An fMRI Study of Episodic Encoding and Recognition of Words in Patients With Schizophrenia in Remission
Abstract
OBJECTIVE: Verbal memory deficits are among the most severe cognitive deficits observed in patients with schizophrenia. This study examined patterns of brain activity during episodic encoding and recognition of words in patients with schizophrenia. METHOD: Functional magnetic resonance imaging (fMRI) was used to study regional brain activation in 10 healthy male comparison subjects and 10 male outpatients with schizophrenia during performance of a modified version of the words subtest of Warrington’s Recognition Memory Test. RESULTS: Despite having intact performance in word recognition, the patients with schizophrenia had less activation of the right dorsolateral and anterior prefrontal cortex, right anterior cingulate, and left lateral temporal cortex during word encoding, compared with the healthy comparison subjects. During word recognition, the patients had impairments in activation of the bilateral dorsolateral prefrontal and lateral temporal cortices. CONCLUSIONS: Schizophrenia was associated with attenuated frontotemporal activation during episodic encoding and recognition of words. These results from an fMRI study replicate earlier findings derived from a positron emission tomography study.
Saykin and co-workers (1) reported that patients with schizophrenia are distinguished from healthy comparison subjects by the selectivity and relative severity of the patients’ impairment in verbal learning and memory, compared with other neuropsychological functions. Such an impairment has been found for different types of stimuli (e.g., words, sentences, stories, and nonsense syllables), in a variety of research paradigms (e.g., paired associates, free recall, cued recall, recognition formats, and repetition priming), and within a large range of schizophrenia patient groups (e.g., in patients with acute, chronic, reactive, and late-onset schizophrenia and in patients with tardive dyskinesia) (2).
Recently, Tracy and co-workers (3) found that patients with schizophrenia have a general impairment of episodic memory, as evidenced by deficits in encoding in both verbal and nonverbal memory tests. However, recognition memory for words appeared to be preserved, in the context of deficits in initial learning and free recall. The authors attributed these findings to a retrieval deficit in schizophrenic patients that was specific to the type of stimulus material.
Schizophrenic patients have been found to have abnormalities in frontal (4) and temporal (5) structures that previous studies have identified as important for human memory performance (6). Gur and co-workers (7) reported correlations between frontal and temporal volume deficits and impaired memory performance in schizophrenia. Regarding functional abnormalities, studies of cerebral blood flow (CBF) that have used verbal memory tasks have detected lower levels of prefrontal activation in patients with schizophrenia than in healthy comparison subjects (8–10). Hazlett and co-workers (11) found that patients with schizophrenia have lower relative glucose metabolic rates in both the frontal and the lateral temporal cortices during a serial verbal learning task. In accordance with other investigators (12), they suggested that an abnormality in the functional connectivity between these structures plays a central role in the pathophysiology of cognitive dysfunction in schizophrenia. Similar observations were made by Ragland and co-workers (13), who contrasted episodic encoding and recognition of words by measuring CBF with positron emission tomography (PET) in outpatients with schizophrenia and in healthy subjects. In that study, patients had deficits in activation of the left frontotemporal regions during word encoding and deficits in activation of the left frontotemporal regions, anterior cingulate, and right thalamus during word recognition. Approximately one-half of the patients were neuroleptic naive, and the others were treated with conventional antipsychotics, with second-generation antipsychotic agents (e.g., clozapine or risperidone), or with both types of agents. As there were also sex differences between the patient and comparison groups in the study by Ragland et al., we attempted to replicate the findings of Ragland et al. by using functional magnetic resonance imaging (fMRI) to investigate the neural circuitry used during episodic encoding and recognition of words in male schizophrenia outpatients, all of whom were treated with second-generation antipsychotics, and in healthy male comparison subjects. We chose to study the pathophysiology of hypothetical core and persistent impairments associated with schizophrenia rather than the transient changes associated with episodes of psychotic relapse. Therefore, the study subjects were stable outpatients with schizophrenia in remission, rather than acutely ill patients, and healthy volunteers.
