortex in compulsive hoarders was consistent with the results of prior imaging studies of OCD patients that found decreased metabolism (36, 89) and grey matter density (90) in this area. Abnormalities in parietal-occipital regions may be related to deficits in visuospatial processing and visual memory seen in some OCD patients (91, 92). Our present findings suggest that these abnormalities may be preferentially found in OCD patients with compulsive hoarding syndrome.
This preliminary study had several limitations that reduced its power and generalizability. Because the original study was designed to compare OCD to major depressive disorder and normal comparison subjects—not to examine OCD symptom factors—the analysis of hoarders and nonhoarders was a post hoc exploratory analysis. Hoarders and nonhoarders were classified retrospectively. No standardized or validated measurements of hoarding severity were used. Compulsive hoarders were significantly older than nonhoarders and comparison subjects, so all analyses had to covary for age. In addition, the use of two different PET scanners also required analyses to covary for scanner type and could have caused artifactual results. The addition of multiple covariates may have decreased the statistical power for finding significant differences among groups. Our results will require replication in a new group of compulsive hoarders and age- and sex-matched groups of normal comparison subjects and nonhoarding OCD patients who are defined prospectively.
However, this study also had several strengths that afford confidence in its findings. Both MRI-based localization of regions of interest and whole-brain SPM analyses were used to identify differences of regional brain metabolism between groups and gave similar results. To our knowledge, the overall number of 45 patients with OCD is the largest group of OCD patients ever studied with any functional imaging modality. All subjects were free of psychotropic medications for at least 4 weeks (and at least 5 weeks for fluoxetine), reducing the risk of medication effects on baseline cerebral metabolism. Compulsive hoarders and nonhoarders were similar in terms of symptom severity and prevalence of comorbid major depression. Because about 35% of our OCD patients (25% of the compulsive hoarders and 39% of the nonhoarding OCD patients) had comorbid depression, which exerts a strong influence on baseline cerebral metabolism (40), all analyses covaried for depression severity, as measured by Hamilton depression scale score. Depression severity did not differ between hoarders and nonhoarders in this study, so the differences in thalamic and cingulate metabolism between these groups cannot be accounted for simply by depression.
In conclusion, the findings of this study add to the growing evidence of neurobiological heterogeneity within the diagnosis of OCD. Neurobiological differences may underlie differences in phenomenology, comorbidity, and treatment response between the different symptom factors. In addition to the observed differences in cerebral glucose metabolism, our results raise the question of whether compulsive hoarders also have structural brain abnormalities and neurocognitive deficits that differ from those seen in nonhoarding OCD patients. Identifying neurobiological differences between OCD subtypes may allow for the prediction of differential treatment response and the development of more syndrome-specific treatments.
![]() |
![]() |
Presented in part at the 41st annual meeting of the American College of Neuropsychopharmacology, San Juan, Puerto Rico, Dec. 8–12, 2002, and the 14th annual meeting of the American Neuropsychiatric Association, Honolulu, Hawaii, Feb. 1–4, 2003. From UCLA Neuropsychiatric Institute, David Geffen School of Medicine at UCLA. Address reprint requests to Dr. Saxena, UCLA Department of Psychiatry and Biobehavioral Sciences, 300 UCLA Medical Plaza, Rm. 2229, Los Angeles, CA 90095; [email protected] (e-mail). Supported by an NIMH Career Development Award (MH-01694) to Dr. Saxena, a grant from the Obsessive-Compulsive Foundation to Dr. Saxena, an NIMH grant (MH-53565) to Drs. Baxter and Saxena, grants from the National Alliance for Research in Schizophrenia and Depression to Drs. Brody and Baxter, a Veterans Affairs Type I Merit Review Award to Dr. Brody, a grant from the Tobacco-Related Disease Research Program to Dr. Brody, a grant from the Department of Energy (DE FCE3-87ER 60615) to Dr. Baxter, and donations from Mr. and Mrs. Brian Harvey. The authors thank Mark A. Mandelkern, M.D., Ph.D., and Lynn A. Fairbanks, Ph.D., for assistance with statistical analyses.
