Deficits in Hippocampal and Anterior Cingulate Functioning During Verbal Declarative Memory Encoding in Midlife Major Depression
Abstract
OBJECTIVE: Prior studies showed that subjects with major depression have deficits in hippocampal-based verbal declarative memory (e.g., recall of a paragraph) and in hippocampal and prefrontal cortical functioning and structure. The purpose of the present study was to assess hippocampal and prefrontal functioning during performance of a verbal declarative memory task in subjects with midlife major depression. METHOD: Subjects with midlife major depression (N=18) and healthy subjects (N=9) underwent positron emission tomography imaging during a control task and verbal encoding of a paragraph. RESULTS: During the verbal memory encoding task the comparison subjects, but not the subjects with depression, activated the right hippocampus and prefrontal cortex (anterior cingulate), as well as the cuneus and cerebellum. CONCLUSIONS: These results are consistent with a failure of hippocampal and anterior cingulate activation in depression, and they support the hypothesis of deficits in hippocampal and anterior cingulate functioning in depression.
Major depression is an important public health problem that affects about 16% of the population at some time in their lives (1). Depression is therefore the most common disorder in this country that is likely to be associated with major morbidity as well as loss of economic productivity (2). The risk of recurrence of depression increases with repeated episodes. For instance, the risk of having another depressive episode after a single episode is about 50%, whereas with a past history of two or more episodes, the risk of having a recurrence of depression is greater than 90%. One possible explanation for this pattern of recurrence is that changes in the brain that are caused by depressive episodes may lead to an increased risk of recurrence. However, in spite of the importance of depression for our society, little is known about changes in the brain that may lead to depressive episodes and promote the recurrence of depression.
Animal models of depression, such as exposure to chronic uncontrollable stress, demonstrate long-term alterations in neurophysiology (3–6). Glucocorticoids such as cortisol, which are released during stress, were associated in some studies with damage to neurons (7–10) and inhibition of neurogenesis (11, 12) in the hippocampus, a brain area involved in learning and memory, with associated deficits in hippocampal-based memory functioning (13, 14). Hypercortisolemia is a consistent finding in a subgroup of subjects with severe depression (15–21) that may be related in part to hippocampal dysfunction (22, 23). Subjects with depression also show deficits in performance on hippocampal-based verbal declarative memory tasks, including paragraph delayed recall and word list learning (24–29), that may be mediated by the effects of cortisol on the brain (30, 31). The hippocampus has important connections with the prefrontal cortex, which has also been implicated in depression (32, 33). The areas of the prefrontal cortex that have been implicated in depression include the dorsolateral prefrontal cortex (involved in working memory) and medial prefrontal cortex (including the anterior cingulate and orbitofrontal cortex).
Imaging studies have implicated the hippocampus and prefrontal cortex in the mediation of depressive symptoms (34–36). Magnetic resonance imaging studies of subjects with depression showed smaller hippocampal volumes and other abnormalities in hippocampal structure in some studies (37–45), but not others (46, 47), and smaller subgenual (anterior cingulate) cortical (48) and orbitofrontal cortical (49, 50) volumes. Multiple studies of blood flow and/or metabolism, using positron emission tomography (PET) or single photon emission computed tomography, in subjects with untreated depression have shown low functioning in the left (51–54) and bilateral (55–57) dorsolateral prefrontal cortex and in the medial prefrontal cortex/anterior cingulate/orbitofrontal cortex (48, 57–62). Induction of depressive symptoms with reduction of brain serotonin (32) or norepinephrine (63) levels resulted in decreased metabolism in the dorsolateral prefrontal cortex, orbitofrontal cortex, and thalamus. In summary, the imaging findings in depression to date are consistent with dysfunction of the hippocampus and prefrontal cortex (specifically anterior cingulate) in depression. A gap in the literature has been the use of specific probes, such as memory encoding, of hippocampal and frontal cortical functioning in depression.
The PET studies performed to date in healthy human subjects have not consistently shown activation of the hippocampus during performance of verbal declarative memory tasks. Some studies have shown activation with tasks such as stem completion (64–66), although a larger number of studies have not (67–75). Factors such as the success of retrieval (69, 76–78) and emotional valence (74) have been suggested as factors that could affect hippocampal activation. More consistent hippocampal activations has been shown for encoding (as opposed to retrieval) tasks (79–85). We have found hippocampal activation in normal subjects with a paragraph encoding task (86). The paragraph encoding task involved encoding of complex and integrated information that uses the integration functions hypothesized as a role for the hippocampus (87). This type of cognitive function is also classically impaired in patients with known hippocampal lesions (88). Deficits in paragraph recall have been associated with depression. We therefore used paragraph encoding as the verbal declarative memory task in the current study, whose purpose was to use a verbal declarative memory encoding task as a probe of hippocampal and anterior cingulate functioning in subjects with midlife depression. We hypothesized that subjects with depression would show less activation of the hippocampus and anterior cingulate during the declarative memory task than would healthy comparison subjects.
Method
Subjects
Twenty-seven men and women participated in the study, including nine subjects without a history of major depression and 18 with midlife depression. The subjects included six healthy women and three healthy men and 13 women and five men with major depression. The project was approved by a local human investigation committee. All subjects were recruited through newspaper advertisement. The diagnosis of major depression was established with the Structured Clinical Interview for DSM-IV (SCID) (89). The subjects were also evaluated with the Hamilton Depression Rating Scale, a validated instrument for measurement of depression severity (90). All subjects gave written informed consent for participation, were free of major medical illness on the basis of history and physical examination, laboratory testing, and electrocardiogram, were not actively abusing substances or alcohol (in the past 6 months), and were free of all medications for at least 4 weeks before the study. The subjects did not stop taking medication for the purpose of participating in the study. Subjects with a serious medical or neurological illness, an organic mental disorder or comorbid psychotic disorder or posttraumatic stress disorder (PTSD), a history of childhood trauma as measured with the Early Trauma Inventory (91), a current or past history of alcohol or substance abuse or dependence, retained metal, or a history of head trauma, loss of consciousness, cerebral infectious disease, or dyslexia were excluded. There was no difference in age between the healthy subjects (mean=38 years, SD=2) and the subjects with major depression (mean=43 years, SD=2) (t=1.03, df=25, p=0.31). There was also no difference in the number of years of education between the healthy subjects (mean=16, SD=2) and the subjects with depression (mean=16, SD=2) (t=0.76, df=25, p=0.45). All subjects were right-handed.
