Anomalous Asymmetry of Fusiform and Parahippocampal Gyrus Gray Matter in Schizophrenia: A Postmortem Study
Abstract
OBJECTIVE: Anomalies of structure and asymmetry of the parahippocampal gyrus (origin of the perforant path input to the hippocampal formation in the medial temporal lobe) have been shown in some postmortem studies of schizophrenia, but previous studies have not included the fusiform gyrus (which may have a role in facial recognition and naming), adjacent to the parahippocampal gyrus on the ventral occipitotemporal surface. METHOD: The volumes of gray matter in the left and right parahippocampal and fusiform gyri were assessed with a stereological point-counting technique in the temporal lobes from formalin-fixed brains of 27 comparison subjects and 31 patients with schizophrenia. Age was a covariate and gender was a factor in the analysis. RESULTS: In relation to the comparison subjects, the schizophrenic patients (both sexes) had lower volumes of both the parahippocampal and fusiform gyri on the left side. For both structures a left-greater-than-right volume asymmetry was present in the comparison subjects, but this asymmetry was reversed in the parahippocampal and fusiform gyri of the schizophrenic patients. A sex difference was present with respect to age at onset—degree of anomaly of asymmetry for both gyri increased with age at onset in men but not in women. CONCLUSIONS: The findings add substance to the view that the sex-related dimension of symmetry/asymmetry is integral to the disease process in schizophrenia and draw attention to the fusiform gyrus as a structure of particular interest in relation to disturbances of identification and naming in psychosis.
Anomalies of brain structure are a potential lead to the pathophysiology of schizophrenia, but the precise nature and distribution of such abnormalities have remained obscure. A degree of ventricular enlargement was established on the basis of radiological (1, 2) and postmortem (3, 4) studies and has been shown to be relatively constant across affected individuals (5–7). A modest reduction in cortical mass appears consistent across studies (8–10), as also does a loss of the subtle asymmetries, reflected in ventricular structure, that are characteristic of the human cerebral cortex (for reviews see references 11 and 12). A parsimonious view is that these three changes are interrelated and that changes in subcortical structures are secondary to global changes at the level of the cerebral cortex. However, this concept gives no direct lead to the origin and diversity of symptoms.
Interest has focused on abnormal structures in the medial temporal lobe, including particularly the parahippocampal gyrus (Brodmann’s areas 27, 28, 35, and 36). This structure receives input from heteromodal association areas of the cortex and gives rise to the perforant path that projects to the hippocampus and thereby transmits information into the limbic circuit. Reductions in volume (3, 13, 14) and cortical thickness (4, 15, 16) of the parahippocampal gyrus have been shown in postmortem studies, although there have also been reports (17, 18) of relative normality in various structural indices. In two studies (4, 15) less asymmetry of the cross-sectional gyral area was observed in patients with schizophrenia than in patients with affective disorder and in groups of normal subjects.
The fusiform gyrus (Brodmann’s areas 19, 20, and 37—sometimes referred to as the medial and lateral occipitotemporal gyri) lies immediately lateral to the parahippocampal gyrus in the temporal lobe and the lingual gyrus in the occipital lobe, on the ventral occipitotemporal surface (figure 1). The gyrus extends for most of the length of the inferior occipitotemporal surface, being bounded medially by the parahippocampal gyrus and laterally by the inferior occipitotemporal gyri. It has connections with striate and prestriate visual areas and projects to language-related regions, including Wernicke’s area in the lateral and superior aspects of the temporal lobe; this relationship is consistent with a role in facial recognition, postulated on the basis of functional magnetic resonance imaging (MRI) (19) and positron emission tomography (20) studies. Although such functions might be relevant to delusional misinterpretation syndromes, to our knowledge there have been no morphological or functional studies of the fusiform gyrus in schizophrenia, perhaps in part on account of its inaccessibility by radiological investigation. According to a postmortem study (21), the fusiform gyrus is one of the more asymmetric structures in the human brain.