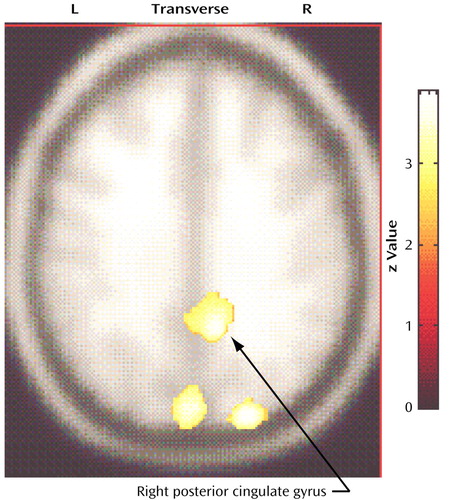
Figure 1. Brain Regions With Significantly Lower Glucose Metabolism in 12 Patients With Compulsive Hoarding Syndrome Than in 17 Normal Comparison Subjectsa
aIn relation to comparison subjects, compulsive hoarders had significantly lower cerebral glucose metabolism in the right posterior cingulate gyrus (Talairach coordinates: x=10, y=–50, z=36; z score=2.71, p=0.003) and the bilateral cuneus (Talairach coordinates: x=–2, y=–84, z=30; z score=2.82, p=0.002).
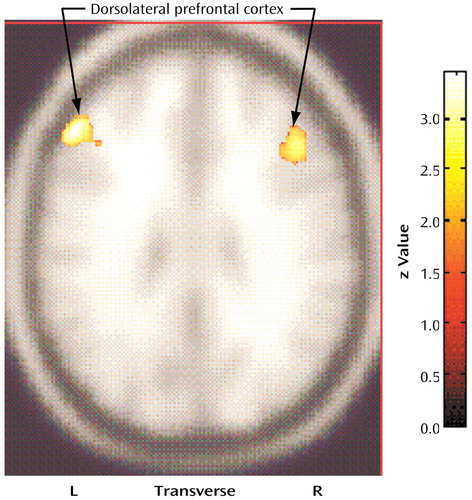
Figure 2. Brain Regions With Significantly Higher Glucose Metabolism in 12 Patients With Compulsive Hoarding Syndrome Than in 17 Normal Comparison Subjectsa
aIn relation to comparison subjects, compulsive hoarders had significantly higher metabolism in the left dorsolateral prefrontal cortex (Talairach coordinates: x=–50, y=30, z=30; z score=3.52, p<0.001) and the right dorsolateral prefrontal cortex (Talairach coordinates: x=44, y=26, z=26; z score=2.72, p=0.003).
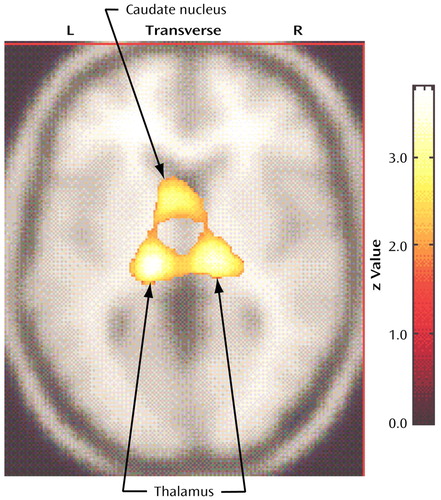
Figure 3. Brain Regions With Significantly Higher Glucose Metabolism in 33 Nonhoarding Patients With Obsessive-Compulsive Disorder (OCD) Than in 17 Normal Comparison Subjectsa
aIn relation to comparison subjects, nonhoarding OCD patients had significantly higher glucose metabolism in the left thalamus (Talairach coordinates: x=–16, y=–20, z=14; z score=3.85, p<0.001, within a cluster extending to include the left caudate nucleus), the right thalamus (Talairach coordinates: x=12, y=–18, z=4; z score=2.97, p=0.001), and the anterior midbrain (Talairach coordinates: x=4, y=–16, z=–18; z score=2.98, p=0.001).
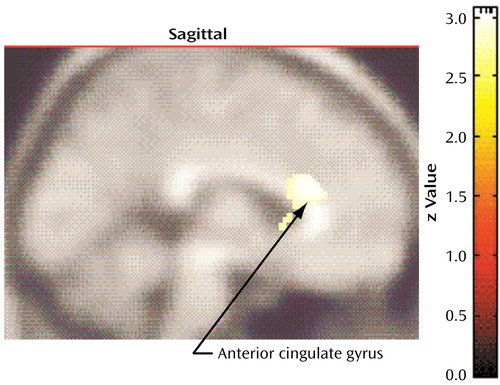
Figure 4. Brain Regions With Significantly Lower Glucose Metabolism in 12 Patients With Compulsive Hoarding Syndrome Than in 33 Nonhoarding Obsessive-Compulsive Disorder (OCD) Patientsa
aIn relation to nonhoarding OCD patients, compulsive hoarders had significantly lower regional cerebral glucose metabolism in the dorsal anterior cingulate gyrus (Talairach coordinates: x=2, y=22, z=20; z score=3.85, p<0.001).