All of the depressed subjects had current and lifetime unipolar major depression. The current level of depressive symptoms as measured with the Hamilton depression scale was 33 (SD=9) in the depressed group. Three of the subjects (17%) had depression with melancholia; none had atypical depression. Two (11%) of the 18 depressed subjects fulfilled the criteria for a lifetime history of dysthymia, and one (6%) had a current history of dysthymia based on the SCID. Five (28%) of the 18 had new-onset major depression; the rest (72%) had recurrent major depression. The comparison subjects did not have a history of psychiatric disorder as measured with the SCID.
PET Scanning Methods
Each subject was scanned on a single day in conjunction with verbal memory tasks. The subjects underwent PET scanning with four activations (two control tasks and two memory encoding tasks). Consecutive PET scans were separated by 10 minutes. H2[15O] was prepared on-site in a cyclotron. An intravenous infusion of normal saline was started to permit the bolus injection of H2[15O]. Each subject was scanned with his or her eyes open in a dimly lit room. The subject was placed in the scanner with the head held in a head holder to minimize motion. The head was positioned with the canthomeatal line parallel to the external laser light. After positioning within the camera gantry, a transmission scan of the head was obtained by using an external 67Ga/68Ge rod source. This data were used to correct emission data from attenuation due to overlying bone and soft tissue. The subject received a 30-mCi intravenous bolus of H2[15O] for each of the four scans. Each condition lasted 60 seconds, with the PET scan acquisition beginning at the initiation of the condition and ending after 60 seconds.
The subjects were initially instructed that this was a test of how “the brain processes information” and were not told that it was a test of memory. Before the first two scans they were read a list of 10 pairs of words for one minute and asked to count the number of times they heard a word that contained the letter “D” (a control condition providing poorly encoded words). They were then read a second list of 10 different word pairs for 1 minute and again asked to count the number of times they heard the letter “D.” These words were neutral in content and were all concrete nouns in common usage in the English language. Methods related to the development of these words, including ratings of emotional content, were previously published (74). The subjects then underwent PET imaging for scan 1 while they were asked to remember the poorly encoded words. This was repeated for scan 2 for the second list. Before scan 3, the subjects were told that a paragraph would be read to them during the next scan, and they were asked to remember it and to form an image of the scene in their mind during the scan (deep encoding). The paragraph read to them during scan 3 contained neutral content. Five minutes later the subjects were asked to remember the paragraph, and the accuracy of their recall was scored. This procedure was repeated for scan 4.
Image Analysis
The images were reconstructed and analyzed on a SunSparc Workstation by using statistical parametric mapping (SPM 96). The images for each patient set were realigned to the first scan of the study session. The mean concentration of radioactivity in each scan was calculated as the area-weighted sum of the concentration of each slice and adjusted to a nominal value of 50 ml/minute per 100 g. The data were transformed into a common anatomical space by using the Montreal Neurological Institute template (92) and were smoothed with a three-dimensional Gaussian filter to 16 mm full-width at half maximum. Regional blood flow, with global blood flow as a covariate, was compared in the control and deep encoding conditions in the subjects with depression and the comparison subjects. The interaction between group (depressed versus comparison subjects) and condition (control condition versus deep paragraph encoding) was also examined. Repeated measures of cerebral blood flow at rest have shown variability of 2.3% (SD=8.7%) within subjects (93) and 4.5% from our site (94). Statistical analyses yielded image data sets in which the values assigned to individual voxels corresponded to t statistic values (95, 96). The statistical images were displayed with values of z score units. A threshold z score of 2.58 (p<0.005) was used to define areas of activation within regions included in our hypothesis. The threshold z score corresponds to a p value of <0.001 for a one-tailed t test. Since the current study used an a priori hypothesis, this justifies the use of a one-tailed test. Locations of areas of activation were identified as the distance from the anterior commissure in millimeters, with x, y, and z coordinates, based on a standard stereotaxic atlas (97).
The relationships between behavioral and brain measures were assessed by using Spearman correlations. Analysis of variance was used to assess the difference in behavioral measures and memory performance between subjects with and without depression.
Results
There were no significant differences between the subjects with depression and the comparison subjects in paragraph recall scores for either the first strongly encoded paragraph (mean=24, SD=7, versus mean=28, SD=8; t=1.43, df=24, p=0.17) or the second one (mean=26, SD=9, versus mean=30, SD=6; t=1.05, df=25, p=0.31). There were also no differences in recall of the weakly encoded memory material.
Memory encoding of a paragraph by the healthy subjects resulted in increased blood flow in the hippocampus, anterior cingulate, cerebellum, lingual gyrus, precuneus, cuneus, visual association cortex, motor cortex, and inferior/middle frontal gyrus. Paragraph encoding resulted in a decrease in blood flow in the right middle/inferior frontal gyrus, anteromedial prefrontal cortex, bilateral fusiform gyrus, cerebellum, and right middle temporal gyrus (Table 1). Memory encoding by the subjects with depression resulted in an increase in blood flow in the bilateral precentral gyrus (motor cortex), right middle and superior frontal gyrus, right visual association cortex, and right posterior cingulate (Table 2). Memory encoding resulted in a decrease in blood flow in the depressed subjects in the right caudate, bilateral middle temporal gyrus, cerebellum, right inferior frontal gyrus, right inferior parietal lobule, and left hippocampus. Comparison of the healthy subjects with the depressed subjects showed that the healthy subjects had greater increases in blood flow during memory encoding in the cerebellum, bilateral anterior cingulate, right hippocampus and amygdala, left hippocampal region, right cuneus, left visual association cortex, left middle temporal gyrus, right superior temporal gyrus, and right inferior frontal gyrus (Table 3, Figure 1, Figure 2). The healthy subjects showed greater decreases in blood flow with memory encoding in the right middle and inferior frontal gyrus, cerebellum, and left inferior parietal lobule (Table 3, Figure 3).