Method
Subjects
Ten German-speaking male outpatients with schizophrenia were recruited for the study. The diagnostic criteria for a schizophrenic disorder according to ICD-10 served as the basis for study inclusion. All patients had received a clinical diagnosis from their treating psychiatrist before entry into the study. They were reassessed by the first or second author (both psychiatrists) on the day of the fMRI scan. The patients’ mean scores on the positive, negative, and global scales of the Positive and Negative Syndrome Scale (14) were 11.7 (SD=6.8), 14.6 (SD=5.2), and 23.3 (SD=4.5), respectively. Their mean age was 32.5 years (SD=6.4), their mean number of years of education was 12.4 (SD=3.2), and the mean time from their first hospitalization was 76.1 months (SD=42.3). All of the patients had been hospitalized on at least one previous occasion while experiencing positive symptoms. At the time of the study, they had been clinically stable for at least 6 months. All were taking second-generation antipsychotics and had been taking fixed doses for at least 1 month before the fMRI scan. Four patients were taking risperidone (mean dose=4.0 mg/day), three were taking olanzapine (mean dose=11.7 mg/day, SD=7.6, range=5–20), and three were treated with clozapine (mean dose=350 mg/day, SD=132.3, range=200–450). No patient received any concomitant medications. The comparison group consisted of 10 German-speaking, age- and sex-matched, healthy volunteers (mean age=29.2 years, SD=6.7; mean number of years of education=14.9, SD=3.5). They had no history of substance abuse or of any other medical, psychiatric, or neurological disorder that might affect CNS function. All subjects were strongly right-handed, as measured by their scores on the Edinburgh Handedness Scale (15) (mean score=18.8, SD=1.9, for the comparison subjects; mean score=18.6, SD=2.3, for the patients).
To guarantee their understanding of the verbal memory task, all subjects practiced performing the words subtest of Warrington’s Recognition Memory Test (16) before fMRI scanning. Written informed consent was obtained from each patient and each comparison subject. The study was approved by the Ethics Committee of the Medical Faculty of Innsbruck University.
Task
The words subtest of Warrington’s Recognition Memory Test (16) assesses material-specific memory deficits in adults by presenting test subjects with a list of 50 commonly used one-syllable words. Test subjects are instructed to indicate whether they like or dislike the item represented by each word. Recognition is subsequently assessed by asking the subject to choose the target word when it is presented in a forced-choice procedure and paired with a similar distractor word. Stimulus items are shown at a rate of one every 3 seconds.
fMRI Examination
We used a blocked periodic design in which a 40-second rest condition was followed by an activation condition of equal length. During both the encoding and the recognition tasks, the cycle of alternation between these conditions was repeated three times in the course of 4 minutes each. The resting baseline reference task was a standard condition during which subjects were instructed to lie still and remain quiet with their eyes open (17). For stimulation during the activation condition, we used a modified version of the words subtest of the Recognition Memory Test for personal computers and projected the material on a screen placed in front of the MRI scanner. Stimuli were presented at a rate of one every 3 seconds and were visible to the participant by means of an angled mirror placed above the head coil. Words were centered on the screen and presented as black block letters against a white background. During the encoding task, the subjects were asked to indicate whether they liked or disliked the item by means of a right index or middle finger press on a two-button response box. This task used all 50 items from the original test. As a main focus of our study was to compare our findings with previous PET findings, we adapted the retrieval task of the words subtest of the Recognition Memory Test to the procedure used by Ragland and colleagues (13). Therefore, the recognition task consisted of 25 of the 50 items that were included in the encoding task and 25 distractor items, which were presented in random order. The subjects were instructed to indicate by means of a key press whether each stimulus appeared familiar or unfamiliar. Responses were recorded by hand and were classified as correct or incorrect. We used only 50 of the 100 words from the original recognition test to allow for the same amount of time for fMRI scanning during both the encoding and recognition procedures. During the resting baseline reference condition, a blank white screen appeared.
Scanning Procedures and Analysis
fMRI was performed on a 1.5-T magnetic resonance scanner (Magnetom VISION, Siemens, Erlangen, Germany) (gradient rise time=300 μsec, 25 mT/msec) by using a circularly polarized head coil (field of view=250 mm). For functional imaging, a T2*-weighted, single-shot, echo-planar sequence was used (echo time=64 msec, echo spacing=1.64 msec, field of view=250 mm, matrix=64×128, slice thickness=3 mm, voxel dimension=2.94×1.95×4.5 mm3, flip angle=90°). A total of 120 blood-oxygenation-level-dependent (BOLD) contrast-sensitive images with an interslice gap of 0.5 mm were acquired at each of 15 planes parallel to the intercommissural line (18, 19). Rest conditions were alternated with activation conditions, each consisting of 10 images at 4-second intervals (repetition time). Before each time series, five dummy images were collected to reach scanner equilibrium. These images were excluded from the following analyses.