The aim of the present study was to assess the structure of the parahippocampal and fusiform gyri in schizophrenia, with particular reference to their asymmetries. We predicted that the brains of schizophrenic subjects would have less asymmetry than would brains of subjects from the general population.
METHOD
Materials
Brains from patients with schizophrenia and from comparison subjects were collected from individuals for whom the next of kin had consented to use of tissues in medical research. The schizophrenic patients were selected on the basis of assessment of clinical notes by a psychiatrist (S.J.C. or T.J.C.) using the DSM-IV criteria for schizophrenia and schizoaffective disorder. The comparison subjects were individuals who had died without a history of neuropsychiatric disorder. The age at onset of schizophrenia (defined by first admission) was noted, and ratings were made of the quantity of neuroleptic medication taken during the subject’s lifetime (little, average, or much). The variable information in the case notes did not justify a more quantitative estimate of medication. The patient and comparison brains were prospectively collected at three centers (Oxford, Belfast, and Wickford, Essex) in the United Kingdom. The brains were fixed by suspension from the basilar artery in 10% formalin and assigned a randomized code by a third party, so that measurements could be made by persons blind to gender, diagnosis, and age. After fixation the brains were macroscopically and microscopically assessed to exclude other pathologies (including Alzheimer’s disease, Parkinson’s disease, and macro- and microscopic vascular disease) by using the criteria of the Consortium to Establish a Registry for Alzheimer’s Disease (22). This study included 13 female and 14 male comparison subjects and 13 female and 18 male patients with schizophrenia or schizoaffective disorder. The female comparison subjects and schizophrenic patients had mean ages of 73.5 years (range=48–90) and 74.7 years (range=44–91), respectively, and the mean ages of the men were 66.6 years (range=40–89) and 61.9 years (range=29–87). Four of the patients had had psychosurgery.
Stereology
Brain volumes, including volumes of gray matter in the regions of interest, were measured by unbiased stereological techniques using the Cavalieri principle (23–25). In order to measure the area and volume of the cortical mantle, the temporal lobes of the brains were sliced to yield section planes separated by known distances, from which it was then possible to determine volume, by using a test grid of points overlaid on randomly oriented section planes of the temporal lobe slices (26).
Procedure
After fixation the brains were carefully stripped of the leptomeninges. The hindbrain was removed by transection through the midbrain, and the cerebrum was bisected. The temporal and occipital lobes were separated from each cerebral hemisphere. The fusiform gyrus of each temporal lobe was defined by using waterproof calligraphy ink. The occipitotemporal lobes were then coronally sliced into 5-mm-thick slices, and the inferior surface was photographed (with a calibration rule in the field of view). The resulting images were then projected, at a magnification of ×2.3, onto a randomly positioned 1-cm-grid array of test points. For each gyrus (left and right, fusiform and parahippocampal), the test points falling on the gray matter on each slice were counted. The sum of these counts multiplied by a scaling coefficient yielded estimates of the gyral volume. The parahippocampal gyrus was defined in the following manner. The posterior boundary was the most posterior temporal lobe slice in which the hippocampus was visible. The anterior-most slice on which the gyrus was measured was at the level of the uncus, where the hippocampus merges with amygdala. The superior boundary was the interface between the hippocampus and the subiculum.
The fusiform gyrus was identified as follows: the medial margin was defined by the collateral and rhinal sulci, i.e., the boundary of the parahippocampal gyrus, and the lateral boundary was taken as the sulcus medial to the inferior temporal gyrus. The anterior extremity of the fusiform gyrus approached the temporal pole. The posterior boundary, as defined for the purpose of this study, was the most posterior temporal lobe slice in which the hippocampus was visible.
For each gyrus, the left and right sides were measured, and the asymmetry coefficient (δ) of the two sides was calculated as follows: δ = (right–left)/(right+left) × 100.
As the mean age of the women in the series was higher than that for the men, age was entered into all the analyses of variance as a covariate to yield a regression analysis for the effects of age.