There was no correlation between hippocampal activation and several demographic factors, including age and sex, in the depressed or nondepressed subjects. There was no relationship between level of depression as measured by the Hamilton depression scale and hippocampal activation in the depressed subjects. There were also no differences in hippocampal activation between the subjects with new-onset depression and those with recurrent depression.
Discussion
Memory encoding resulted in greater hippocampal and anterior cingulate activation in healthy subjects than in subjects with currently untreated midlife depression. These findings are consistent with the hypothesis of hippocampal and anterior cingulate dysfunction in depression. The use of a verbal memory encoding task as a specific probe of hippocampal and prefrontal functioning provides evidence that converges with findings from a number of structural and functional baseline imaging studies that suggest dysfunction in the hippocampus and prefrontal cortex in depression.
The network of brain regions activated by memory encoding differed between the subjects with depression and the healthy subjects. For example, there was greater activation of the right middle and inferior frontal gyri in the subjects with depression. These subjects may have relied on these areas to a greater degree because of dysfunction in the hippocampus and anterior cingulate. The right inferior frontal gyrus has been hypothesized to be involved in the effort of retrieval, but not necessarily in successful retrieval. It is of interest that the depressed subjects relied more on the right middle frontal gyrus while the nondepressed subjects used the left middle frontal gyrus in encoding. There were no differences in performance of the paragraph recall task, however, between the subjects with and without depression. This suggests that the differences in brain activation were not primarily related to differences in performance.
Memory encoding in the healthy subjects involved recruitment of an extended network of brain regions similar to those shown by prior imaging studies of the neural correlates of memory. Brain regions involved in memory encoding by the healthy subjects in the current study that have been found to be activated in prior studies of the neural correlates of declarative memory include the precuneus (72, 73, 98), cuneus (65, 74), left inferior/middle frontal gyrus (65, 69, 70, 72, 73), and precentral gyrus (70, 73, 74). The inferior/middle frontal gyrus portion of the prefrontal cortex is felt to be involved in verbal memory encoding and/or retrieval (99), as well as verbal processing (100, 101) and working memory (102, 103). In prior PET studies of normal subjects, the anterior cingulate (65, 68–70, 72, 74, 98, 99) has been consistently associated with verbal memory retrieval, while some studies (but not all) showed hippocampal activation (65, 67, 76). The depressed subjects in this study shared with the healthy subjects recruitment of the visual association cortex.
We did not find a correlation between hippocampal activation and memory performance in the patients with depression. This is in contrast to the findings in a study using a word generation task in patients with geriatric depression (61), which showed hippocampal (and anterior cingulate) deficits that correlated with deficits in baseline memory scores (outside of the scanner) derived from the Dementia Rating Scale. That study had several important differences from the current one, including the age of the study group, differences in the cognitive task, and the fact that memory ratings did not correspond to the task performed during the imaging study.
There are several possible explanations for the findings of the current study. Hypercortisolemia associated with stress and/or depression may lead to dysfunction of the hippocampus during the performance of verbal declarative memory tasks by subjects with depression. We did not obtain blood samples to test this hypothesis, however, and our prior studies did not indicate a correlation between hippocampal volume and a single plasma cortisol measurement (37, 104). Other factors linked to stress, such as decreased brain-derived neurotrophic factor, may lead to hippocampal dysfunction. Stress may independently lead to neurochemical alterations resulting in hippocampal dysfunction and depression, or stress may cause hippocampal dysfunction, which then leads to both the emotional dysregulation and cognitive disturbances seen in depression. It is also possible that altered hippocampal function from birth is a risk factor for the development of depression, rather than a result of stress and/or depressive episodes. With regard to prefrontal functioning, there is emerging evidence that early life experience may influence neurogenesis within the prefrontal cortex. Alternatively, a circuit within the hippocampus may be affected in depression, or alterations in functioning from birth may account for changes in functioning in this area among subjects with depression.
There are several limitations of the current study that should be taken into consideration. The reason for having a fixed order of tasks, with the control tasks followed by the deep encoding tasks, was that once the subjects were aware that this was a test of memory they would remember words in spite of the instructions. However, this design may have led to order effects (e.g., subjects doing better as they went along). Also, the control task was not well matched to the encoding task. However, since the same protocol was applied to the depressed and nondepressed subjects, this should not explain differences across patient groups. The statistical parametric mapping data analysis technique has inherent limitations. For instance, images are transformed to a common anatomical space. Since individual subjects have different brain sizes, this may affect the final results. Also, the assessment of multiple regions of the brain may lead to false results related to multiple comparisons.
The proposed model of an abnormal circuit involving the hippocampus and prefrontal cortex is similar to that proposed for other disorders, including schizophrenia and PTSD. A number of studies have shown abnormalities in structure and functioning in the hippocampus and the functionally connected medial prefrontal cortex in schizophrenia (105–107). These findings raise the question of the specificity of circuit abnormalities in depression versus other psychiatric disorders. Dysfunction in these areas may represent the neural correlate of specific aspects of the disorder, such as hippocampal dysfunction that mediates cognitive abnormalities common to, for instance, both depression and schizophrenia, while abnormalities of the medial prefrontal cortex underlie both attentional deficits and affective blunting, which are common to both disorders. At this time it is not clear why similar circuits should subsume psychiatric disorders that are clearly different. One possibility is that although the neuroanatomical substrates may be similar, there may be differences in neurochemical functioning within the circuits that mediate differential expression of the disorders.