Image analysis was performed offline on a SPARC Ultra 1 workstation (Sun Microsystems, Santa Clara, Calif.) by using MATLAB and SPM99 statistical parametric mapping software (20–22). The 60 volume images of each task were automatically realigned to the first image of the time series to correct for head movement between scans. Low-frequency artifacts that were assumed to arise from cardiorespiratory and other cyclical components were removed with high-pass filtering (0.5 cycle/minute) of the time series. The functional data sets from each subject were slightly smoothed with a Gaussian filter with a root-mean-square radius of 4×4×9 mm (for the x, y, and z axes, respectively) to suppress high-frequency noise in the images and to improve signal-to-noise ratio. The alternating periods of rest and activation were modeled by using a simple, smoothed, delayed half-sine-wave reference vector to take into account the delayed CBF changes after stimulus presentation. The general linear model approach for time series data was used to search for significantly activated voxels. We defined a design matrix comprising contrast testing for significant activation during the activation condition versus the rest condition. The within-group t statistics were expressed as standardized z scores in final projection maps. The threshold for significant activation was p<0.001, uncorrected. In addition, to be identified as activated, a voxel had to be part of a cluster of 150 or more contiguous voxels that exceeded the threshold. Anatomical location of the activated foci was assigned with the aid of the atlas of Talairach and Tournoux (23). In a final processing step, we performed group analyses and subtracted the value at activation minus the value at rest for the patients from the corresponding value for the comparison subjects. The threshold for significant activation was p<0.001, uncorrected. Deactivation was calculated correspondingly. With the threshold criteria that were used, the probability of finding a region activated or deactivated by chance was <5%.
Results
No significant differences between groups were found for age (p>0.1), years of education (p>0.1), or task performance (p>0.5). We were not able to record reaction time data. However, recognition levels were high for both groups (percentage of items correctly recognized: mean=91.2%, SD=5.5%, for comparison subjects; mean=90.6%, SD=11.4%, for patients).
fMRI Data
Word encoding
Locations of significant increases in BOLD signal during word encoding are presented in Table 1 and Figure 1. The comparison subjects showed prefrontal activations in bilateral Brodmann’s areas 44 and 10 and in the right Brodmann’s area 47. Further effects were seen in the left anterior cingulate (Brodmann’s area 32) and in the left angular gyrus (Brodmann’s area 39). Deactivation was found in the right lingual gyrus (Brodmann’s area 18). In patients, activation was detected in the left Brodmann’s area 45, left precentral gyrus (Brodmann’s area 6), and bilateral occipital cortices (Brodmann’s area 18), while the bilateral cunei (Brodmann’s area 18) were deactivated. By subtracting the activation values of patients from those of healthy subjects, we found greater activation in the right prefrontal cortex (Brodmann’s areas 9 and 10), right anterior cingulate (Brodmann’s area 32), and left lateral temporal cortex (Brodmann’s area 22) in the comparison subjects. Greater deactivation was found in the patients’ bilateral dorsolateral prefrontal cortices (Brodmann’s area 9), right anterior cingulate (Brodmann’s area 24), right lateral temporal cortex (Brodmann’s area 22), right insula, right thalamus, and bilateral occipital cortices (Brodmann’s area 18).
Word recognition
Word recognition activated widespread areas in both hemispheres. The locations of these areas are presented in Table 2 and Figure 2. Regarding frontal lobe activation, the comparison subjects showed effects in the bilateral ventrolateral (bilateral Brodmann’s area 44, left Brodmann’s areas 45 and 47) and right dorsolateral (Brodmann’s area 46) regions, left supplementary motor area (Brodmann’s area 6), right anterior cingulate (Brodmann’s area 32), and bilateral occipital cortices (Brodmann’s areas 18 and Brodmann’s area 19). Additional effects were detected in the left thalamus and in the bilateral subthalamic nuclei. Deactivation was found in the bilateral Brodmann’s area 10. In patients, activation was seen in the left Brodmann’s area 44 and in the left inferior parietal lobule (Brodmann’s area 40), while the right posterior cingulate (Brodmann’s area 31) and bilateral cunei (left Brodmann’s area 30, right Brodmann’s area 18) were deactivated. The comparison between healthy subjects and patients revealed that healthy subjects had relatively greater activation in the bilateral dorsolateral prefrontal cortices (Brodmann’s area 46), left Brodmann’s area 6, and bilateral lateral temporal cortices (bilateral Brodmann’s area 21, left Brodmann’s area 22). On the other hand, they showed greater deactivation in the bilateral Brodmann’s area 10 extending to the anterior cingulate (Brodmann’s area 32), left posterior cingulate (Brodmann’s area 30), and right cuneus (Brodmann’s area 18).