Accuracy
An assessment of the accuracy of the estimate of the mean volume of a structure for each comparison group (female comparison, male comparison, female schizophrenia, male schizophrenia) is given by the group coefficient of error (standard error of the mean divided by the mean). The lower the value of this ratio, the more accurately the mean value attained reflects the true population mean. For both the parahippocampal and fusiform gyri, all groups had group coefficient errors below 0.092.
The observed coefficient of error for the individual estimates of volume were calculated as described by Gundersen and Jensen (23). The mean observed coefficient of error for the different volumes were as follows: parahippocampal gyrus, 0.087 for the left and 0.086 for the right; fusiform gyrus, 0.080 for the left and 0.081 for the right.
From the coefficients of error for the individual volume measures, one can estimate the percentage of observed relative variance of each measure that is accounted for by true interindividual variance, as opposed to the stereological volume estimate (27, 28). True interindividual variation accounted for a minimum of 74% for each measure for each group.
RESULTS
For each gyrus, the asymmetry data and left and right volumes were entered into an analysis of covariance (ANCOVA) with diagnosis and gender as factors and age as covariate. This generates a statistic z, which is assessed in terms of the normal distribution to produce a p value (i.e., without degrees of freedom). The results of these analyses are summarized in table 1.
Parahippocampal Gyrus
For the left parahippocampal gyrus volume (table 1 and figure 2, top), the ANCOVA revealed no effect of gender and no gender-by-diagnosis interaction. There was a nonsignificant effect of diagnosis, which corresponded to a 10.6% smaller volume of the left parahippocampal gyrus in the schizophrenic group. There was no significant effect of age.
For the right parahippocampal gyrus volume (table 1 and figure 2, top), the ANCOVA revealed no effect of diagnosis, no effect of gender, and no gender-by-diagnosis interaction. There was a significant effect of age corresponding to a volume decrease in this gyrus of 14.4 mm3, on average, per year.
For the asymmetry of the parahippocampal gyrus volume (table 1 and figure 2, top, and figure 3, top), the ANCOVA revealed a significant effect of diagnosis, such that the comparison subjects showed a left-greater-than-right asymmetry and the schizophrenic patients showed a right-greater-than-left asymmetry. There was no effect of gender and no gender-by-diagnosis interaction. There was no significant effect of age.
The analyses of the parahippocampal gyrus asymmetry and left-side volume were repeated after exclusion of the patients who had undergone leukotomy, to elucidate whether the apparent diagnosis effects were due to psychosurgery. The repeat analysis revealed a significant effect of diagnosis (F=4.62, df=1, 49, p=0.04) on the left parahippocampal volume and a nonsignificant effect of diagnosis (F=3.42, df=1, 49, p=0.07) on asymmetry.
Fusiform Gyrus
For the left fusiform gyrus volume (table 1 and figure 2, bottom), the ANCOVA revealed a significant effect of diagnosis and a significant effect of gender, such that the women had approximately 15% smaller volumes than the men, but no gender-by-diagnosis interaction. The effect of diagnosis corresponded to 13.2% smaller left fusiform gyrus volumes in the patients with schizophrenia than in the comparison subjects. There was no significant effect of age.
For the right fusiform gyrus volume (table 1 and figure 2, bottom), the ANCOVA revealed no effect of diagnosis, a significant effect of gender, such that the women had 19.0% smaller volumes than the men, and no gender-by-diagnosis interaction. There was a significant effect of age, suggesting that the volume of this structure decreased by 20.3 mm3 per year.
For the asymmetry of the fusiform gyrus volume (table 1 and figure 2, bottom, and figure 3, bottom), the ANCOVA revealed a significant effect of diagnosis. There was no effect of gender and no gender-by-diagnosis interaction. As for the parahippocampal gyrus, the comparison subjects showed a left-greater-than-right asymmetry and the schizophrenic patients had a right-greater-than-left asymmetry (figure 2, bottom, and figure 3, bottom). There was no significant effect of age. Again, analysis of the fusiform gyrus data was repeated after exclusion of the patients who had undergone leukotomy. The repeat ANCOVAs for the fusiform gyrus revealed significant effects of diagnosis on left-side volume (F=7.64, df=1, 49, p=0.008) and on asymmetry (F=5.89, df=1, 49, p=0.02).