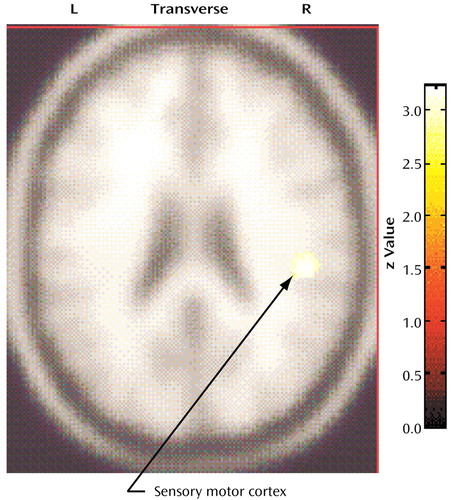
Figure 5. Brain Regions With Significantly Higher Glucose Metabolism in 12 Patients With Compulsive Hoarding Syndrome Than in 33 Nonhoarding Obsessive-Compulsive Disorder (OCD) Patientsa
aCompulsive hoarders had significantly higher metabolism in a small locus in the right sensory motor cortex (Talairach coordinates: x=46, y=–24, z=26; z score=4.02, p<0.001) than nonhoarding OCD patients.
1. Leckman JF, Grice DE, Boardman J, Zhang H, Vitale A, Bondi C, Alsobrook J, Peterson BS, Cohen DJ, Rasmussen SA, Goodman WK, McDougle CJ, Pauls DL: Symptoms of obsessive-compulsive disorder. Am J Psychiatry 1997; 154:911–917Link, Google Scholar
2. Summerfeldt LJ, Richter MA, Antony MM, Swinson RP: Symptom structure in obsessive-compulsive disorder: a confirmatory factor-analytic study. Behav Res Therapy 1999; 37:297–311Crossref, Medline, Google Scholar
3. Cavallini MC, Di Bella D, Siliprandi F, Malchiodi F, Bellodi L: Exploratory factor analysis of obsessive-compulsive patients and association with 5-HTTLPR polymorphism. Am J Med Genet 2002; 114:347–353Crossref, Medline, Google Scholar
4. Mataix-Cols D, Rauch SL, Baer L, Eisen JL, Shera DM, Goodman WK, Rasmussen SA, Jenike MA: Symptom stability in adult obsessive-compulsive disorder: data from a naturalistic two-year follow-up study. Am J Psychiatry 2002; 159:263–268Link, Google Scholar
5. Leckman JF, Pauls DL, Zhang H, Rosario-Campos MC, Katsovich L, Kidd KK, Paktiss AJ, Alsobrook JP, Robertson MM, McMahon WM, Walkup JT, van de Wetering BJM, King RA, Cohen DJ, and the Tourette Syndrome Association International Consortium for Genetics: Obsessive-compulsive symptom dimensions in affected sibling pairs diagnosed with Gilles de la Tourette syndrome. Am J Med Genet 2003; 116B:60–68Google Scholar
6. Samuels J, Bienvenu OJ, Riddle MA, Cullen BAM, Grados MA, Liang KY, Hoehn-Saric R, Nestadt G: Hoarding in obsessive-compulsive disorder: results from a case-control study. Behav Res Therapy 2002; 40:517–528Crossref, Medline, Google Scholar
7. Black DW, Monahan P, Gable J, Blum N, Clancy G, Baker P: Hoarding and treatment response in non-depressed subjects with obsessive-compulsive disorder. J Clin Psychiatry 1998; 59:420–425Crossref, Medline, Google Scholar
8. Winsberg ME, Cassic KS, Koran LM: Hoarding in obsessive-compulsive disorder: a report of 20 cases. J Clin Psychiatry 1999; 60:591–597Crossref, Medline, Google Scholar
9. Mataix-Cols D, Rauch SL, Manzo PA, Jenike MA, Baer L: Use of factor-analyzed symptom dimensions to predict outcome with serotonin reuptake inhibitors and placebo in the treatment of obsessive-compulsive disorder. Am J Psychiatry 1999; 156:1409–1416Abstract, Google Scholar
10. Basoglu M, Lax T, Kasviks Y, Marks M: Predictors of improvement in obsessive-compulsive disorder. J Anxiety Disorders 1988; 2:299–317Crossref, Google Scholar
11. Mataix-Cols D, Marks IM, Greist JH, Kobak KA, Baer L: Obsessive-compulsive symptom dimensions as predictors of compliance with and response to behaviour therapy: results from a controlled trial. Psychother Psychosom 2002; 71:255–262Crossref, Medline, Google Scholar
12. Frost RO, Gross RC: The hoarding of possessions. Behav Res Ther 1993; 31:367–381Crossref, Medline, Google Scholar
13. Stein DJ, Seedat S, Potocnik F: Hoarding: a review. Isr J Psychiatry Relat Sci 1999; 36:35–46Medline, Google Scholar
14. Frost RO, Krause MS, Steketee G: Hoarding and obsessive-compulsive symptoms. Behav Modif 1996; 20:116–132Crossref, Medline, Google Scholar