Discussion
The findings of this fMRI study of episodic memory in schizophrenia, contrasting encoding and recognition of words in the same experiment, replicated previous neuropsychological (3) and PET findings (13) showing a lower level of frontotemporal activation in patients with schizophrenia, compared to healthy subjects, despite intact recognition performance.
The activation pattern that we observed during episodic encoding in the comparison group was for the most part consistent with previous reports (24–31). Only our finding of right hemispheric activations in the frontal cortex did not confirm the hemispheric encoding/retrieval asymmetry model (32). However, previous studies have shown that the encoding of verbal materials is not invariably left-lateralized. Lee and co-workers (29) described activations in the bilateral anterior frontal cortices when activation values for verbal retrieval were subtracted from those for verbal encoding, and Otten et al. (31) found bilateral ventrolateral prefrontal activations when subjects subsequently remembered words they had judged to refer to animate things. As suggested by Mottaghy et al. (28), the use of verbal materials with a high content of object imagery might be one reason for such bilateral prefrontal activations. Furthermore, in the current study all the material was familiar to the subjects, since it had been presented before scanning. Such a condition has been associated with activation of the right ventrolateral prefrontal cortex (33). With regard to episodic memory recognition, our findings for the healthy subjects corroborate the findings of other researchers (13, 25–28, 30, 34–38).
Differences between the patients and the comparison subjects during encoding were found in the right dorsolateral and anterior prefrontal regions, right anterior cingulate, and left lateral temporal cortex. During recognition, patients had impairments in activation in the bilateral dorsolateral prefrontal and lateral temporal cortices.
The left dorsolateral prefrontal and right anterior prefrontal regions have been associated with verbal working memory in healthy individuals (39). Correspondingly, impairment of working memory and executive functions in schizophrenia has been associated with decreased blood flow in the dorsolateral prefrontal cortex (40). Our finding of a lower level of activation in these regions for the patients than for the comparison subjects might, therefore, be related to impaired working memory in schizophrenia. However, levels of activation during recognition were comparable in both groups, which indicates that the patients’ working memory abilities permitted successful encoding. In agreement with Ragland and co-workers (13), we suggest that the lower level of prefrontal activation during episodic encoding in the patient group may represent executive difficulties. This suggestion is further supported by our finding of a lower level of activity in the anterior cingulate in the schizophrenia group. This structure has been proposed to play a prominent role in the executive control of cognition (41). The lack of anterior cingulate activation may reflect difficulties in both initiating appropriate responses and suppressing inappropriate ones, especially when attentional demands are high.
During both encoding and recognition, relatively lower activity was seen in the patients’ lateral temporal cortices. Similar findings have been reported by Ganguli et al. (8), who investigated changes in regional CBF associated with the performance of an auditory verbal supraspan memory task. The lateral temporal cortex (Brodmann’s areas 21 and 22) (42, 43) has been associated with the semantic processing of words. Thus, difficulties in this area may be associated with the differences between the patients and the healthy subjects that were observed in lateral temporal activation during episodic recognition.
Animal studies have implicated a cortical circuit involving the dorsolateral prefrontal cortex and parietal, temporal, and thalamic regions in working memory (44). The disruption of an equivalent circuit in humans could be responsible for the executive dysfunction observed in patients with schizophrenia.