![]() |
![]() |
![]() |
Received May 15, 2002; revision received Sept. 15, 2003; accepted Sept. 18, 2003. From the Departments of Psychiatry and Behavioral Sciences, Radiology, and Internal Medicine (Cardiology) and the Emory Center for Positron Emission Tomography, Emory University School of Medicine, Atlanta; the Atlanta VA Medical Center, Decatur, Ga.; and the Mood and Anxiety Disorders Program, NIMH, Bethesda, Md. Address reprint requests to Dr. Bremner, PET Center/Nuclear Medicine, Emory University Hospital, 1364 Clifton Rd., Atlanta, GA 30322; [email protected] (e-mail). Supported by a National Alliance for Research in Schizophrenia and Affective Disorders Young Investigator Award to Dr. Bremner, NIMH grant MH-56120 to Dr. Bremner, a grant from the VA National Center for Posttraumatic Stress Disorder, and a VA Career Development Award to Dr. Bremner. The authors thank Helen Sayward, M.S., for image processing and data analysis and Jacque Piscitelli, M.S.W., for assistance in data collection.

Figure 1. Statistical Parametric Map Overlaid on an MRI Template Showing Brain Areas of Greater Increases in Blood Flow During a Verbal Memory Encoding Task in Healthy Subjects (N=9) Than in Subjects With Depression (N=18)a
aAreas of greater increases in blood flow included the hippocampus (x=26, y=–14, z=–10) and anterior cingulate (x=12, y=26, z=0) (p<0.001).
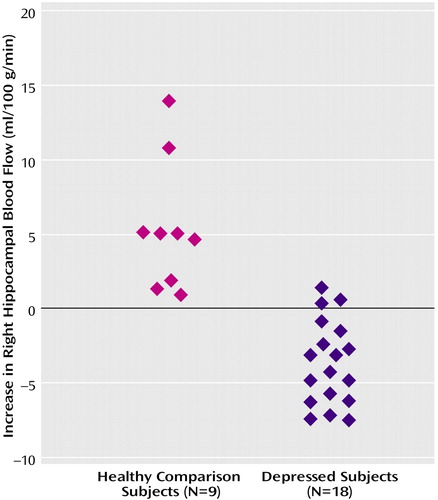
Figure 2. Increased Right Hippocampal Blood Flow During a Verbal Memory Encoding Task in Healthy Subjects (N=9) and Subjects With Depression (N=18)a
aIndividual symbols represent the difference between average blood flow in the right hippocampus (normalized to global blood flow) (x=26, y=–14, z=–10) during the verbal memory encoding task and blood flow during a control task in individual subjects with and without depression. There was an increase in mean hippocampal blood flow in the healthy subjects but not the subjects with depression (two-tailed t test comparing the delta of blood flow with memory versus the control task in patients versus comparison subjects (t=6.52, df=25, p<0.0001).

Figure 3. Statistical Parametric Map Overlaid on an MRI Template Showing Brain Areas of Greater Decreases in Blood Flow During a Verbal Memory Encoding Task in Healthy Subjects (N=9) Than in Subjects With Depression (N=18)a
aAreas of greater decreases in blood flow included the right middle and inferior frontal gyri (p<0.001).
1. Kessler RC, Berglund P, Demler O, Jin R, Koretz D, Merikangas KR, Rush AJ, Walters EE, Wang PS: The epidemiology of major depressive disorder: results from the National Comorbidity Survey Replication (NCS-R). JAMA 2003; 289:3095–3105Crossref, Medline, Google Scholar
2. Stewart WF, Ricci JA, Chee E, Hahn SR, Morganstein D: Cost of lost productive work time among US workers with depression. JAMA 2003; 289:3135–3144; correction, 2003; 290:2218Google Scholar
3. Makino S, Smith MA, Gold PW: Increased expression of corticotropin-releasing hormone and vasopressin messenger ribonucleic acid (mRNA) in the hypothalamic paraventricular nucleus during repeated stress: association with reduction in glucocorticoid mRNA levels. Endocrinology 1995; 136:3299–3309Crossref, Medline, Google Scholar
4. Stanton ME, Gutierrez YR, Levine S: Maternal deprivation potentiates pituitary-adrenal stress responses in infant rats. Behav Neurosci 1988; 102:692–700Crossref, Medline, Google Scholar
5. Ladd CO, Owens MJ, Nemeroff CB: Persistent changes in CRF neuronal systems produced by maternal separation. Endocrinology 1996; 137:1212–1218Crossref, Medline, Google Scholar
6. Plotsky PM, Meaney MJ: Early postnatal stress and the hypothalamic-pituitary-adrenal axis. Mol Brain Res 1993; 18:195–200Crossref, Medline, Google Scholar
7. Sapolsky RM: Why stress is bad for your brain. Science 1996; 273:749–750Crossref, Medline, Google Scholar
8. McEwen BS, Angulo J, Cameron H, Chao HM, Daniels D, Gannon MN, Gould E, Mendelson S, Sakai R, Spencer R, Woolley CS: Paradoxical effects of adrenal steroids on the brain: protection versus degeneration. Biol Psychiatry 1992; 31:177–199Crossref, Medline, Google Scholar
9. Sapolsky RM, Uno H, Rebert CS, Finch CE: Hippocampal damage associated with prolonged glucocorticoid exposure in primates. J Neurosci 1990; 10:2897–2902Crossref, Medline, Google Scholar
10. Magarinos AM, McEwen BS, Flugge G, Fluchs E: Chronic psychosocial stress causes apical dendritic atrophy of hippocampal CA3 pyramidal neurons in subordinate tree shrews. J Neurosci 1996; 16:3534–3540Crossref, Medline, Google Scholar
11. Gould E, Tanapat P, McEwen BS, Flugge G, Fuchs E: Proliferation of granule cell precursors in the dentate gyrus of adult monkeys is diminished by stress. Proc Natl Acad Sci USA 1998; 95:3168–3171Crossref, Medline, Google Scholar
12. Gould E, McEwen BS, Tanapat P, Galea LAM, Fuchs E: Neurogenesis in the dentate gyrus of the adult tree shrew is regulated by psychosocial stress and NMDA receptor activation. J Neurosci 1997; 17:2492–2498Crossref, Medline, Google Scholar