15. Rasmussen S, Eisen JL: The epidemiology and clinical features of obsessive-compulsive disorder. Psychiatric Clin North Am 1992; 15:743–758Crossref, Medline, Google Scholar
16. Hanna GL: Demographic and clinical features of obsessive-compulsive disorder in children and adolescents. J Am Acad Child Adolesc Psychiatry 1995; 34:19–27Crossref, Medline, Google Scholar
17. Frost RO, Steketee G, Williams LF, Warren R: Mood, personality disorder symptoms, and disability in obsessive compulsive hoarders: a comparison with clinical and non-clinical controls. Behav Res Ther 2000; 38:1071–1081Crossref, Medline, Google Scholar
18. Saxena S, Maidment KM, Vapnik T, Golden G, Rishwain T, Rosen RM, Tarlow G, Bystritsky A: Obsessive-compulsive hoarding: symptom severity and response to multi-modal treatment. J Clin Psychiatry 2002; 63:21–27Crossref, Medline, Google Scholar
19. Steketee G, Frost RO, Kim H-J: Hoarding by elderly people. Health Social Work 2001; 26:176–184Crossref, Medline, Google Scholar
20. Greenberg D: Compulsive hoarding. Am J Psychother 1987; 41:409–416Crossref, Medline, Google Scholar
21. Zhang H, Leckman JF, Pauls DL, Tsai C-P, Kidd KK, Rosario Campos M, and the Tourette Syndrome Association International Consortium for Genetics: Genomewide scan of hoarding in sib pairs in which both sibs have Gilles de la Tourette syndrome. Am J Hum Genet 2002; 70:896–904Crossref, Medline, Google Scholar
22. Mogenson GJ, Wu M: Disruption of food hoarding by injections of procaine into mediodorsal thalamus, GABA into subpallidal regions, and haloperidol into accumbens. Brain Res Bull 1988; 20:247–251Crossref, Medline, Google Scholar
23. Saxena S, Brody AL, Schwartz JM, Baxter LR: Neuroimaging and frontal-subcortical circuitry in obsessive-compulsive disorder. Br J Psychiatry 1998; 173(suppl 35):26–38Google Scholar
24. de Brabander JM, de Bruin JP, van Eden CG: Comparison of the effects of neonatal and adult medial prefrontal cortex lesions on food hoarding and spatial delayed alternation. Behav Brain Res 1991; 42:67–75Crossref, Medline, Google Scholar
25. Blundell JE, Herberg LJ: Effectiveness of lateral hypothalamic stimulation, arousal, and food deprivation in the initiation of food hoarding behavior in naïve rats. Physiol Behav 1973; 10:763–767Crossref, Medline, Google Scholar
26. Kolb B: Studies on the caudate-putamen and dorsomedial thalamic nucleus of the rat: implications for mammalian frontal-lobe functions. Physiol Behav 1977; 18:237–244Crossref, Medline, Google Scholar
27. Blundell JE, Strupp BJ, Latham CJ: Pharmacological manipulation of hoarding: further analysis of amphetamine isomers and pimozide. Physiol Psychol 1977; 5:462–468Crossref, Google Scholar
28. Kalsbeek A, De Bruin JPC, Feenstra MGP, Matthijssen MA, Uylings HB: Neonatal thermal lesions of the mesolimbocortical dopaminergic projection decrease food-hoarding behavior. Brain Res 1988; 475:80–90Crossref, Medline, Google Scholar
29. Kelley AE, Stinus L: Disappearance of hoarding behavior after 6-hydroxydopamine lesions of the mesolimbic dopamine neurons and its reinstatement with l-dopa. Behavioral Neurosci 1985; 99:531–545Crossref, Medline, Google Scholar
30. Fantino M, Boucher H, Faion F, Mathiot P: Dexfenfluramine and body weight regulations: experimental study with hoarding behavior. Clin Neuropharmacol 1988; 11(suppl 1):S97-S104Google Scholar