Several details of our study design that may limit the generalizability of the results must be considered. First, the study included a small number of subjects, and all subjects were male. Some neuropsychological studies of gender differences in cognition in schizophrenia have demonstrated more impairment among male patients in several functional domains (45, 46), whereas others have indicated either no gender differences (47, 48) or even greater abnormalities among women (46, 49, 50). Further studies are needed to elucidate the effect of gender on memory performance and brain activation in schizophrenia. Second, the patients in the study were stable outpatients with schizophrenia in remission who were treated with novel antipsychotics. These drugs have been associated with improved performance on cognitive tasks (51). Consequently, our study group may have already profited from such a therapeutic effect, and therefore they would not have the dysfunctions of untreated schizophrenia when they joined the study. However, episodic memory impairments in schizophrenia have also been found in studies that have reduced the confounding effects of medication (2), and there is no evidence that long-term neuroleptic exposure in schizophrenia affects CBF in the prefrontal and/or temporal lobe regions (52). Third, since all subjects had completed the words subtest of the Recognition Memory Test before scanning, training effects must be considered. The activation patterns observed in the study group thus cannot be equated with those that would have emerged without this test run. On the other hand, having subjects complete a prescan test may eliminate artificial findings resulting from mere differences between participants in ability to follow test procedures. Last, we chose to contrast encoding and recognition with a resting baseline reference condition rather than performing serial subtractions of increasingly complex reference tasks (53). We were therefore not able to control for nonmemory functions. However, the use of active reference conditions has the potential limitation of confounding the interpretation of activation effects if cognitive components interact between one or more reference tasks (54). Indeed, this study was not designed to look for cognitive differences between the patients and the healthy comparison subjects but to look for differences of functional networks employed to process the tasks in question. Nevertheless, our findings would have been strengthened by including a motor baseline task with the resting baseline task to control for motor activation during the acquisition and recognition conditions.
Our findings are highly clinically relevant insofar as encoding and recognition are needed both for everyday life and for participation in the workforce. Therefore, deficiencies in this area may render psychosocial rehabilitation efforts more difficult. The study findings add to our understanding of neuropsychological dysfunction in patients with schizophrenia and support the need to take such specific impairments into account when planning treatment.
![]() |
![]() |
Received Feb. 11, 2002; revisions received June 11 and Oct. 21, 2002; accepted Oct. 28, 2002. From the Departments of Biological Psychiatry and Radiology, Innsbruck University Clinics. Address reprint requests to Dr. Hofer, Department of Biological Psychiatry, Innsbruck University Clinics, Anichstrasse 35, A-6020 Innsbruck, Austria; [email protected] (e-mail). Supported by grant 7359 from the Österreichische Nationalbank (National Bank of Austria).
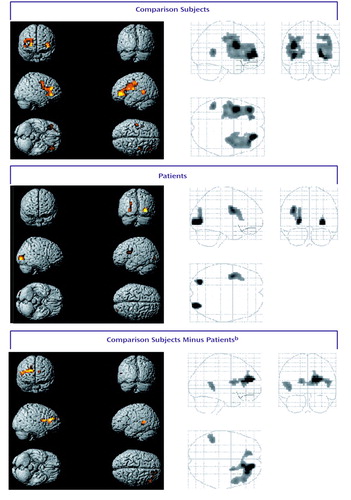
Figure 1. Statistical Parametric Maps Showing Areas of Significant Brain Activation During Word Encoding for 10 Healthy Comparison Subjects and 10 Patients With Schizophreniaa
aAreas of significant activation, shown in color, were identified on the basis of blood-oxygen-level-dependent signal values during encoding minus values during baseline (p<0.001, uncorrected). For localization of activation, see Table 1.
bAreas of significant difference in activation between groups.
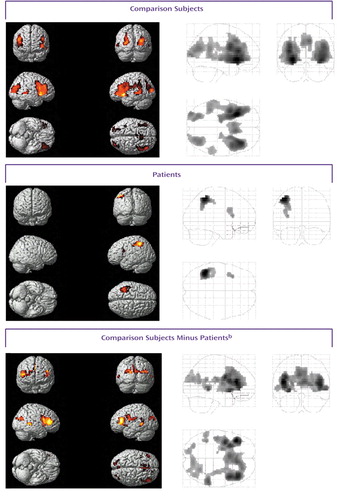
Figure 2. Statistical Parametric Maps Showing Areas of Significant Brain Activation During Word Recognition for 10 Comparison Subjects and 10 Patients With Schizophreniaa
aAreas of significant activation, shown in color, were identified on the basis of blood-oxygen-level-dependent signal values during retrieval minus values during baseline (p<0.001, uncorrected). For localization of activation, see Table 2.
bAreas of significant difference in activation between groups.