The similarity of the abnormalities in left (but not right) gyrus volume in both the parahippocampal and fusiform gyri of the schizophrenic patients raises the question of whether they reflect the same cause. Across the entire cohort, the fusiform and parahippocampal gyrus asymmetries showed a statistically significant Pearson’s correlation coefficient (r=0.50, df=58, p<0.0005). The correlation was significant within the schizophrenic patients (r=0.66, df=31, p<0.0005) but not the comparison subjects (r=0.23, df=27, p=0.24). After Fisher’s z transformation was applied, a t test comparison revealed a significant difference (t=2.01, df=54, p=0.05) between the correlation coefficients for the comparison subjects and patients.
Age at Onset
Onset for the male patients (mean age=28.94, SD=7.34) was earlier than for the female patients (mean=32.23, SD=9.43), as is usually found, although the difference was not statistically significant (t=1.08, df=28, p=0.29). The asymmetry scores were found to relate to age at onset. Figure 4 (top) reveals a positive correlation between the asymmetry coefficient for the parahippocampal gyrus and age at onset for men (r=0.67, df=18, p=0.002) and a negative correlation between the asymmetry coefficient and age at onset for women (r=–0.46, df=13, p=0.12). Thus, for female schizophrenic patients, age at onset was not significantly related to parahippocampal gyrus asymmetry. For male patients, however, there was a relationship such that patients with a left-greater-than-right asymmetry had earlier onsets. With increasing rightward asymmetry, later onset was seen. The correlation coefficients for men and women were subjected to Fisher’s z transformation and compared by means of a t test. This revealed the gender difference to be significant (t=3.23, df=27, p=0.003).
Figure 4 (bottom) shows a similar relationship between asymmetry in the fusiform gyrus and age at onset for the two sexes. For women there was a nonsignificant negative correlation between asymmetry and age at onset (r=–0.48, df=13, p=0.09). For men there was a significant positive correlation between the two (r=0.50, df=18, p=0.04). The difference between these two correlation coefficients was significant (t=2.65, df=27, p=0.01). Thus, for the female patients, age at onset was not significantly related to fusiform gyrus asymmetry. For the male patients, however, those with a left-greater-than-right asymmetry had earlier onsets. With increasing rightward asymmetry (i.e., with increasing anomaly), later onset was seen.
Neuroleptic Medication and Postmortem Delay
The mean delay between death and brain removal for the groups was 43.38 hours (SD=25.80) for the comparison women, 41.38 hours (SD=29.29) for the comparison men, 39.69 hours (SD=32.89) for the female patients, and 44.65 hours (SD=32.56) for the male patients. There were no significant intergroup differences (diagnosis: F<0.005, df=1, 52, p=1.00; gender: F=0.03, df=1, 52, p=0.86; interaction: F=0.18, df=1, 52, p=0.67).
Finally, we performed an ANCOVA on the data from the schizophrenic patients to ensure that the findings were not an artifact of the neuroleptic medication that the patients had taken (see Method section). Lifetime medication level (little, average, much) was entered as the only factor, with age as a covariate. No effect of medication was found for parahippocampal gyrus asymmetry (F=0.74, df=2, 27, p=0.49) or fusiform gyrus asymmetry (F<0.005, df=2, 27, p=1.00).
Quester and Schröder (29) demonstrated that any alteration due to the fixation of brains proceeds rapidly to a stable state after a maximum of 3 weeks. As all of our brains were fixed for much longer than this, all would have reached their stable, postfixation configurations. Thus, no differences between groups by fixation artifact would be expected.