31. Coling JG, Herberg LJ: Effect of ovarian and exogenous hormones on defended body weight, actual body weight, and the paradoxical hoarding of food by female rats. Physiol Behav 1982; 29:687–691Crossref, Medline, Google Scholar
32. MacNamara RK, Whishaw IQ: Blockade of hoarding in rats by diazepam: an analysis of the anxiety and object value hypotheses of hoarding. Psychopharmacology 1990; 101:214–221Crossref, Medline, Google Scholar
33. Kavaliers M, Hirst M: Differential opiate influences on food hoarding and intake in the deer mouse. Life Sciences 1985; 37:2213–2220Crossref, Medline, Google Scholar
34. Baxter LR, Phelps ME, Mazziotta JC, Guze BH, Schwartz JM, Selin CE: Local cerebral glucose metabolic rates in obsessive-compulsive disorder— a comparison with rates in unipolar depression and in normal controls. Arch Gen Psychiatry 1987; 44:211–218Crossref, Medline, Google Scholar
35. Baxter LR Jr, Schwartz JM, Mazziotta JC, Phelps ME, Pahl JJ, Guze BH, Fairbanks L: Cerebral glucose metabolic rates in nondepressed patients with obsessive-compulsive disorder. Am J Psychiatry 1988; 145:1560–1563Link, Google Scholar
36. Nordahl TE, Benkelfat C, Semple WE, Gross M, King AC, Cohen RM: Cerebral glucose metabolic rates in obsessive-compulsive disorder. Neuropsychopharm 1989; 2:23–28Crossref, Medline, Google Scholar
37. Swedo S, Schapiro MG, Grady CL, Cheslow DL, Leonard HL, Kumar A, Friedland R, Rapaport SI, Rapaport JL: Cerebral glucose metabolism in childhood onset obsessive-compulsive disorder. Arch Gen Psychiatry 1989; 46:518–523Crossref, Medline, Google Scholar
38. Sawle GV, Hymas NF, Lees AJ, Frackowiak RS: Obsessional slowness: Functional studies with positron emission tomography. Brain 1991; 114:191–202Google Scholar
39. Perani D, Colombo C, Bressi S, Bonfanti A, Grassi F, Scarone S, Bellodi L, Smeraldi E, Fazio F: [18F]FDG PET study in obsessive-compulsive disorder: a clinical/metabolic correlation study after treatment. Br J Psychiatry 1995; 166:244–250Crossref, Medline, Google Scholar
40. Saxena S, Brody AL, Ho ML, Alborzian S, Ho MK, Maidment KM, Huang SC, Wu HM, Au SC, Baxter LR: Cerebral metabolism in major depression and obsessive-compulsive disorder occurring separately and concurrently. Biol Psychiatry 2001; 50:170–181Crossref, Google Scholar
41. Benkelfat C, Nordahl TE, Semple WE, King AC, Murphy DL, Cohen RM: Local cerebral glucose metabolic rates in obsessive-compulsive disorder: patients treated with clomipramine. Arch Gen Psychiatry 1990; 47:840–848Crossref, Medline, Google Scholar
42. Baxter LR, Schwartz JM, Bergman KS, Szuba MP, Guze BH, Mazziota JC, Alazraki A, Selin CE, Ferng HK, Munford P, Phelps ME: Caudate glucose metabolic rate changes with both drug and behavior therapy for obsessive-compulsive disorder. Arch Gen Psychiatry 1992; 49:681–689Crossref, Medline, Google Scholar
43. Swedo SE, Pietrini P, Leonard HL, Schapiro MB, Rettew DC, Goldberger EL, Rapaport SI, Rapaport JL, Grady CL: Cerebral glucose metabolism in childhood-onset obsessive-compulsive disorder: revisualization during pharmacotherapy. Arch Gen Psychiatry 1992; 49:690–694Crossref, Medline, Google Scholar
44. Schwartz JM, Stoessel PW, Baxter LR, Martin KM, Phelps ME: Systematic changes in cerebral glucose metabolic rate after successful behavior modification treatment of obsessive-compulsive disorder. Arch Gen Psychiatry 1996; 53:109–113Crossref, Medline, Google Scholar