1. Saykin AJ, Gur RC, Gur RE, Mozley D, Mozley LH, Resnick SM, Kester B, Stafiniak P: Neuropsychological function in schizophrenia: selective impairment in memory and learning. Arch Gen Psychiatry 1991; 48:618-624Crossref, Medline, Google Scholar
2. Paulsen JS, Heaton RK, Sadek JR, Perry W, Delis DC, Braff D, Kuck J, Zisook S, Jeste DV: The nature of learning and memory impairments in schizophrenia. J Int Neuropsychol Soc 1995; 1:88-99Crossref, Medline, Google Scholar
3. Tracy JI, Mattson R, King C, Bundick T, Celenza MA, Glosser G: A comparison of memory for verbal and non-verbal material in schizophrenia. Schizophr Res 2001; 50:199-211Crossref, Medline, Google Scholar
4. Goldman-Rakic PS, Selemon LD: Functional and anatomical aspects of prefrontal pathology in schizophrenia. Schizophr Bull 1997; 23:437-458Crossref, Medline, Google Scholar
5. Arnold SE: The medial temporal lobe in schizophrenia. J Neuropsychiatry Clin Neurosci 1997; 9:460-470Crossref, Medline, Google Scholar
6. Gabrieli JDE: Cognitive neuroscience of human memory. Annu Rev Psychol 1998; 49:87-115Crossref, Medline, Google Scholar
7. Gur RE, Cowell P, Turetsky BI, Gallacher F, Cannon T, Bilker W, Gur RC: A follow-up magnetic resonance imaging study of schizophrenia: relationship of neuroanatomical changes to clinical and neurobehavioral measures. Arch Gen Psychiatry 1998; 55:145-152Crossref, Medline, Google Scholar
8. Ganguli R, Carter C, Mintun M, Brar J, Becker J, Sarma R, Nichols T, Bennington E: PET brain mapping study of auditory verbal supraspan memory versus visual fixation in schizophrenia. Biol Psychiatry 1997; 41:33-42Crossref, Medline, Google Scholar
9. Fletcher PC, McKenna PJ, Frith CD, Grasby PM, Friston KJ, Dolan RJ: Brain activations in schizophrenia during a graded memory task studied with functional imaging. Arch Gen Psychiatry 1998; 55:1001-1008Crossref, Medline, Google Scholar
10. Crespo-Facorro B, Paradiso S, Andreasen NC, O’Leary DS, Watkins GL, Boles Ponto LL, Hichwa RD: Recalling word lists reveals “cognitive dysmetria” in schizophrenia: a positron emission tomography study. Am J Psychiatry 1999; 156:386-392Abstract, Google Scholar
11. Hazlett EA, Buchsbaum MS, Jeu LA, Nenadic I, Fleischman MB, Shihabuddin L, Haznedar MM, Harvey PD: Hypofrontality in unmedicated schizophrenia patients studied with PET during performance of a serial verbal learning task. Schizophr Res 2000; 43:33-46Crossref, Medline, Google Scholar
12. Ragland JD, Gur RC, Glahn DC, Censits DM, Smith RJ, Lazarev MG, Alavi A, Gur RE: Frontotemporal cerebral blood flow change during executive and declarative memory tasks in schizophrenia: a positron emission tomography study. Neuropsychology 1998; 12:399-413Crossref, Medline, Google Scholar
13. Ragland JD, Gur RC, Raz J, Schroeder L, Kohler CG, Smith RJ, Alavi A, Gur RE: Effect of schizophrenia on frontotemporal activity during word encoding and recognition: a PET cerebral blood flow study. Am J Psychiatry 2001; 158:1114-1125Link, Google Scholar
14. Kay SR, Fiszbein A, Opler LA: The Positive and Negative Syndrome Scale (PANSS) for schizophrenia. Schizophr Bull 1987; 13:261-276Crossref, Medline, Google Scholar
15. Oldfield RC: The assessment and analysis of handedness: the Edinburgh Inventory. Neuropsychologia 1971; 9:97-113Crossref, Medline, Google Scholar
16. Warrington EK: Recognition Memory Test. Windsor, UK, Nelson, 1984Google Scholar
17. Gur RC, Gur RE, Obrist WD, Hungerbuhler JP, Younkin D, Rosen AD, Skolnick BE, Reivich M: Sex and handedness differences in cerebral blood flow during rest and cognitive activity. Science 1982; 217:659-661Crossref, Medline, Google Scholar
18. Kwong KK: Functional magnetic resonance imaging with echo planar imaging. Magn Reson Q 1995; 11:1-20Medline, Google Scholar
19. Ogawa S, Lee TM, Kay AR, Tank DW: Brain magnetic resonance imaging with contrast dependent blood oxygenation. Proc Natl Acad Sci USA 1990; 87:9868-9872Crossref, Medline, Google Scholar