DISCUSSION
The salient observation was the reversal in schizophrenia of the asymmetries of the gray matter of the parahippocampal and fusiform gyri that are characteristic of the brains of most normal individuals (table 1). Two previous studies (4, 15) are relevant to our laterality findings for the parahippocampal gyrus. Although both studies showed anomalies of laterality in schizophrenia, single cross-sectional areas were measured. Further, in the latter investigation (15) the study group was small (nine male and three female patients). In contrast, the stereological volume measures described here provide a more meaningful assessment of the anatomy of this structure and are not influenced by random variations in the precise region of gyrus measured.
The findings for the fusiform gyrus extend the parahippocampal observations. The strategy of demarcating the fusiform gyrus with ink before sampling allows greater accuracy in outlining this structure on coronal slices. The abnormalities in the two gyri are similar. On the right, there was a modest age-related loss of substance (14.4 mm3 per year for parahippocampal gyrus, 20.3 mm3 per year for fusiform gyrus). On the left side, there was evidence of a loss of substance in schizophrenia (consistent with the findings of the MRI studies reviewed by Lawrie and Abukmeil [30]) that is of the order of 10.6% (p=0.05) for the parahippocampal gyrus and 13.2% (p=0.01) for the fusiform gyrus. Most striking is the change from left-sided predominance in the comparison subjects to right-sided predominance in the patients (p<0.05 and 0.03 for the parahippocampal and fusiform gyri, respectively; see figure 2 and figure 3). This pattern may be the result of either of two changes in brain configuration. The first possibility is a purely left-sided reduction in volume. The second corresponds to an asymmetry shift superimposed on a bilateral reduction in volume, as described by Ward et al. (31).
By contrast with recent observations on surface asymmetries in the cortex (32) and in callosal fiber densities (33), no strong interactions with sex were apparent in the raw volume assessments. However, such interactions were uncovered when age at onset of schizophrenia was taken into account (figure 4). For the women, the correlations between asymmetry index and age at onset were negative and nonsignificant. For the men, the correlations were positive and significant. The differences between the genders were highly significant. Thus, greater age at onset is associated with increasingly atypical asymmetry in men but not in women. This conclusion may be relevant to the general finding of a 2–3-year earlier onset in males (34–36).
The findings can be viewed in the context of the recent literature on structural brain abnormalities in schizophrenia. In addition to larger ventricles and somewhat smaller overall cortical mass, in relation to normal subjects, there is evidence for a difference in asymmetry (generally less) in postmortem and radiological studies with indexes that include scan densities (37), brain widths (38–40), segmental volumes (41, 42), cortical surface measurements (32), length of the Sylvian fissure (43, 44), planum temporale assessments (45–47), volume (48) and gray matter (49) assessments of the temporal lobe, and area (50) and volume (51) assessments of the temporal horn. Although the abnormalities are consistent, many are small in magnitude, and contrary interpretations have been expressed (52–55). If, as has been claimed (11, 12, 50), loss of asymmetry is the most characteristic feature of the brain in schizophrenia, and a clue to the genetic predisposition, some explanation for the subtlety and distribution of the asymmetries in the normal brain and their deviations in schizophrenia is required. While some abnormalities in asymmetry, such as those in the present study, are relatively large (on the order of 5% to 6%), in other studies (e.g., of cerebral widths) the differences are smaller (on the order of 1% or 2%), and sometimes no such differences are detected (e.g., in the lateral measures reported by us [32] and Flaum et al. [52]). A possible explanation is that the asymmetries are local rather than global and can be detected only when the relevant cortical structures are assessed. Thus, it appears that there is an axis of asymmetry from the right dorsolateral frontal to the left occipitotemporal cortex and that this asymmetry is expressed to a greater extent in “heteromodal association” areas (56) than in primary sensorimotor and association cortex. Such a distribution is consistent with the concept that the areas that are latest to develop in ontogeny carry the greatest asymmetries, and these areas are also those that would be expected to show the greatest interindividual variability. The correlation between the two asymmetries in schizophrenia, and its absence in the comparison subjects, could be taken as evidence for greater connectivity between the fusiform and parahippocampal gyri in schizophrenia. We have previously offered such an interpretation for a greater degree of gyrification in the temporal lobe (57).
