Uncinate Fasciculus Findings in Schizophrenia: A Magnetic Resonance Diffusion Tensor Imaging Study
Abstract
OBJECTIVE: Disruptions in connectivity between the frontal and temporal lobes may explain some of the symptoms observed in schizophrenia. Conventional magnetic resonance imaging (MRI) studies, however, have not shown compelling evidence for white matter abnormalities, because white matter fiber tracts cannot be visualized by conventional MRI. Diffusion tensor imaging is a relatively new technique that can detect subtle white matter abnormalities in vivo by assessing the degree to which directionally organized fibers have lost their normal integrity. The first three diffusion tensor imaging studies in schizophrenia showed lower anisotropic diffusion, relative to comparison subjects, in whole-brain white matter, prefrontal and temporal white matter, and the corpus callosum, respectively. Here the authors focus on fiber tracts forming temporal-frontal connections. METHOD: Anisotropic diffusion was assessed in the uncinate fasciculus, the most prominent white matter tract connecting temporal and frontal brain regions, in 15 patients with chronic schizophrenia and 18 normal comparison subjects. A 1.5-T GE Echospeed system was used to acquire 4-mm-thick coronal line-scan diffusion tensor images. Maps of the fractional anisotropy were generated to quantify the water diffusion within the uncinate fasciculus. RESULTS: Findings revealed a group-by-side interaction for fractional anisotropy and for uncinate fasciculus area, derived from automatic segmentation. The patients with schizophrenia showed a lack of normal left-greater-than-right asymmetry seen in the comparison subjects. CONCLUSIONS: These findings demonstrate the importance of investigating white matter tracts in vivo in schizophrenia and support the hypothesis of a disruption in the normal pattern of connectivity between temporal and frontal brain regions in schizophrenia.
Evidence suggests that a disruption in connectivity between different brain regions, especially between the frontal and temporal lobes, may partly explain some of the primary symptoms of schizophrenia (e.g., references 1–3). This idea, first proposed by Wernicke in 1906 (4), posits a disturbance in functional connectivity between the frontal and temporal cortices in schizophrenia that might have as its basis a disruption in the white matter tracts connecting them.
This speculation has attracted recent attention because of functional, anatomical, and neuropathological reports of abnormalities in both frontal and temporal brain regions. For example, many in vivo magnetic resonance imaging (MRI) studies have shown volume deficits in frontal and temporal gray matter (5–7), although neuropathologic studies show more mixed results, with some showing abnormalities in the number and/or density of cells in various prefrontal regions (e.g., references 8, 9) and others not showing brain abnormalities in these regions (e.g., reference 10). There is also evidence from studies of regional cerebral blood flow that functional connectivity between these two brain regions might be abnormal in schizophrenia (11, 12). These latter studies have demonstrated that patients with schizophrenia have a failure of deactivation in the temporal lobes that is normally associated with activation of the dorsolateral prefrontal cortex during a verbal fluency task (finding replicated in a functional MRI study [13] but not replicated by the same authors in a different study group [14]). Some investigators (11, 15) have also tried to characterize the nature of the disruptions in connectivity by attributing them to late stages of myelination. Moreover, Deakin and Simpson (2) hypothesized that genetically transmitted abnormalities in the development of the ventral frontal lobe may lead to a progressive degeneration of its projections coursing through the uncinate fasciculus to the temporal lobe. Finally, MRI structural studies (16, 17) have shown an association between volume reduction of the prefrontal cortex and temporal lobe in schizophrenia.
There is, however, a lack of direct in vivo evidence of abnormal connectivity between the frontal and temporal brain regions in schizophrenia as evinced by abnormalities in the fiber bundles connecting the two regions. This is not surprising given that conventional MRI studies are not suitable for visualizing fiber tracts. In contrast, diffusion tensor imaging (18) is an MRI technique that can detect subtle white matter abnormalities in vivo by assessing the degree to which directionally organized tissues have lost their normal integrity. This technique is based on sensitizing the MR signal to the movement of water and determining the magnitude and direction of the water diffusion in three dimensions. In white matter, diffusion perpendicular to the direction of axons is restricted by myelin sheath and cell membrane such that diffusion is greater along the length of the axon than perpendicular to it. In contrast, in CSF and gray matter, water diffusion is random and equal in all directions. This characteristic of the diffusion (differing according to the medium’s relative orientation) is termed “anisotropy.” The estimation of the diffusion tensor, a mathematical entity that characterizes water diffusion in all directions, has been proposed as an effective measure of diffusion in anisotropic tissue. In addition, several scalar measures describing the extent to which diffusion is anisotropic, indicating the presence of directional bias of the diffusion, have been proposed. The most popular are fractional anisotropy and relative anisotropy indices (19); the fractional anisotropy index has a slightly better signal-to-noise ratio than the relative anisotropy index (20). Higher anisotropy indices indicate greater directionality and coherence of the fiber tracts, while lower anisotropy indicates less directionality and more random movement of water in all directions measured.