13. Luine V, Villegas M, Martinez C, McEwen BS: Repeated stress causes reversible impairments of spatial memory performance. Brain Res 1994; 639:167–170Crossref, Medline, Google Scholar
14. Diamond DM, Fleshner M, Ingersoll N, Rose GM: Psychological stress impairs spatial working memory: relevance to electrophysiological studies of hippocampal function. Behav Neurosci 1996; 110:661–672Crossref, Medline, Google Scholar
15. Nemeroff CB, Widerlov E, Bissette G, Walleus H, Karlsson I, Eklund K, Kilts CD, Loosen PT, Vale W: Elevated concentrations of CSF corticotropin-releasing factor-like immunoreactivity in depressed patients. Science 1984; 226:1342–1344Crossref, Medline, Google Scholar
16. Sachar EJ, Hellman L, Roffwarg HP, Halpern FS, Fukush DK, Gallagher TF: Disrupted 24 hour patterns of cortisol secretion in psychotic depressives. Arch Gen Psychiatry 1973; 28:19–24Crossref, Medline, Google Scholar
17. Gold PW, Loriaux DL, Roy A, Kling MA, Calabrese JR, Kellner CH, Nieman LK, Post RM, Pickar D, Galluci W: Response to corticotropin-releasing hormone in the hypercortisolism of depression and Cushing’s disease. N Engl J Med 1986; 314:1329–1335Crossref, Medline, Google Scholar
18. Ritchie JC, Belkin BM, Krishnan KRR, Nemeroff CB, Carroll BJ: Plasma dexamethasone concentrations and the dexamethasone suppression test. Biol Psychiatry 1990; 27:159–173Crossref, Medline, Google Scholar
19. Carroll BJ, Curtis GC, Davies BM, Mendels J, Sugarman AA: Urinary free cortisol excretion in depression. J Psychol Med 1976; 6:43–50Crossref, Medline, Google Scholar
20. Rubinow DR, Post RM, Savard R, Gold PW: Cortisol hypersecretion and cognitive impairment in depression. Arch Gen Psychiatry 1984; 41:279–283Crossref, Medline, Google Scholar
21. Young EA, Haskett RF, Grunhaus L: Increased circadian activation of the hypothalamic pituitary adrenal axis in depressed patients in the evening. Arch Gen Psychiatry 1994; 51:701–707Crossref, Medline, Google Scholar
22. Young EA, Haskett RF, Murphy-Weinberg V, Watson SJ, Akil H: Loss of glucocorticoid fast feedback in depression. Arch Gen Psychiatry 1991; 48:693–699Crossref, Medline, Google Scholar
23. Nemeroff CB, Owens MJ, Bissette G, Andorn AC, Stanley M: Reduced corticotropin-releasing factor (CRF) binding sites in the frontal cortex of suicides. Arch Gen Psychiatry 1988; 45:577–579Crossref, Medline, Google Scholar
24. Sternberg DE, Jarvik ME: Memory functions in depression. Arch Gen Psychiatry 1976; 33:219–224Crossref, Medline, Google Scholar
25. Cohen RM, Weingartner H, Smallberg SA, Pickar D, Murphy DL: Effort and cognition in depression. Arch Gen Psychiatry 1982; 145:164–167Google Scholar
26. Weingartner H, Cohen RM, Martello IDI, Gerdt C: Cognitive processes in depression. Arch Gen Psychiatry 1981; 38:42–47Crossref, Medline, Google Scholar
27. Roy-Byrne PP, Weingartner H, Bierer LM, Thompson PM, Post RM: Effortful and automatic cognitive processes in depression. Arch Gen Psychiatry 1986; 43:265–267Crossref, Medline, Google Scholar
28. Danion J-M, Willard-Schroeder D, Zimmerman M-A, Grange D, Schlienger J-L, Singer L: Explicit memory and repetition priming in depression. Arch Gen Psychiatry 1991; 48:707–711Crossref, Medline, Google Scholar
29. Burt DB, Zembar MJ, Niederehe G: Depression and memory impairment: a meta-analysis of the association, its pattern, and specificity. Psychol Bull 1995; 117:285–305Crossref, Medline, Google Scholar
30. Kellner CH, Rubinow DR, Gold PW, Post RM: Relationship of cortisol hypersecretion to brain CT scan alterations in depressed patients. Psychiatry Res 1983; 8:191–197Crossref, Medline, Google Scholar
31. Rothschild A, Benes F, Hebben N, Woods B, Luciana M, Bakanus E, Samson JA, Schatzberg AF: Relationships between brain CT scan findings and cortisol in psychotic and nonpsychotic depressed patients. Biol Psychiatry 1989; 26:565–575Crossref, Medline, Google Scholar
32. Bremner JD, Innis RB, Salomon RM, Staib L, Ng CK, Miller HL, Bronen RA, Duncan J, Krystal JH, Rich D, Malison R, Price LH, Dey H, Soufer R, Charney DS: PET measurement of cerebral metabolic correlates of depressive relapse. Arch Gen Psychiatry 1997; 54:364–374Crossref, Medline, Google Scholar
33. George MS, Ketter TA, Post RM: Prefrontal cortex dysfunction in clinical depression. Depression 1994; 2:59–72Crossref, Google Scholar
34. Bremner JD: Structural changes in the brain in depression and relationship to symptom recurrence. CNS Spectrums 2002; 7:129–139Crossref, Medline, Google Scholar
35. Steffens DC, Krishnan KRR: Structural neuroimaging and mood disorders: recent findings, implications for classification, and future directions. Biol Psychiatry 1998; 43:705–712Crossref, Medline, Google Scholar
36. Mayberg HS, Brannan SK, Mahurin RK, Jerabek PA, Brickman JS, Tekell JL, Silva JA, McGinnis S, Glass TG, Martin CC, Fox PT: Cingulate function in depression: a potential predictor of treatment response. Neuroreport 1997; 8:1057–1061Crossref, Medline, Google Scholar
37. Bremner JD, Narayan M, Anderson ER, Staib LH, Miller HL, Charney DS: Hippocampal volume reduction in major depression. Am J Psychiatry 2000; 157:115–117Link, Google Scholar