One can speculate on the possible functions of the structures in which variations relating to schizophrenia have been found. While the parahippocampal gyrus appears to play a very general role in transmitting information from all areas of the cortex into the hippocampus and limbic circuit, the fusiform gyrus, at least in its posterior extent, is more selectively related to the visual modality. In that anteriorly it approximates to the basal speech area in the inferior temporal gyrus (58), it might plausibly be assigned a role in attaching verbal labels to important visual inputs. In functional imaging studies, activation of the fusiform gyrus has been demonstrated during facial recognition (19) and matching (20, 59–61), while losses of facial, gender, and object recognition are reported after lesions in this region, with deficits in biographical information being greater with lesions in the nondominant hemisphere and deficits in facial recognition greater with lesions in the dominant hemisphere (62). A magnetoencephalographic study revealed face-specific responses in the inferior occipitotemporal cortex in the right hemisphere (61). A key component in the human use of language is the ability to recognize, name, and attribute characteristics to others. If the fusiform gyrus contributes to this process, it is conceivable that a deviation could be manifested as a delusional misidentification or other syndrome of a paranoid type.
The sex difference in age at onset of psychosis is unexplained (35, 36). In another study (32) on this set of brains we observed a sex difference in the asymmetry of the length of the frontal lobe (normal men have a right-greater-than-left asymmetry, and normal women have a left-greater-than-right asymmetry) that was reversed in patients suffering from schizophrenia. These tendencies were differentially related to age at onset, just as the present findings on the parahippocampal and fusiform gyri were correlated with age at onset in men and not in women. Although we have no detailed explanation, these relationships are consistent with the possibility that the sex difference in age at onset in schizophrenia is directly related to the sex difference in cerebral asymmetry, a difference that itself can be explained by the fact that the gene for asymmetry is in the class that is present in homologous form on the X and Y chromosomes. This hypothesis is based on the neuropsychological deficits associated with sex chromosome aneuploidies (63–65) and is consistent with observations on the transmission of handedness within families (66).
Received March 29, 1999; revision received July 2, 1999; accepted July 9, 1999. From the Schizophrenia Research Group, Department of Neuropathology, Radcliffe Infirmary, Oxford, U.K.; the Regional Neuropathology Laboratory, Royal Hospitals Trust, Belfast, U.K.; the Department of Mental Health, The Queen’s University of Belfast; and the Prince of Wales International Centre, University Department of Psychiatry, Warneford Hospital, Headington, U.K. Address reprint requests to Dr. Crow, University Department of Psychiatry, Warneford Hospital, Oxford OX3 7JX, U.K.; [email protected] (e-mail). This work was supported by a Medical Research Council project grant to Drs. Crow and Esiri, by a Medical Research Council research studentship to Dr. Highley, and by the U.K. charity SANE. The authors thank the late Clive Bruton and the staff of the Neuropathology Department at Runwell Hospital for help in collecting some of the material in this series and Mr. Tim Marshall and Dr. Mario Cortina Borja for help with the Fisher’s z test.