This method has been used to discern the directionality of white matter tracts in the normal human brain (21) and to evaluate white matter fiber integrity in multiple sclerosis (22), stroke (23), and Alzheimer’s disease (24). Of note, the first three studies to quantify anisotropic diffusion in patients with schizophrenia showed lower anisotropy within prefrontal white matter (25), in whole-brain white matter (26), and in the splenium of the corpus callosum (27) than in comparison subjects. None of these studies, however, focused on specific temporal-frontal fiber tracts in schizophrenia, as proposed here. In this study, we applied line-scan diffusion tensor imaging (28) to obtain fractional anisotropy maps for 15 patients with schizophrenia and 18 comparison subjects in order to measure the integrity of fibers within the uncinate fasciculus, the most prominent of all white matter fiber tracts connecting the frontal and temporal lobes.
Method
Subjects
Fifteen patients with schizophrenia were recruited from inpatient, day treatment, outpatient, and foster care programs at the VA Boston Healthcare System, Brockton, Mass. Assessments with the Structured Clinical Interview for DSM-IV—Patient Version (SCID) were used to make DSM-IV diagnoses, and the nonpatient edition of the SCID was completed for 18 normal comparison subjects.
The comparison subjects were recruited from the general community and were group-matched to the patients on age, sex, handedness (29), and parental socioeconomic status (30).
The inclusion criteria for all subjects were right-handedness, age between 17 and 55 years, no history of electroconvulsive shock treatment, no history of neurological illness, no alcohol or drug abuse in the last 5 years, no medication with known effects on MR (such as steroids), a verbal IQ above 75, and an ability and desire to cooperate with the procedures as evidenced by written informed consent. In addition, the comparison subjects were screened to exclude individuals who had a first-degree relative with an axis I disorder.
Clinical Measures
As part of a comprehensive neuropsychological battery, all subjects were evaluated by means of the verbal paired associate learning subtest of the Wechsler Memory Scale—3rd ed. (WMS) (31), the Wisconsin Card Sorting Test (32), the Trail Making Test, and the similarities subtest of the WAIS-III (33). This subset of neuropsychological tests was selected because in prior studies in our laboratory (32), poor performance on them was correlated with reduced volume of either temporal or frontal lobe regions, as assessed by conventional MRI, in patients with schizophrenia. We used these tests to assess the functional significance of the uncinate fasciculus in patients with schizophrenia.
MRI Protocol
All subjects were scanned by means of line-scan diffusion tensor imaging, a technique that can be implemented on conventional MR scanners (28). Unlike the single-shot (34) and navigated echo (35) echo-planar imaging, the most commonly used MR diffusion imaging techniques, by which a whole volume or whole slice is acquired at once, line-scan diffusion tensor imaging is composed of a series of parallel columns lying in the image plane. The sequential collection of these line data in independent acquisitions makes the sequence largely insensitive to bulk motion artifact since no phase encoding is used and shot-to-shot phase variations are fully removed by calculating the magnitude of the signal. In addition, the existing movement artifacts can be easily removed, since interpolation is much easier for a missing line than for a missing slice or volume.
MR scans were performed with a quadrature head coil on a 1.5-T GE Echospeed system (General Electric Medical Systems, Milwaukee), which permits maximum gradient amplitudes of 40 mT/meter. We began with a set of three orthogonal T1-weighted images used as localizers: sagittal, axial aligned to the anterior commissure–posterior commissure (AC-PC) line, and another sagittal aligned to the interhemispheric fissure. From the last sagittal T1-weighted image, the line-scan diffusion tensor imaging sequence in the coronal orientation was then aligned to the AC-PC line. For each line, six images with high diffusion weighting (1000 sec/mm2) along six directions were collected. For low diffusion weighting (5 sec/mm2) we collected only two images, since diffusion-related signal changes are minimal. The following scan characteristics were used: rectangular field of view, 220×165 mm; 128×128 scan matrix (256×256 image matrix); slice thickness, 4 mm; interslice distance, 1 mm; receiver bandwidth, ±4 kHz; TE (echo time), 64 msec; effective TR (repetition time), 2592 msec; scan time, 60 sec/slice section. We acquired a total of 31–35 coronal slices covering the entire brain, depending on brain size. The total scan time was 31–35 minutes.
After reconstruction, the diffusion-weighted images were transferred to a workstation, where eigenvalue, eigenvector, and fractional anisotropy maps of the diffusion tensor were calculated. Motion-related artifact maps were also constructed.
Quantification and Statistical Analysis
To quantify uncinate fasciculus diffusion, we used one coronal slice, perpendicular to the AC-PC line, that intersects the uncinate fasciculus in the temporal stem—the densest portion of this fiber tract (Figure 1 and Figure 2). The uncinate fasciculus in this location is parallel to the AC-PC line and appears visible on only one slice in the segmentation defined by the maximum diffusivity (λ1, the largest eigenvalue of the diffusion tensor) (Figure 3). All measurements were done by one of us (M.K.) while blind to diagnostic group.