38. Sha PJ, Ebmeier KP, Glabus MF, Goodwin GM: Cortical grey matter reductions associated with treatment-resistant chronic unipolar depression. Br J Psychiatry 1998; 172:527–532Crossref, Medline, Google Scholar
39. Krishnan KRR, Doraiswamy PM, Figiel GS, Husain MM, Shaw SA, Na C, Boyko OB, McDonald WM, Nemeroff CB, Ellinwood EH Jr: Hippocampal abnormalities in depression. J Neuropsychiatry Clin Neurosci 1991; 3:387–391Crossref, Medline, Google Scholar
40. Sheline YI, Sanhavi M, Mintun MA, Gado MU: Depression duration but not age predicts hippocampal volume loss in medically healthy women with recurrent major depression. J Neurosci 1999; 19:5034–5043Crossref, Medline, Google Scholar
41. Vakili K, Pillay SS, Lafer B, Fava M, Renshaw PF, Bonello-Cintron CM, Yurgelun-Todd DA: Hippocampal volume in primary unipolar major depression: a magnetic resonance imaging study. Biol Psychiatry 2000; 47:1087–1090Crossref, Medline, Google Scholar
42. Steffens DC, Byrum CE, McQuoid DR, Greenburg DL, Payne ME, Blichington TT, McFall JR, Krishnan KRR: Hippocampal volume in geriatric depression. Biol Psychiatry 2000; 48:301–309Crossref, Medline, Google Scholar
43. Mervaala E, Fohr J, Konogen M, Valkonen-Korhonen M, Vainio P, Partanen J, Tiihonen J, Vinamaki H, Karjalainen AK, Lehtonen J: Quantitative MRI of the hippocampus and amygdala in severe depression. Psychol Med 2000; 30:117–125Crossref, Medline, Google Scholar
44. Sheline YI: 3D MRI studies of neuroanatomical changes in unipolar major depression: the role of stress and medical comorbidity. Biol Psychiatry 2000; 48:791–800Crossref, Medline, Google Scholar
45. Sheline YI, Wang P, Gado M, Csernansky J, Vannier M: Hippocampal atrophy in major depression. Proc Natl Acad Sci USA 1996; 93:3908–3913Crossref, Medline, Google Scholar
46. Pantel J, Schroder J, Essig M, Popp D, Deeh H, Knopp MV, Scherd LR, Eysenbach K, Backenstrass M, Friedlinger M: Quantitative magnetic resonance imaging in geriatric depression and primary degenerative dementia. J Affect Disord 1997; 42:69–83Crossref, Medline, Google Scholar
47. Ashtari M, Greenwald BS, Kramer-Ginsberg E, Hu J, Wu H, Patel M, Aupperle P, Pollock S: Hippocampal/amygdala volumes in geriatric depression. Psychol Med 1999; 29:629–638Crossref, Medline, Google Scholar
48. Drevets WC, Price JL, Simpson JRJ, Todd RD, Reich T, Vannier M, Raichle ME: Subgenual prefrontal cortex abnormalities in mood disorders. Nature 1997; 386:824–827Crossref, Medline, Google Scholar
49. Lai TJ, Payne ME, Byrum CE, Steffens D, Krishnan KR: Reduction of orbital frontal cortex volume in geriatric depression. Biol Psychiatry 2000; 48:971–975Crossref, Medline, Google Scholar
50. Bremner JD, Vythilingam M, Vermetten E, Nazeer A, Adil J, Khan S, Staib LH, Charney DS: Reduced volume of orbitofrontal cortex in major depression. Biol Psychiatry 2002; 51:273–279Crossref, Medline, Google Scholar
51. Ebert D, Feistel H, Barocka A: Effects of sleep deprivation on the limbic system and the frontal lobes in affective disorders: a study with Tc-99m-HMPAO SPECT. Psychiatry Res Neuroimaging 1991; 40:247–251Crossref, Medline, Google Scholar
52. Bench CJ, Friston KJ, Brown RG, Scott LC, Frackowiak RSJ, Dolan RJ: The anatomy of melancholia: focal abnormalities of cerebral blood flow in major depression. Psychol Med 1992; 22:607–615Crossref, Medline, Google Scholar
53. Baxter LR, Schwartz JM, Phelps ME, Mazziotta JC, Guze BH, Selin CE, Gerner RH, Smida RM: Reduction of prefrontal cortex glucose metabolism common to three types of depression. Arch Gen Psychiatry 1989; 46:243–249Crossref, Medline, Google Scholar
54. Martinot JL, Hardy P, Feline A, Huret J-D, Mazoyer B, Attar-Levy D, Pappata S, Syrota A: Left prefrontal glucose hypometabolism in the depressed state: a confirmation. Am J Psychiatry 1990; 147:1313–1317Link, Google Scholar
55. Mayberg HS, Lewis PJ, Regenold W, Wagner HN: Paralimbic hypoperfusion in unipolar depression. J Nucl Med 1994; 35:929–934Medline, Google Scholar
56. Mayberg HS: Frontal lobe dysfunction in secondary depression. J Neuropsychiatry Clin Neurosci 1994; 6:428–442Crossref, Medline, Google Scholar
57. Biver F, Goldman S, Delvenne V, Luxen A, De Maertelaer V, Hubain P, Mendlewicz J, Lotstra F: Frontal and parietal metabolic disturbances in unipolar depression. Biol Psychiatry 1994; 36:381–388Crossref, Medline, Google Scholar
58. Mayberg HS, Starkstein SE, Peyser CE, Brandt J, Dannals RF, Folstein SE: Paralimbic frontal lobe hypometabolism in depression associated with Huntington’s disease. Neurology 1992; 42:1791–1797Crossref, Medline, Google Scholar
59. Mayberg HS, Starkstein SE, Sadzot B, Preziosi T, Andrezejewski PL, Dannals RF, Wagner HN, Robinson RG: Selective hypometabolism in the inferior frontal lobe in depressed patients with Parkinson’s disease. Annal Neurol 1990; 28:57–64Crossref, Medline, Google Scholar
60. Ring RA, Bench CJ, Trimble MR, Brooks DJ, Frackowiak RSJ, Dolan RJ: Depression in Parkinson’s disease: a positron emission study. Br J Psychiatry 1994; 165:333–339Crossref, Medline, Google Scholar