![]() |
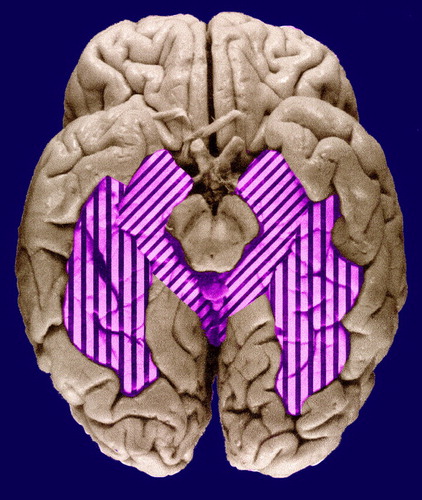
FIGURE 1. Parahippocampal Gyrus (diagonal stripes) and Fusiform Gyrus (vertical stripes) on the Ventral Occipitotemporal Surface of the Brain
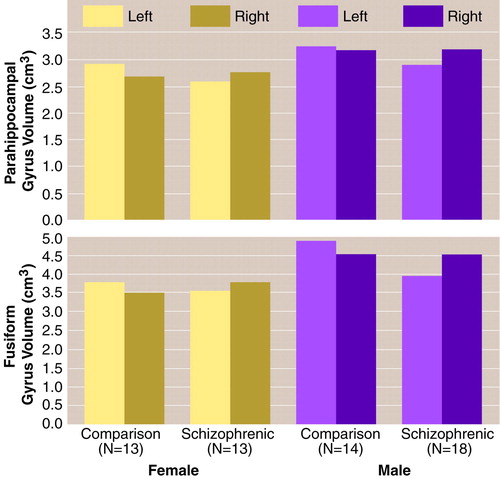
FIGURE 2. Volumes of Parahippocampal Gyrus and Fusiform Gyrus in Postmortem Brains From Female and Male Patients With Schizophrenia and Normal Comparison Subjects
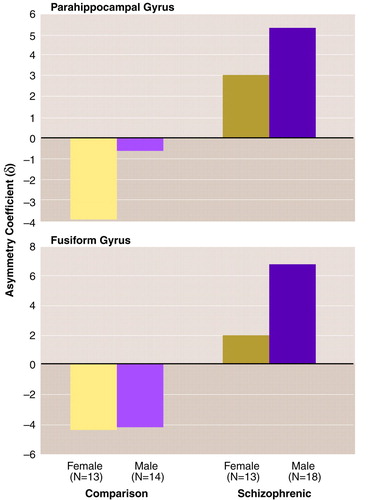
FIGURE 3. Asymmetries of Parahippocampal Gyrus and Fusiform Gyrus in Postmortem Brains From Female and Male Patients With Schizophrenia and Normal Comparison Subjects
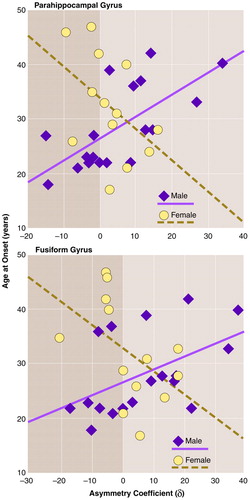
FIGURE 4. Relation of Age at Onset to Asymmetries of Parahippocampal Gyrus and Fusiform Gyrus for 13 Female and 18 Male Patients With Schizophrenia a
aThe differences between the regression lines for the two sexes were significant for the parahippocampal gyrus (p=0.002) and for the fusiform gyrus (p=0.01).
1. Johnstone EC, Crow TJ, Frith CD, Husband J, Kreel L: Cerebral ventricular size and cognitive impairment in chronic schizophrenia. Lancet 1976; 2:924–926Crossref, Medline, Google Scholar
2. Weinberger DR, Torrey EF, Neophytides AN, Wyatt RJ: Lateral cerebral ventricular enlargement in chronic schizophrenia. Arch Gen Psychiatry 1979; 36:735–739Crossref, Medline, Google Scholar
3. Bogerts B, Meerts E, Schonfeldt-Bausch R: Basal ganglia and limbic system pathology in schizophrenia: a morphometric study of brain volume and shrinkage. Arch Gen Psychiatry 1985; 42:784–791Crossref, Medline, Google Scholar
4. Brown R, Colter N, Corsellis JAN, Crow TJ, Frith CD, Jagoe R, Johnstone EC, Marsh L: Postmortem evidence of structural brain changes in schizophrenia: differences in brain weight, temporal horn area, and parahippocampal gyrus compared with affective disorder. Arch Gen Psychiatry 1986; 43:36–42Crossref, Medline, Google Scholar
5. Harvey I, McGuffin P, Williams M, Toone BK: The ventricle-brain ratio (VBR) in functional psychoses: an admixture analysis. Psychiatr Res Neuroimaging 1990; 35:61–69Crossref, Medline, Google Scholar
6. Owens DGC, Johnstone EC, Crow TJ, Frith CD, Jagoe JR, Kreel L: Cerebral ventricular enlargement in schizophrenia: relationship to the disease process and its clinical correlates. Psychol Med 1985; 15:27–41Crossref, Medline, Google Scholar
7. Daniel DG, Goldberg TE, Gibbons RD, Weinberger DR: Lack of a bimodal distribution of ventricular size in schizophrenia: a Gaussian mixture analysis of 1056 cases and controls. Biol Psychiatry 1991; 30:886–903Crossref, Google Scholar
8. Zipursky RB, Lim KO, Sullivan EV, Brown BW, Pfefferbaum A: Widespread cerebral grey matter volume deficits in schizophrenia. Arch Gen Psychiatry 1992; 49:195–205Crossref, Medline, Google Scholar