For each subject, a point centered within each fiber tract (separately for left and right) was selected, and fractional anisotropy (which describes deviation from the isotropic diffusion) was calculated by using the following formula (19):
As we were interested in the extent to which anisotropy differences between groups reflect the degree of connectivity between the frontal and temporal lobes, we also measured the area of the uncinate fasciculus, derived from the maximum diffusivity segmentation (1×10–3 mm2/sec was used as the fixed threshold for all the cases; greater area in the presence of greater anisotropy should represent more interconnecting fiber tracts), and we measured the sum of fractional anisotropy for the entire area. In addition, as the manual selection of a point could potentially bias the measurement, the point with the maximal fractional anisotropy value within the uncinate fasciculus was also automatically calculated.
To examine motion artifact, we calculated the degree of motion-related artifacts within the segmented region of interest. This measure was defined as the number of lines missing in the raw line-scan diffusion tensor imaging data (six directed diffusion images).
Repeated measures analysis of variance (ANOVA), with group as a between-subjects factor and side as a within-subjects factor, were used to test for group differences in uncinate fasciculus diffusion. In the case of a significant group-by-side interaction, independent t tests were used to compare group differences, separately for the right and left hemispheres, and paired t tests were used within each group to test for hemispheric asymmetry differences in diffusion. Associations between neuropsychological test results and diffusion measures were evaluated by using Spearman rank-order correlations. Because our correlations were hypothesis driven, we did not correct for multiple comparisons; however, we conservatively considered only p values below 0.01 (two-tailed) as significant.
Results
Demographic Data
There were no group differences in age, handedness, or gender (Table 1). The groups differed in education and socioeconomic status but not in parental socioeconomic status. All of the patients with schizophrenia were receiving medication; none of the diffusion measures was correlated with medication dose.
Measures of Uncinate Fasciculus Anisotropy
Movement artifacts were detected within the region of interest in only two patients. As the algorithm for analyzing diffusion data replaces the missing lines with interpolated data from the neighboring voxels, and because, in both cases, only one of eight lines for a given voxel was missing, we believe that none of the measures was affected. Nevertheless, we analyzed our data set with and without these subjects, and we found no differences in results.
The patient and comparison groups did not differ in fractional anisotropy (F=0.01, df=1, 31, p=0.92). However, there was a significant group-by-side interaction (F=9.25, df=1, 31, p=0.005) (Figure 4). The same results were achieved with automatic detection of the measured voxel. There was also a group-by-side interaction in measured area (Figure 4), as derived from the directional diffusion threshold (F=4.15, df=1, 31, p=0.05), and in the total fractional anisotropy over the segmented area (F=4.99, df=1, 31, p=0.04) (Figure 4). Follow-up t tests revealed no significant differences between groups on any of the three measurements (fractional anisotropy, area, total fractional anisotropy) on either the left (t=1.33, df=31, p=0.19; t=1.13, df=31, p=0.26; t=1.14, df=31, p=0.27) or the right (t=0.17, df=31, p=0.09; t=0.97, df=31, p=0.34; t=0.95, df=31, p=0.36). However, t tests comparing the left and right hemispheres in each group revealed that the normal comparison group showed an asymmetry of the fractional anisotropy (left greater than right) for each measurement (t=3.32, df=17, p=0.004; t=2.20, df=17, p=0.05; t=3.11, df=17, p=0.006) that was absent in the schizophrenia group (t=0.89, df=14, p=0.38; t=0.81, df=14, p=0.43; t=0.13, df=14, p=0.90).
Correlations of Uncinate Fasciculi Anisotropy to Clinical and Cognitive Measures
For our measure of visual attention, lower fractional anisotropy of the right uncinate fasciculus correlated significantly with a higher number of errors on the Trail Making Test (r=–0.71, df=13, p<0.01) for the patients only. Similarly, for the patients only, lower fractional anisotropy of the right uncinate fasciculus correlated significantly with a lower score on the similarities subtest of the WAIS-III (r=0.55, df=13, p<0.05), a measure of verbal abstraction and categorization. For the verbal paired associate learning subtest of the WMS, worse immediate recall of word pairs correlated significantly with lower fractional anisotropy of the left uncinate fasciculus (r=0.79, df=9, p<0.01) for the patients only. In contrast, performance on the Wisconsin Card Sorting Test, as assessed by the number of categories achieved, did not correlate significantly with either right or left fractional anisotropy of the uncinate fasciculus (right: r=–0.05, df=12, p=0.87; left: r=–0.28, df=12, p=0.35).
Discussion
We here report an asymmetry (left greater than right) in uncinate fasciculus anisotropic diffusion in normal male subjects, which was not evident in male patients with chronic schizophrenia. This result was obtained by measuring fractional anisotropy within the uncinate fasciculus, and it was confirmed by measures of the area of the uncinate fasciculus and by the sum of fractional anisotropy for the entire area, derived from an automated segmentation. To our knowledge, this result has not been previously reported in the literature.
The asymmetry of uncinate fasciculus diffusion anisotropy in the comparison subjects may reflect structural and functional differences between the two hemispheres that are neurodevelopmental in origin. Such asymmetries have been documented in several brain regions, including the Sylvian fissure (37), planum temporale (38), and frontal operculum (37), during the second and third trimesters of gestation. Many of these asymmetry differences may be relevant to specialized functions of the brain, which are lateralized in humans, such as language (e.g., references 39, 40). The greater anisotropy found in the left than in the right uncinate fasciculus in normal subjects in our study may indicate a higher number and/or density of fibers in the left uncinate fasciculus than in that on the right.