45. Saxena S, Brody AL, Ho ML, Alborzian S, Maidment KM, Zohrabi N, Ho MK, Huang SC, Wu HM, Baxter LR: Differential cerebral metabolic changes with paroxetine treatment of obsessive-compulsive disorder versus major depression. Arch Gen Psychiatry 2002; 59:250–261Crossref, Medline, Google Scholar
46. Hansen ES, Hasselbach S, Law I, Bolwig TG: The caudate nucleus in obsessive-compulsive disorder: reduced metabolism following treatment with paroxetine: a PET study. Int J Neuropsychopharmacology 2002; 5:1–10Crossref, Medline, Google Scholar
47. McGuire PK, Bench CJ, Frith CD, Marks IM, Frackowiak RS, Dolan RJ: Functional anatomy of obsessive-compulsive phenomena. Br J Psychiatry 1994; 164:459–468Crossref, Medline, Google Scholar
48. Rauch SL, Jenike MA, Alpert NM, Baer L, Breiter HC, Savage CR, Fischman AJ: Regional cerebral blood flow measured during symptom provocation in obsessive-compulsive disorder using oxygen 15-labeled carbon dioxide and positron emission tomography. Arch Gen Psychiatry 1994; 51:62–70Crossref, Medline, Google Scholar
49. Breiter HC, Rauch SL, Kwong KK, Baker JR, Weiskoff RM, Kennedy DN, Kendrick AD, Davis TL, Jiang A, Cohen MS, Stern CE, Belliveau JW, Baer L, O’Sullivan RM, Savage CR, Jenike MA, Rosen BR: Functional magnetic resonance imaging of symptom provocation in obsessive-compulsive disorder. Arch Gen Psychiatry 1996; 53:595–606Crossref, Medline, Google Scholar
50. Cottraux J, Gerard D, Cinotti L, Froment J-C, Deiber M-P, Le Bars D, Galy G, Millet P, Labbe C, Lavenne F, Bouvard M, Mauguiere F: A controlled positron emission tomography study of obsessive and neutral auditory stimulation in obsessive-compulsive disorder with checking rituals. Psychiatry Res 1996; 60:101–112Crossref, Medline, Google Scholar
51. Alexander GE, DeLong MR, Strick PL: Parallel organization of functionally segregated circuits linking basal ganglia and cortex. Ann Review Neurosci 1986; 9:357–381Crossref, Medline, Google Scholar
52. Rapaport JL, Wise SP: Obsessive-compulsive disorder: is it a basal ganglia dysfunction? Psychopharm Bull 1988; 1:27–36Google Scholar
53. Insel TR: Obsessive-compulsive disorder: a neuroethological perspective. Psychopharm Bull 1988; 24:365–369Medline, Google Scholar
54. Modell JG, Mountz JM, Curtis GC, Greden JF: Neurophysiologic dysfunction in basal ganglia/limbic striatal and thalamocortical circuits as a pathogenetic mechanism of obsessive-compulsive disorder. J Neuropsychiatry Clin Neurosci 1989; 1:27–36Crossref, Medline, Google Scholar
55. Baxter LR, Saxena S, Brody AL, Ackermann RF, Colgan ME, Schwartz JM, Allen-Martinez Z, Fuster JM, Phelps ME: Brain mediation of obsessive-compulsive disorder symptoms: evidence from functional brain imaging studies in the human and non-human primate. Sem Clin Neuropsychiatry 1996; 1:32–46Medline, Google Scholar
56. Saxena S, Bota RG, Brody AL: Brain-behavior relationships in obsessive-compulsive disorder. Sem Clin Neuropsychiatry 2001; 6:82–101Crossref, Medline, Google Scholar
57. Rauch SL, Dougherty DD, Shin LM, Alpert NM, Manzo P, Leahy L, Fischman AJ, Jenike MA, Baer L: Neural correlates of factor-analyzed OCD symptom dimensions: a PET study. CNS Spectrums 1998; 3:37–43Crossref, Google Scholar
58. Rauch SL, Shin LM, Dougherty DD, Alpert NM, Fischman AJ, Jenike MA: Predictors of fluvoxamine response in contamination-related obsessive-compulsive disorder: a PET symptom provocation study. Neuropsychopharmacology 2002; 27:782–791Crossref, Medline, Google Scholar