61. de Asis JM, Stern E, Alexopoulos GS, Pan H, Van Gorp W, Blumberg H, Kalayam B, Eidelberg D, Kiosses D, Silbersweig DA: Hippocampal and anterior cingulate activation deficits in patients with geriatric depression. Am J Psychiatry 2001; 158:1321–1323Link, Google Scholar
62. Mann JJ, Malone KM, Diehl DJ, Perel J, Cooper TB, Mintun MA: Demonstration in vivo of reduced serotonin responsivity in the brain of untreated depressed patients. Am J Psychiatry 1996; 153:174–182; correction, 153:588Link, Google Scholar
63. Bremner JD, Vythilingam M, Ng CK, Vermetten E, Nazeer A, Oren D, Berman RM, Charney DS: Regional brain metabolic correlates of positron emission tomographic measurement of alpha-methylparatyrosine-induced depressive symptoms: implications for the neural circuitry of depression. JAMA 2003; 289:3125–3134Crossref, Medline, Google Scholar
64. Squire LR, Ojemann JG, Miezin FM, Petersen SE, Videen TO, Raichle ME: Activation of the hippocampus in normal humans: a functional anatomical study of memory. Proc Natl Acad Sci USA 1992; 89:1837–1841Crossref, Medline, Google Scholar
65. Schacter DL, Alpert NM, Savage CR, Rauch SL, Albert MS: Conscious recollection and the human hippocampal formation: evidence from positron emission tomography. Proc Natl Acad Sci USA 1996; 93:321–325Crossref, Medline, Google Scholar
66. Schacter DL, Savage CR, Alpert NM, Rauch SL, Albert MS: The role of hippocampus and frontal cortex in age-related memory changes: a PET study. Neuroreport 1996; 7:1165–1169Crossref, Medline, Google Scholar
67. Grasby PM, Frith CD, Friston KJ, Bench C, Frackowiak RSJ, Dolan RJ: Functional mapping of brain areas implicated in auditory-verbal memory function. Brain 1993; 116:1–20Crossref, Medline, Google Scholar
68. Tulving E, Kapur S, Markowitsch HJ, Craik FIM, Habib R, Houle S: Neuroanatomical correlates of retrieval in episodic memory: auditory sentence recognition. Proc Natl Acad Sci USA 1994; 91:2012–2015Crossref, Medline, Google Scholar
69. Nyberg L, McIntosh AR, Cabeza R, Habib R, Houle S, Tulving E: General and specific brain regions involved in encoding and retrieval of events: what, where, and when. Proc Natl Acad Sci USA 1996; 93:11280–11285Crossref, Medline, Google Scholar
70. Buckner RL, Petersen SE, Ojemann JG, Miezin FM, Squire LR, Raichle ME: Functional anatomical studies of explicit and implicit memory retrieval tasks. J Neurosci 1995; 15:12–29Crossref, Medline, Google Scholar
71. Shallice T, Fletcher P, Frith CD, Grasby P, Frackowiak RSJ, Dolan RJ: Brain regions associated with acquisition and retrieval of verbal episodic memory. Nature 1994; 368:633–635Crossref, Medline, Google Scholar
72. Andreasen NC, O’Leary DS, Arndt S, Cizadlo T, Hurtig R, Rezai K, Watkins GL, Boles Ponto LL, Hichwa RD: Short-term and long-term verbal memory: a positron emission tomography study. Proc Natl Acad Sci USA 1995; 92:5111–5115Crossref, Medline, Google Scholar
73. Fletcher PC, Frith CD, Grasby PM, Schallice T, Frackowiak RSJ, Dolan RJ: Brain systems of encoding and retrieval of auditory-verbal memory: an in vivo study in humans. Brain 1995; 118:401–416Crossref, Medline, Google Scholar
74. Bremner JD, Soufer R, McCarthy G, Delaney RC, Staib LH, Duncan JS, Charney DS: Gender differences in cognitive and neural correlates of remembrance of emotional words. Psychopharmacol Bull 2001; 35:55–87Medline, Google Scholar
75. Kapur S, Craik FIM, Tulving E, Wilson AA, Houle S, Brown GM: Neuroanatomical correlates of encoding in episodic memory: levels of processing effect. Proc Natl Acad Sci USA 1994; 91:2008–2011Crossref, Medline, Google Scholar
76. Nyberg L, McIntosh AR, Houle S, Nilsson L-G, Tulving E: Activation of medial temporal structures during episodic memory retrieval. Nature 1996; 380:715–717Crossref, Medline, Google Scholar
77. Grasby PM, Frith CD, Friston KJ, Frackowiak RSJ, Dolan RJ: Activation of the human hippocampal formation during auditory-verbal long-term memory function. Neurosci Lett 1993; 163:185–188Crossref, Medline, Google Scholar
78. Dolan RJ, Fletcher PC: Dissociating prefrontal and hippocampal function in episodic memory encoding. Nature 1997; 388:582–585Crossref, Medline, Google Scholar
79. Grady CL, McIntosh AR, Horwitz B, Maisog JM, Ungerleider LG, Mentis MJ, Pietrini P, Schapiro MB, Haxby JV: Age-related reductions in human recognition memory due to impaired encoding. Science 1995; 269:218–221Crossref, Medline, Google Scholar
80. Haxby JV, Horwitz B, Ungerleider LG, Maisog JM, Pietrini P, Grady CL: The functional organization of human extrastriate cortex: a PET-rCBF study of selective attention to faces and locations. J Neurosci 1994; 14:6336–6353Crossref, Medline, Google Scholar
81. Haxby JV, Ungerleider LG, Horwitz B, Maisog JM, Rapoport SI, Grady CL: Face encoding and recognition in the human brain. Proc Natl Acad Sci USA 1996; 93:922–927Crossref, Medline, Google Scholar
82. Kapur N, Friston KJ, Young A, Frith CD, Frackowiak RSJ: Activation of human hippocampal formation during memory for faces: a PET study. Cortex 1995; 31:99–108Crossref, Medline, Google Scholar
83. Schacter DL, Reiman E, Uecker A, Polster MR, Yun LS, Cooper LA: Brain regions associated with retrieval of structurally coherent visual information. Nature 1995; 376:587–590Crossref, Medline, Google Scholar