The uncinate fasciculus is the largest of the three big fiber bundles connecting the frontal and temporal lobes; the other two are the cingulate and the superior longitudinal fasciculus. The absence of asymmetry in schizophrenia suggests a significant abnormality in the integrity of the fiber tracts connecting the frontal and temporal lobes. Not known, however, is how this lack of normal asymmetry might be understood vis-à-vis the pathophysiology of schizophrenia. For example, abnormalities in anisotropy within white matter might be explained by abnormalities in the number and/or density of the interconnecting fiber tracts or by abnormalities in myelination (41). In addition, differences in anisotropy between schizophrenia patients and comparison subjects might be explained by a lack of, or a loss of, coherence of white matter fiber bundles traveling between two distinct brain regions, affecting the connectivity between these regions. Thus, an abnormality in one or in a combination of these features might be manifest in a loss of asymmetry, as we report here in schizophrenia.
There are several candidate pathological processes that might influence the integrity of white matter fiber connections between frontal and temporal regions. For example, Akbarian et al. (42) reported an abnormal distribution of interstitial neurons in prefrontal and temporal regions in schizophrenia, which they attributed to the disruption of fetal brain development and failure of neuron migration during the second trimester of pregnancy, thereby affecting the ingrowth of connections to the cortex. The higher number of neurons in schizophrenia might therefore adversely affect the number and organization of their possible axonal projections and, consequently, water diffusion within the white matter tracts, as measured by diffusion tensor imaging.
In addition, Deakin and Simpson (2) suggested that neurochemical, histological, and functional abnormalities in schizophrenia most likely reflect progressive degeneration of projections from the ventral frontal to the anterior temporal lobe. They suggested that dysplastic cellular architecture in the ventral frontal cortex gives rise to projections to the temporal cortex, which are vulnerable, as a consequence of their dysplastic origins, to some extrinsic and intrinsic pathological processes. These speculations, in conjunction with their report of left-lateralized glutamate uptake sites in the polar temporal cortex of patients with schizophrenia (43), are consistent with our finding of changes in uncinate fasciculus diffusion anisotropy in schizophrenia.
The results reported here are also consonant with MRI (44) and postmortem (45) findings suggesting an absence of normal brain asymmetry in schizophrenia. According to Crow et al. (46), who posited a “lateralization hypothesis of schizophrenia,” abnormal neural development of brain lateralization is critical to the etiology of schizophrenia. They stated that the left hemisphere may be more vulnerable to insult or damage because it develops later than the right hemisphere (see also reference 39) and that this damage might occur in schizophrenia. If such damage occurs in white matter, it might affect white matter fiber tracts as measured by diffusion tensor imaging.
Differences in brain development are also the focal point of work by Benes and co-workers (47), who suggested that myelination in specific brain regions (especially the frontal and temporal lobes, where myelination still occurs in the second decade of life) might play a neuroprotective role, particularly with respect to the timing of the appearance of symptoms in individuals at risk for schizophrenia. In addition, in a study using magnetization transfer contrast imaging, which measures the myelin component of white matter (48), the myelin component of fibers in the temporal lobe was lower in patients with schizophrenia. Thus, it is possible that schizophrenia patients have an abnormality of myelin. As myelin sheaths form linear structures surrounding and insulating axons, their integrity and thickness influence the water diffusion within the brain, and measures of diffusion might reflect such alterations (41).
Other diffusion tensor imaging studies have also shown anisotropy abnormalities in schizophrenia. Lim and co-workers (26), for example, using a measure of fractional anisotropy averaged over large regions of interest, reported widespread lower anisotropy in patients with schizophrenia than in normal subjects. Buchsbaum et al. (1), using methods based on statistical probability maps, reported lower relative anisotropy (another index of anisotropy with a slightly different scaling parameter) within the left prefrontal white matter region in schizophrenia. Finally, Foong et al. (27) reported lower anisotropy within the splenium but not genu of the corpus callosum in schizophrenia patients than in comparison subjects, determined by using small region-of-interest averaging techniques and fractional anisotropy.
These findings, taken together, provide strong evidence for loss of integrity within the white matter fiber tracts in schizophrenia. The question remains, however, as to whether it is the same widespread pathological process affecting the whole white matter or whether the process is more localized, affecting several white matter fiber tracts. A further question is whether the anomaly is primary and due to loss of gray matter volume or secondary to the regional abnormalities within gray matter areas interconnected by the fiber tract, as several MR structural studies have shown abnormalities of orbitofrontal and temporal pole volume in schizophrenia, specifically reduced volume in patients with chronic schizophrenia (49) and increased volume on the right side in subjects with first-episode schizophrenia (50) in the orbital frontal region and volume reduction within the temporal pole in schizophrenia subjects (7, 51) when compared with normal comparison subjects.