59. Mataix-Cols D, Cullen S, Lange K, Zelaya F, Andrew C, Amaro E, Brammer MJ, Williams SCR, Speckens A, Phillips ML: Neural correlates of anxiety associated with obsessive-compulsive symptom dimensions in normal volunteers. Biol Psychiatry 2003; 53:482–493Crossref, Medline, Google Scholar
60. Spitzer RL, Endicott J: Schedule for Affective Disorders and Schizophrenia (SADS), 3rd ed. New York, New York State Psychiatric Institute, Biometrics Research, 1978Google Scholar
61. Goodman WK, Price LH, Rasmussen SA, Mazure C, Fleischmann RL, Hill CL, Heninger GR, Charney DS: The Yale-Brown Obsessive Compulsive Scale, I: development, use, and reliability. Arch Gen Psychiatry 1989; 46:1006–1011Crossref, Medline, Google Scholar
62. Hamilton M: Diagnosis and rating scale for depression. Br J Psychiatry 1960; 3:76–79Google Scholar
63. Hamilton M: A rating scale for anxiety. J Neurol Neurosurg Psychiatry 1960; 23:56–62Crossref, Medline, Google Scholar
64. Endicott J, Spitzer RL, Fleiss JL, Cohen J: The Global Assessment Scale: a procedure for measuring overall severity of psychiatric disturbance. Arch Gen Psychiatry 1976; 41:586–601Google Scholar
65. Zohar J, Judge R (OCD Paroxetine Study Investigators): Paroxetine versus clomipramine in the treatment of obsessive-compulsive disorder. Br J Psychiatry 1996; 169:468–474Crossref, Medline, Google Scholar
66. Benkelfat C, Nordahl TE, Semple WE, King AC, Murphy DL, Cohen RM: Local cerebral glucose metabolic rates in obsessive-compulsive disorder: patients treated with clomipramine. Arch Gen Psychiatry 1990; 47:840–848Crossref, Medline, Google Scholar
67. Wood A, Tollefson GD, Birkett M: Pharmacotherapy of obsessive-compulsive disorder—experience with fluoxetine. Int Clin Psychopharmacol 1993; 8:301–306Crossref, Medline, Google Scholar
68. Friston K, Frith C, Liddle P, Frackowiak R: Comparing functional (PET) images: the assessment of significant change. J Cereb Blood Flow Metab 1991; 11:690–699Crossref, Medline, Google Scholar
69. Friston KJ, Worsley KJ, Frackowiak RSJ, Mazziotta JC, Evans AC: Assessing the significance of focal activations using their spatial extent. Human Brain Mapping 1994; 1:214–220Google Scholar
70. Steinmetz H, Seitz RJ: Functional anatomy of language processing: neuroimaging and the problem of individual variability. Neuropsychologia 1991; 29:1149–1161Crossref, Medline, Google Scholar
71. Lin KP, Huang S-C, Baxter LR, Phelps ME: A general technique for inter-study registration of multi-function and multimodality images. J Cereb Blood Flow Metab 1993; 9:96–103Google Scholar
72. Damasio H: Human Brain Anatomy in Computerized Images. New York, Oxford University Press, 1995Google Scholar
73. Mai JK, Assheuer J, Paxton DA: Atlas of the Human Brain. San Diego, Academic Press, Harcourt Brace and Company, 1997Google Scholar
74. Rajkowska G, Goldman-Rakic PS: Cytoarchitectonic definition of prefrontal areas in the normal human cortex, II: variability in locations of areas 9 and 46 and relationship to the Talairach coordinate system. Cereb Cortex 1995; 5:323–337Crossref, Medline, Google Scholar
75. Friston KJ, Holmes AP, Worsley KJ, Poline JP, Frith CD, Frackowiak RSJ: Statistical parametric maps in functional imaging: a general linear approach. Human Brain Mapp 1995; 2:189–210Crossref, Google Scholar
76. Talairach J, Tournoux P: Co-Planar Stereotaxic Atlas of the Human Brain: Three-Dimensional Proportional System. New York, Thieme Medical, 1988Google Scholar
77. Drevets WC: Functional neuroimaging studies of depression: the anatomy of melancholia. Ann Rev Med 1998; 49:341–361Crossref, Medline, Google Scholar