20. Friston KJ, Ashburner J, Poline JB, Frith CD, Heather JD, Frackowiak RSJ: Spatial registration and normalization of images. Hum Brain Mapp 1995; 2:165-189Crossref, Google Scholar
21. Friston KJ, Holmes AP, Worsley KJ, Poline JB, Frith CD, Frackowiak RSJ: Statistical parametric mapping in functional imaging: a general linear approach. Hum Brain Mapp 1995; 2:189-210Crossref, Google Scholar
22. Friston KJ, Holmes AP, Poline JB, Grasby PJ, Williams SCR, Frackowiak RSJ, Turner R: Analysis of fMRI time series revisited. Neuroimage 1995; 2:45-53Crossref, Medline, Google Scholar
23. Talairach J, Tournoux P: Co-Planar Stereotaxic Atlas of the Human Brain: Three-Dimensional Proportional System. New York, Thieme Medical, 1988Google Scholar
24. Kapur S, Tulving E, Cabeza R, McIntosh AR, Houle S, Craik FIM: The neural correlates of intentional learning of verbal materials: a PET study in humans. Brain Res Cogn Brain Res 1996; 4:243-249Crossref, Medline, Google Scholar
25. McDermott KB, Ojemann JG, Petersen SE, Ollinger JM, Snyder AZ, Akbudak E, Conturo TE, Raichle ME: Direct comparison of episodic encoding and retrieval of words: an event-related fMRI study. Memory 1999; 7:661-678Crossref, Medline, Google Scholar
26. Kirchhoff BA, Wagner AD, Maril A, Stern CE: Prefrontal-temporal circuitry for episodic encoding and subsequent memory. J Neurosci 2000; 20:6173-6180Crossref, Medline, Google Scholar
27. Halsband U, Krause BJ, Schmidt D, Herzog H, Tellmann L, Müller-Gärtner HW: Encoding and retrieval in declarative learning: a positron emission tomography study. Behav Brain Res 1998; 97:69-78Crossref, Medline, Google Scholar
28. Mottaghy FM, Shah NJ, Krause BJ, Schmidt D, Halsband U, Jäncke L, Müller-Gärtner HW: Neuronal correlates of encoding and retrieval in episodic memory during a paired-word association learning task: a functional magnetic resonance imaging study. Exp Brain Res 1999; 128:332-342Crossref, Medline, Google Scholar
29. Lee ACH, Robbins TW, Pickard JD, Owen AM: Asymmetric frontal activation during episodic memory encoding: the effects of stimulus type on encoding and retrieval. Neuropsychologia 2000; 38:677-692Crossref, Medline, Google Scholar
30. Ragland JD, Gur RC, Lazarev MG, Smith RJ, Schroeder L, Raz J, Turetsky BI, Alavi A, Gur RE: Hemispheric activation of anterior and inferior prefrontal cortex during verbal encoding and recognition: a PET study of healthy volunteers. Neuroimage 2000; 11:624-633Crossref, Medline, Google Scholar
31. Otten LJ, Henson RNA, Rugg MD: Depth of processing effects on neural correlates of memory encoding: relationship between findings from across- and within-task comparisons. Brain 2001; 124:399-412Crossref, Medline, Google Scholar
32. Tulving E, Kapur S, Craik FIM, Moscovitch M, Houle S: Hemispheric encoding/retrieval asymmetry in episodic memory: positron emission tomography findings. Proc Natl Acad Sci USA 1994; 91:2016-2020Crossref, Medline, Google Scholar
33. Fletcher PC, Dolan RJ: Right prefrontal cortex responds to item familiarity during a memory encoding task. Memory 1999; 7:703-713Crossref, Medline, Google Scholar
34. Wagner AD, Desmond JE, Glover GH, Gabrieli JDE: Prefrontal cortex and recognition memory: functional MRI evidence of context-dependent retrieval processes. Brain 1998; 121:1985-2002Crossref, Medline, Google Scholar
35. Saykin AJ, Johnson SC, Flashman LA, McAllister TW, Sparling M, Darcey TM, Moritz CH, Guerin SJ, Weaver J, Mamourian A: Functional differentiation of medial temporal and frontal regions involved in processing novel and familiar words: an fMRI study. Brain 1999; 122:1963-1971Crossref, Medline, Google Scholar
36. Andreasen NC, O’Leary DS, Arndt S, Cizadlo T, Hurtig R, Rezai K, Watkins GL, Boles Ponto LL, Hichwa RD: Short-term and long-term verbal memory: a positron emission tomography study. Proc Natl Acad Sci USA 1995; 92:5111-5115Crossref, Medline, Google Scholar