With respect to correlations with clinical measures, the diffusion tensor imaging measures correlated in expected ways with neuropsychological measures in the patients with schizophrenia. That is, poor performance on a measure of visual attention correlated with right-sided white matter abnormalities, whereas poor verbal associative memory correlated with left-sided white matter abnormalities. These correlations between neuropsychological performance and measures of connectivity suggest the presence of a disruption in frontal-temporal functional connectivity.
A possible limitation of our study is the small number of subjects, although, to date, most diffusion studies in schizophrenia have been based on relatively small study groups. In addition, our study suggests that motion artifact in diffusion tensor imaging studies may be an issue. Although line-scan diffusion tensor imaging is less sensitive to movement artifacts than is echo-planar imaging (28), one might still want to examine motion artifacts as a potential confound.
While the expected direction of the fiber tracts in the uncinate fasciculus is anterior-posterior, fibers traveling in other directions might decrease the diffusion anisotropy within our region of interest. Hanyu et al. (24), who calculated anisotropy diffusion within the temporal stem in patients with Alzheimer’s disease, noted that fiber bundles crossing the anterior commissure might be an additional source of alterations in diffusion anisotropy within the anterior temporal region. Analogously, it is possible that other fibers crossing the uncinate fasciculus within our region of interest could decrease anisotropy findings (19), although we would expect to find this effect in both groups equally.
In summary, we believe that this study presents new findings relevant to the status of white matter tracts in schizophrenia. We were able to evaluate directly in vivo fiber tracts of the uncinate fasciculus, a bundle of fibers that might play an important role in the pathology of this disorder. Our study revealed disruptions in connectivity between the frontal and temporal lobes that were marked by a significant loss of normal left-greater-than-right asymmetry in patients with schizophrenia. Further studies are needed to determine the exact functional role of the uncinate fasciculus and the implications of abnormalities in this white matter fiber tract in schizophrenia.
![]() |
Presented in part at the 153rd annual meeting of the American Psychiatric Association, Chicago, May 13–18, 2000. Received June 8, 2001; revision received Nov. 8, 2001; accepted Nov. 19, 2001. From the Clinical Neuroscience Division, Laboratory of Neuroscience, Department of Psychiatry, Harvard Medical School and VA Boston Healthcare System—Brockton Division; and the Surgical Planning Laboratory, MRI Division, Department of Radiology, Brigham and Women’s Hospital and Harvard Medical School, Boston. Address reprint requests to Dr. Shenton or Dr. McCarley, Department of Psychiatry–116A, VA Boston Healthcare System—Brockton Division, 940 Belmont St., Brockton, MA 02301; [email protected], [email protected] (e-mail). Supported by a Kosciuszko Foundation Research Award (Dr. Kubicki), by a Young Investigator Award from the National Alliance for Research on Schizophrenia and Depression (Dr. Kubicki), by NIMH grants MH-01110 and MH-50747 (Dr. Shenton) and MH-40799 (Dr. McCarley), by VA Merit Awards (Drs. Shenton and McCarley), by NIH National Center for Research Resources grants RR-11747 (Dr. Kikinis) and RR-13218 (Drs. Jolesz and Westin), by the Whitaker Foundation (Dr. Maier), and by a VA Psychiatry/Neuroscience Research Fellowship Award (Dr. Frumin). The authors thank Marie Fairbanks for administrative assistance and Christopher Allen for recruiting subjects and conducting interviews with the normal comparison subjects.
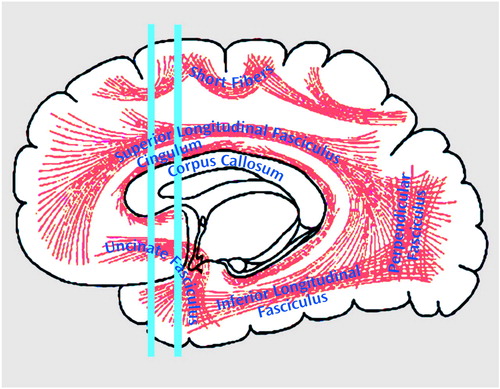
Figure 1. Approximate Location of the Brain Slice Selected for Measurement of Anisotropic Water Diffusion in the Uncinate Fasciculus in Patients With Chronic Schizophreniaa
aThe posterior line intersects the uncinate fasciculus in the densest portion, perpendicular to the plane (thus it was selected for the measurements), whereas the anterior line intersects the fewest number of fibers perpendicular to the plane. Adapted from Gray’s Anatomy of the Human Body as displayed at Bartleby.com (36).