78. Devinsky O, Morrell MJ, Vogt BA: Contributions of anterior cingulate cortex to behavior. Brain 1995; 118:279–306Crossref, Medline, Google Scholar
79. Awh E, Gehring WJ: The anterior cingulate lends a hand in response selection. Nature Neurosci 1999; 2:853–854Crossref, Medline, Google Scholar
80. Carter CS, Braver TS, Barch DM, Botvinik MM, Noll D, Cohen J: Anterior cingulate cortex, error detection, and online monitoring of performance. Science 1998; 280:747–749Crossref, Medline, Google Scholar
81. Krawczyk DC: Contributions of the prefrontal cortex to the neural basis of decision making. Neurosci Biobehav Rev 2002; 26:631–664Crossref, Medline, Google Scholar
82. Vogt BA, Finch DM, Olson CR: Functional heterogeneity in the cingulate cortex: the anterior executive and posterior evaluative regions. Cereb Cortex 1992; 2:435–443Medline, Google Scholar
83. Maddock RJ: The retrosplenial cortex and emotion: new insight from functional neuroimaging of the human brain. Trends Neurosci 1999; 22:310–316Crossref, Medline, Google Scholar
84. Rauch SL, Dougherty DD, Cosgrove GR, Cassem EH, Alpert NM, Price BH, Nierenberg AA, Mayberg HS, Baer L, Jenike MA, Fischman AJ: Cerebral metabolic correlates as potential predictors of response to anterior cingulotomy for obsessive-compulsive disorder. Biol Psychiatry 2001; 50:659–667Crossref, Medline, Google Scholar
85. Mayberg HS, Brannan SK, Mahurin RK, Jerabek PA, Brickman JS, Tekell JL, Silva JA, McGinnis S, Glass TG, Martin CC, Fox PT: Cingulate function in depression: a potential predictor of treatment response. Neuroreport 1997; 8:1057–1061Crossref, Medline, Google Scholar
86. Wu J, Buchsbaum MS, Gillin JC, Tang C, Cadwell S, Wiegand M, Najafi A, Klein E, Hazen K, Bunney WE Jr, Fallon JH, Keator D: Prediction of antidepressant effects of sleep deprivation by metabolic rates in the ventral anterior cingulate and medial prefrontal cortex. Am J Psychiatry 1999; 156:1149–1158; correction, 1999; 156:1666Google Scholar
87. Pizzagalli D, Pascual-Marqui RD, Nitschke JB, Oakes TR, Larson CL, Abercrombie HC, Schaefer SM, Koger JV, Benca RM, Davidson RJ: Anterior cingulate activity as a predictor of degree of treatment response in major depression: evidence from brain electrical tomography analysis. Am J Psychiatry 2001; 158:405–415Link, Google Scholar
88. Saxena S, Brody AL, Ho ML, Zohrabi N, Maidment KM, Baxter LR Jr: Differential brain metabolic predictors of response to paroxetine in obsessive-compulsive disorder versus major depression. Am J Psychiatry 2003; 160:522–532Link, Google Scholar
89. Kwon JS, Kim J-J, Lee DW, Lee JS, Lee DS, Kim M-S, Lyoo IK, Cho MJ, Lee MC: Neural correlates of clinical symptoms and cognitive dysfunctions in obsessive-compulsive disorder. Psychiatry Res Neuroimaging 2003; 122:37–47Crossref, Medline, Google Scholar
90. Kim J-J, Lee MC, Kim J, Kim IY, Kim SI, Han MH, Chang K-H, Kwon JS: Grey matter abnormalities in obsessive-compulsive disorder: SPM of segmented magnetic resonance images. Br J Psychiatry 2001; 179:330–334Crossref, Medline, Google Scholar
91. Purcell R, Maruff P, Kyrios M, Pantelis C: Cognitive deficits in obsessive-compulsive disorder in tests of fronto-striatal function. Biol Psychiatry 1998; 43:348–357Crossref, Medline, Google Scholar
92. Savage CR, Baer L, Keuthen NJ, Brown HD, Rauch SL, Jenike MA: Organizational strategies mediate nonverbal memory impairment in obsessive-compulsive disorder. Biol Psychiatry 1999; 45:905–916Crossref, Medline, Google Scholar