37. Henson RNA, Rugg MD, Shallice T, Josephs O, Dolan RJ: Recollection and familiarity in recognition memory: an event-related functional magnetic resonance imaging study. J Neurosci 1999; 19:3962-3972Crossref, Medline, Google Scholar
38. Kim JJ, Andreasen NC, O’Leary DS, Wiser AK, Boles Ponto LL, Watkins GL, Hichwa RD: Direct comparison of the neural substrates of recognition memory for words and faces. Brain 1999; 122:1069-1083Crossref, Medline, Google Scholar
39. Petrides M, Alivisatos B, Meyer E, Evans AC: Functional activation of the human frontal cortex during the performance of verbal working memory tasks. Proc Natl Acad Sci USA 1993; 90:878-882Crossref, Medline, Google Scholar
40. Berman KF, Zec RF, Weinberger DR: Physiologic dysfunction of dorsolateral prefrontal cortex in schizophrenia, II: role of neuroleptic treatment, attention, and mental effort. Arch Gen Psychiatry 1986; 43:126-135Crossref, Medline, Google Scholar
41. D’Esposito M, Detre JA, Alsop DC, Shin RK, Atlas S, Grossman M: The neural basis of the central executive system of working memory. Nature 1995; 378:279-281Crossref, Medline, Google Scholar
42. Fiez JA, Raichle ME, Balota DA, Tallal P, Petersen LE: PET activation of posterior temporal regions during passive auditory word presentation and verb generation. Cereb Cortex 1996; 6:1-10Crossref, Medline, Google Scholar
43. Price CJ, Moore CJ, Humphreys GW, Wise RJS: Segregating semantic from phonological processes during reading. J Cogn Neurosci 1997; 9:727-733Crossref, Medline, Google Scholar
44. Goldman-Rakic PS: Topography of cognition: parallel distributed networks in primate association cortex. Annu Rev Neurosci 1988; 11:137-156Crossref, Medline, Google Scholar
45. Seidman LJ, Goldstein JM, Goodman JM, Koren D, Turner WM, Faraone SV, Tsuang MT: Sex differences in olfactory identification and Wisconsin Card Sorting performance in schizophrenia: relationship to attention and verbal ability. Biol Psychiatry 1997; 42:104-115Crossref, Medline, Google Scholar
46. Albus M, Hubmann W, Mohr F, Scherer J, Sobizack N, Franz U, Hecht S, Borrmann M, Wahlheim C: Are there gender differences in neuropsychological performance in patients with first-episode schizophrenia? Schizophr Bull 1997; 28:39-50Crossref, Google Scholar
47. Scottish Schizophrenia Research Group: The Scottish First Episode Schizophrenia Study. III: cognitive performance. Br J Psychiatry 1987; 150:338-340Crossref, Medline, Google Scholar
48. Andia AM, Zisook S, Heaton RK, Hesselink J, Jernigan T, Kuck J, Morganville J, Braff DL: Gender differences in schizophrenia. J Nerv Ment Dis 1995; 183:522-528Crossref, Medline, Google Scholar
49. Perlick D, Mattis S, Stastny P, Teresi J: Gender differences in cognition in schizophrenia. Schizophr Res 1992; 8:69-73Crossref, Medline, Google Scholar
50. Lewine RRJ, Walker EF, Shurett R, Caudle J, Haden C: Sex differences in neuropsychological functioning among schizophrenic patients. Am J Psychiatry 1996; 153:1178-1184Link, Google Scholar
51. Weiss EM, Bilder RM, Fleischhacker WW: The effects of second-generation antipsychotics on cognitive functioning and psychosocial outcome in schizophrenia. Psychopharmacology (Berl) 2002; 162:11-17Crossref, Medline, Google Scholar
52. Goldberg TE, Weinberger DR: Effects of neuroleptic medications on the cognition of patients with schizophrenia: a review of recent studies. J Clin Psychiatry 1996; 57(suppl 9):62-65Medline, Google Scholar
53. Posner MI, Petersen SE, Fox PT, Raichle ME: Localization of cognitive operations in the human brain. Science 1988; 240:1627-1631Crossref, Medline, Google Scholar
54. Friston KJ, Price CJ, Fletcher P, Moore C, Frackowiak RSJ, Dolan RJ: The trouble with cognitive subtraction. Neuroimage 1996; 4:97-104Crossref, Medline, Google Scholar