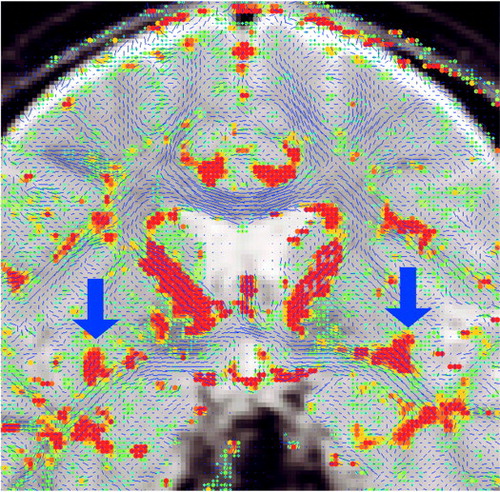
Figure 2. Diffusion Tensor Map for a Selected Coronal Plane in a Normal Comparison Subject, Showing the Directionality of the White Matter Tractsa
aThe blue lines indicate the in-plane diffusion, and the other colors indicate the magnitude of the out-of-plane diffusion, with red-orange indicating the maximal diffusion. Note the clear appearance of the uncinate fasciculi (orange-red) bilaterally within the temporal stem (see arrows).
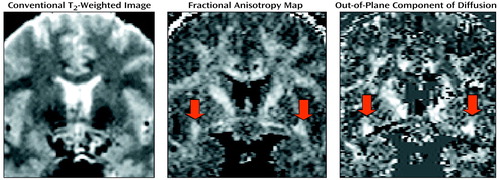
Figure 3. Conventional T2-Weighted Image, Fractional Anisotropya Map, and Out-of-Plane Diffusion Map for a Selected Coronal Plane in a Normal Comparison Subjectb
aDeviation from the isotropic diffusion.
bThe conventional image does not show white matter fiber tracts in the brain. The fractional anisotropy map highlights the white matter bundles in the brain, with the red arrows pointing to the uncinate fasciculi. The image on the right displays the out-of-plane component of the diffusion; high intensity corresponds to the fiber tracts perpendicular to the plane, thus differentiating the uncinate fasciculi from the fiber tracts with other directions (again, red arrows point to uncinate fasciculi).
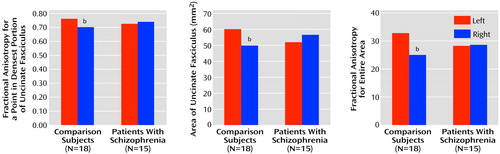
Figure 4. Mean Values of Patients With Chronic Schizophrenia and Normal Comparison Subjects for Measures of Fractional Anisotropya in the Uncinate Fasciculus, Reflecting White Matter Integrity
aDeviation from the isotropic diffusion.
bSignificant group-by-side interaction (ANOVA, p≤0.05) and significant asymmetry in the comparison subjects (t test, p<0.05).
1. Friston KJ, Frith CD: Schizophrenia: a disconnection syndrome? Clin Neurosci 1995; 3:89-97Medline, Google Scholar
2. Deakin JF, Simpson MD: A two-process theory of schizophrenia: evidence from studies in post-mortem brain. J Psychiatr Res 1997; 31:277-295Crossref, Medline, Google Scholar
3. McGuire PK, Frith CD: Disordered functional connectivity in schizophrenia (editorial). Psychol Med 1996; 26:663-667Crossref, Medline, Google Scholar
4. Wernicke C: Grundrisse der Psychiatrie. Leipzig, Thieme, 1906Google Scholar
5. Buchanan RW, Vladar K, Barta PE, Pearlson GD: Structural evaluation of the prefrontal cortex in schizophrenia. Am J Psychiatry 1998; 155:1049-1055Link, Google Scholar
6. Shenton ME, Dickey CC, Frumin M, McCarley RW: A review of MRI findings in schizophrenia. Schizophr Res 2001; 49:1-52Crossref, Medline, Google Scholar
7. Gur RE, Turetsky BI, Cowell PE, Finkelman C, Maany V, Grossman RI, Arnold SE, Bilker WB, Gur RC: Temporolimbic volume reductions in schizophrenia. Arch Gen Psychiatry 2000; 57:769-775Crossref, Medline, Google Scholar
8. Benes FM, Vincent SL, Alsterberg G, Bird ED, SanGiovanni JP: Increased GABAA receptor binding in superficial layers of cingulate cortex in schizophrenics. J Neurosci 1992; 12:924-929Crossref, Medline, Google Scholar
9. Selemon LD, Rajkowska G, Goldman-Rakic PS: Abnormally high neuronal density in the schizophrenic cortex: a morphometric analysis of prefrontal area 9 and occipital area 17. Arch Gen Psychiatry 1995; 52:805-818; discussion 52:819-820Crossref, Medline, Google Scholar
10. Highley JR, Walker MA, Esiri MM, McDonald B, Harrison PJ, Crow TJ: Schizophrenia and the frontal lobes: post-mortem stereological study of tissue volume. Br J Psychiatry 2001; 178:337-343Crossref, Medline, Google Scholar
11. Weinberger DR, Berman KF, Suddath R, Torrey EF: Evidence of dysfunction of a prefrontal-limbic network in schizophrenia: a magnetic resonance imaging and regional cerebral blood flow study of discordant monozygotic twins. Am J Psychiatry 1992; 149:890-897Link, Google Scholar
12. Frith CD, Friston KJ, Herold S, Silbersweig D, Fletcher P, Cahill C, Dolan RJ, Frackowiak RS, Liddle PF: Regional brain activity in chronic schizophrenic patients during the performance of a verbal fluency task. Br J Psychiatry 1995; 167:343-349Crossref, Medline, Google Scholar