84. Ghaem O, Mellet E, Crivello F, Tzourio N, Mazoyer B, Berthoz A, Denis M: Mental navigation along recognition in the human brain. Proc Natl Acad Sci USA 1996; 93:922–927Crossref, Medline, Google Scholar
85. Maguire EA, Frackowiak RSJ, Frith CD: Learning to find your way: a role for the human hippocampal retrieval of structurally coherent visual information. Nature 1995; 376:587–590Crossref, Medline, Google Scholar
86. Bremner JD, Vythilingam M, Vermetten E, Southwick SM, McGlashan T, Nazeer A, Khan S, Vaccarino LV, Soufer R, Garg PK, Ng CK, Staib LH, Duncan JS, Charney DS: MRI and PET study of deficits in hippocampal structure and function in women with childhood sexual abuse and posttraumatic stress disorder. Am J Psychiatry 2003; 160:924–932Link, Google Scholar
87. Zola-Morgan SM, Squire LR: The primate hippocampal formation: evidence for a time-limited role in memory storage. Science 1990; 250:288–290Crossref, Medline, Google Scholar
88. Scoville WB, Milner B: Loss of recent memory after bilateral hippocampal lesions. J Neurol Psychiatry 1957; 20:11–21Crossref, Medline, Google Scholar
89. First M, Spitzer R, Williams J, Gibbon M: Structured Clinical Interview for DSM-IV—Non-Patient Edition (SCID-NP, Version 1.0). Washington, DC, American Psychiatric Press, 1995Google Scholar
90. Hamilton M: A rating scale for depression. J Neurol Neurosurg Psychiatry 1960; 23:56–62Crossref, Medline, Google Scholar
91. Bremner JD, Vermetten E, Mazure CM: Development and preliminary psychometric properties of an instrument for the measurement of childhood trauma: the Early Trauma Inventory. Depress Anxiety 2000; 12:1–12Crossref, Medline, Google Scholar
92. Friston KJ, Ashburner J, Frith CD, Poline JB, Heather JD, Frackowiak RS: Spatial registration and normalization of images. Hum Brain Mapp 1995; 2:165–189Crossref, Google Scholar
93. Matthew E, Andreason P, Carson RE, Herscovitch P, Pettigrew K, Cohen R, King C, Johanson CE, Paul SM: Reproducibility of resting cerebral blood flow measurements with H2(15)O positron emission tomography in humans. J Cereb Blood Flow Metab 1993; 13:748–754Crossref, Medline, Google Scholar
94. Li HH, Votaw JR: Optimization of PET activation studies based on the SNR measured in the 3-D Hoffman Brain Phantom. IEEE Trans Med Imaging 1998; 17:596–606Crossref, Medline, Google Scholar
95. Friston KJ: Statistical parametric mapping, in Functional Neuroimaging: Technical Foundations. Edited by Thatcher RW, Hallett M, Zeffiro T, John ER, Huerta M. San Diego, Academic Press, 1994, pp 79–93Google Scholar
96. Friston KJ, Frith CD, Liddle PF, Frackowiak RS: Comparing functional (PET) images: the assessment of significant change. J Cereb Blood Flow Metab 1991; 11:690–699Crossref, Medline, Google Scholar
97. Talairach J, Tournoux P: Co-Planar Stereotaxic Atlas of the Human Brain: Three-Dimensional Proportional System. New York, Thieme Medical, 1988Google Scholar
98. Schallice T, Fletcher P, Frith CD, Grasby P, Frackowiak RSJ, Dolan RJ: Brain regions associated with acquisition and retrieval of verbal episodic memory. Nature 1994; 368:633–635Crossref, Medline, Google Scholar
99. Tulving E, Kapur S, Craik FIM, Moscovitch M, Houle S: Hemispheric encoding/retrieval asymmetry in episodic memory: positron emission tomography findings. Proc Natl Acad Sci USA 1994; 91:2016–2020Crossref, Medline, Google Scholar
100. Petersen SE, Fox PT, Posner MI, Mintun MA, Raichle ME: Positron emission tomographic studies of the cortical anatomy of single word processing. Nature 1988; 331:585–589Crossref, Medline, Google Scholar
101. Frith CD, Friston K, Liddle PF, Frackowiak RS: Willed action and the prefrontal cortex in man: a study with PET. Proc R Soc Lond B Biol Sci 1991; 244:241–246Crossref, Medline, Google Scholar
102. Goldman-Rakic PS: Topography of cognition: parallel distributed networks in primate association cortex. Annu Rev Neurosci 1988; 11:137–156Crossref, Medline, Google Scholar
103. McCarthy G: Functional neuroimaging of memory. Neuroscientist 1995; 1:155–163Crossref, Google Scholar
104. Bremner JD, Narayan M, Charney DS: Reply to LT Van Elst: Hippocampus and amygdala pathology in depression (letter). Am J Psychiatry 2001; 158:653Link, Google Scholar
105. Nelson MD, Saykin AJ, Flashman LA, Riordan HJ: Hippocampal volume reduction in schizophrenia as assessed by magnetic resonance imaging: a meta-analytic study. Arch Gen Psychiatry 1998; 55:433–440Crossref, Medline, Google Scholar
106. Tamminga CA, Thaker GK, Buchanan R, Kirkpatrick B, Alphs LD, Chase TN, Carpenter WT: Limbic system abnormalities identified in schizophrenia using positron emission tomography with fluorodeoxyglucose and neocortical alterations with deficit syndrome. Arch Gen Psychiatry 1992; 49:522–530Crossref, Medline, Google Scholar
107. Shenton ME, Kikinis R, Jolesz FA, Pollak SD, Lemay M, Wible CG, Hokama H, Martin J, Metcalf D, Coleman M, McCarley RW: Abnormalities of the left temporal lobe and thought disorder in schizophrenia: a quantitative magnetic resonance imaging study. N Engl J Med 1992; 327:604–612Crossref, Medline, Google Scholar