13. Yurgelun-Todd DA, Waternaux CM, Cohen BM, Gruber SA, English CD, Renshaw PF: Functional magnetic resonance imaging of schizophrenic patients and comparison subjects during word production. Am J Psychiatry 1996; 153:200-205Link, Google Scholar
14. Spence SA, Liddle PF, Stefan MD, Hellewell JS, Sharma T, Friston KJ, Hirsch SR, Frith CD, Murray RM, Deakin JF, Grasby PM: Functional anatomy of verbal fluency in people with schizophrenia and those at genetic risk: focal dysfunction and distributed disconnectivity reappraised. Br J Psychiatry 2000; 176:52-60Crossref, Medline, Google Scholar
15. Randall PL: Schizophrenia, abnormal connection, and brain evolution. Med Hypotheses 1983; 10:247-280Crossref, Medline, Google Scholar
16. Wible CG, Shenton ME, Hokama H, Kikinis R, Jolesz FA, Metcalf D, McCarley RW: Prefrontal cortex and schizophrenia: a quantitative magnetic resonance imaging study. Arch Gen Psychiatry 1995; 52:279-288Crossref, Medline, Google Scholar
17. Woodruff PW, Wright IC, Shuriquie N, Russouw H, Rushe T, Howard RJ, Graves M, Bullmore ET, Murray RM: Structural brain abnormalities in male schizophrenics reflect fronto-temporal dissociation. Psychol Med 1997; 27:1257-1266Crossref, Medline, Google Scholar
18. Basser PJ, Mattiello J, LeBihan D: MR diffusion tensor spectroscopy and imaging. Biophys J 1994; 66:259-267Crossref, Medline, Google Scholar
19. Basser PJ, Pierpaoli C: Microstructural and physiological features of tissues elucidated by quantitative-diffusion-tensor MRI. J Magn Reson B 1996; 111:209-219Crossref, Medline, Google Scholar
20. Papadakis NG, Xing D, Houston GC, Smith JM, Smith MI, James MF, Parsons AA, Huang CL, Hall LD, Carpenter TA: A study of rotationally invariant and symmetric indices of diffusion anisotropy. Magn Reson Imaging 1999; 17:881-892Crossref, Medline, Google Scholar
21. Pierpaoli C, Jezzard P, Basser PJ, Barnett A, Di Chiro G: Diffusion tensor MR imaging of the human brain. Radiology 1996; 201:637-648Crossref, Medline, Google Scholar
22. Filippi M, Cercignani M, Inglese M, Horsfield MA, Comi G: Diffusion tensor magnetic resonance imaging in multiple sclerosis. Neurology 2001; 56:304-311Crossref, Medline, Google Scholar
23. Zelaya F, Flood N, Chalk JB, Wang D, Doddrell DM, Strugnell W, Benson M, Ostergaard L, Semple J, Eagle S: An evaluation of the time dependence of the anisotropy of the water diffusion tensor in acute human ischemia. Magn Reson Imaging 1999; 17:331-348Crossref, Medline, Google Scholar
24. Hanyu H, Imon Y, Sakurai H, Iwamoto T, Takasaki M, Shindo H, Kakizaki D, Abe K: Regional differences in diffusion abnormality in cerebral white matter lesions in patients with vascular dementia of the Binswanger type and Alzheimer’s disease. Eur J Neurol 1999; 6:195-203Crossref, Medline, Google Scholar
25. Buchsbaum MS, Tang CY, Peled S, Gudbjartsson H, Lu D, Hazlett EA, Downhill J, Haznedar M, Fallon JH, Atlas SW: MRI white matter diffusion anisotropy and PET metabolic rate in schizophrenia. Neuroreport 1998; 9:425-430Crossref, Medline, Google Scholar
26. Lim KO, Hedehus M, Moseley M, de Crespigny A, Sullivan EV, Pfefferbaum A: Compromised white matter tract integrity in schizophrenia inferred from diffusion tensor imaging. Arch Gen Psychiatry 1999; 56:367-374Crossref, Medline, Google Scholar
27. Foong J, Maier M, Clark CA, Barker GJ, Miller DH, Ron MA: Neuropathological abnormalities of the corpus callosum in schizophrenia: a diffusion tensor imaging study. J Neurol Neurosurg Psychiatry 2000; 68:242-244Crossref, Medline, Google Scholar
28. Gudbartsson H, Maier S, Mulkern R: Line scan diffusion imaging. Magn Reson Med 1996; 36:509-519Crossref, Medline, Google Scholar
29. Oldfield RC: The assessment and analysis of handedness: the Edinburgh Inventory. Neuropsychologia 1971; 9:97-113Crossref, Medline, Google Scholar
30. Hollingshead AB: Two-Factor Index of Social Position. New Haven, Conn, Yale University, 1965Google Scholar
31. Wechsler D: The Wechsler Memory Scale—3rd ed. San Antonio, Tex, Psychological Corp (Harcourt), 1997Google Scholar
32. Nestor PG, Shenton ME, Wible C, Hokama H, O’Donnell BF, Law S, McCarley RW: A neuropsychological analysis of schizophrenic thought disorder. Schizophr Res 1998; 29:217-225Crossref, Medline, Google Scholar
33. Wechsler D: Wechsler Adult Intelligence Scale—3rd ed. San Antonio, Tex, Psychological Corp (Harcourt), 1997Google Scholar
34. Turner R, von Kienlin M, Moonen CT, van Zijl PC: Single-shot localized echo-planar imaging (STEAM-EPI) at 4.7 tesla. Magn Reson Med 1990; 14:401-408Crossref, Medline, Google Scholar
35. Ordidge RJ, Helpern JA, Qing ZX, Knight RA, Nagesh V: Correction of motional artifacts in diffusion-weighted MR images using navigator echoes. Magn Reson Imaging 1994; 12:455-460Crossref, Medline, Google Scholar
36. Gray H: Anatomy of the Human Body (1918), 20th ed. Bartleby. com Great Books Online. http://bartleby.com/107/illus751.htmlGoogle Scholar
37. Chi JG, Dooling EC, Gilles FH: Gyral development of the human brain. Ann Neurol 1977; 1:86-93Crossref, Medline, Google Scholar
38. Wada JA, Clarke R, Hamm A: Cerebral hemispheric asymmetry in humans: cortical speech zones in 100 adults and 100 infant brains. Arch Neurol 1975; 32:239-246Crossref, Medline, Google Scholar
39. Galaburda AM, Sanides F, Geschwind N: Human brain: cytoarchitectonic left-right asymmetries in the temporal speech region. Arch Neurol 1978; 35:812-817Crossref, Medline, Google Scholar
40. Chi JG, Dooling EC, Gilles FH: Left-right asymmetries of the temporal speech areas of the human fetus. Arch Neurol 1977; 34:346-348Crossref, Medline, Google Scholar
41. Beaulieu C, Allen PS: Determinants of anisotropic water diffusion in nerves. Magn Reson Med 1994; 31:394-400Crossref, Medline, Google Scholar
42. Akbarian S, Kim JJ, Potkin SG, Hetrick WP, Bunney WE Jr, Jones EG: Maldistribution of interstitial neurons in prefrontal white matter of the brains of schizophrenic patients. Arch Gen Psychiatry 1996; 53:425-436Crossref, Medline, Google Scholar
43. Deakin JF, Slater P, Simpson MD, Gilchrist AC, Skan WJ, Royston MC, Reynolds GP, Cross AJ: Frontal cortical and left temporal glutamatergic dysfunction in schizophrenia. J Neurochem 1989; 52:1781-1786Crossref, Medline, Google Scholar
44. Bilder RM, Wu H, Bogerts B, Ashtari M, Robinson D, Woerner M, Lieberman JA, Degreef G: Cerebral volume asymmetries in schizophrenia and mood disorders: a quantitative magnetic resonance imaging study. Int J Psychophysiol 1999; 34:197-205Crossref, Medline, Google Scholar
45. Falkai P, Bogerts B, Greve B, Pfeiffer U, Machus B, Folsch-Reetz B, Majtenyi C, Ovary I: Loss of sylvian fissure asymmetry in schizophrenia: a quantitative post mortem study. Schizophr Res 1992; 7:23-32Crossref, Medline, Google Scholar
46. Crow TJ, Ball J, Bloom SR, Brown R, Bruton CJ, Colter N, Frith CD, Johnstone EC, Owens DG, Roberts GW: Schizophrenia as an anomaly of development of cerebral asymmetry: a postmortem study and a proposal concerning the genetic basis of the disease. Arch Gen Psychiatry 1989; 46:1145-1150Crossref, Medline, Google Scholar
47. Benes FM, Turtle M, Khan Y, Farol P: Myelination of a key relay zone in the hippocampal formation occurs in the human brain during childhood, adolescence, and adulthood. Arch Gen Psychiatry 1994; 51:477-484Crossref, Medline, Google Scholar
48. Foong J, Maier M, Barker GJ, Brocklehurst S, Miller DH, Ron MA: In vivo investigation of white matter pathology in schizophrenia with magnetisation transfer imaging. J Neurol Neurosurg Psychiatry 2000; 68:70-74Crossref, Medline, Google Scholar
49. Goldstein JM, Goodman JM, Seidman LJ, Kennedy DN, Makris N, Lee H, Tourville J, Caviness VS Jr, Faraone SV, Tsuang MT: Cortical abnormalities in schizophrenia identified by structural magnetic resonance imaging. Arch Gen Psychiatry 1999; 56:537-547Crossref, Medline, Google Scholar
50. Szeszko PR, Bilder RM, Lencz T, Pollack S, Alvir JM, Ashtari M, Wu H, Lieberman JA: Investigation of frontal lobe subregions in first-episode schizophrenia. Psychiatry Res 1999; 90:1-15Crossref, Medline, Google Scholar
51. Wright IC, Ellison ZR, Sharma T, Friston KJ, Murray RM, McGuire PK: Mapping of grey matter changes in schizophrenia. Schizophr Res 1999; 35:1-14Crossref, Medline, Google Scholar