9. Harvey I, Ron MA, Du Boulay S, Wicks D, Lewis SW, Murray RM: Reduction of cortical volume in schizophrenia on magnetic resonance imaging. Psychol Med 1993; 23:591–604Crossref, Medline, Google Scholar
10. Lim KO, Tew W, Kushner M, Chow K, Matsumoto B, DeLisi LE: Cortical gray matter volume deficit in patients with first-episode schizophrenia. Am J Psychiatry 1996; 153:1548–1553Google Scholar
11. Crow TJ: Temporal lobe asymmetries as the key to the etiology of schizophrenia. Schizophr Bull 1990; 16:433–443Crossref, Medline, Google Scholar
12. Crow TJ: Schizophrenia as failure of hemispheric dominance for language. Trends Neurosci 1997; 20:339–343Crossref, Medline, Google Scholar
13. Falkai P, Bogerts B, Rozumek M: Limbic pathology in schizophrenia: the entorhinal region—a morphometric study. Biol Psychiatry 1988; 24:515–521Crossref, Medline, Google Scholar
14. Jeste DV, Lohr JB: Hippocampal pathologic findings in schizophrenia: a morphometric study. Arch Gen Psychiatry 1989; 46:1019–1024Google Scholar
15. Altschuler LL, Casanova MF, Goldberg TE, Kleinman JE: The hippocampus and para-hippocampus in schizophrenic, suicide and control brains. Arch Gen Psychiatry 1990; 47:1029–1034Google Scholar
16. Colter N, Battal S, Crow TJ, Johnstone EC, Brown R, Bruton C: White matter reduction in the parahippocampal gyrus of patients with schizophrenia (letter). Arch Gen Psychiatry 1987; 44:1023Crossref, Medline, Google Scholar
17. Heckers S, Heinsen H, Heinsen YC, Beckmann H: Limbic structures and lateral ventricle in schizophrenia: a quantitative postmortem study. Arch Gen Psychiatry 1990; 47:1016–1022Google Scholar
18. Benes FM, Sorensen I, Bird ED: Reduced neuronal size in posterior hippocampus of schizophrenic patients. Schizophr Bull 1991; 17:597–608Crossref, Medline, Google Scholar
19. Kanwisher N, McDermott J, Chun MM: The fusiform face area: a module in human extrastriate cortex specialized for face perception. J Neurosci 1997; 17:4302–4311Google Scholar
20. Grady CL: Age-related changes in cortical blood flow activation during perception and memory. Ann NY Acad Sci 1996; 777:14–21Crossref, Medline, Google Scholar
21. Kopp N, Michel P, Carrier H, Biron A, Duviellard P: Études de certaines asymétries hémisphériques du cerveau humain. J Neurol Sci 1977; 34:349–363Crossref, Medline, Google Scholar
22. Mirra SS, Heyman A, McKeel D, Sumi SM, Crain BJ, Brownlee LM, Vogel FS, Hughes JP, van Belle G, Berg L: The Consortium to Establish a Registry for Alzheimer’s Disease (CERAD), part II: standardization of the neuropathologic assessment of Alzheimer’s disease. Neurology 1991; 41:479–486Crossref, Medline, Google Scholar
23. Gundersen HJG, Jensen EB: The efficiency of systematic sampling in stereology and its prediction. J Microsc 1987; 147:229–263Crossref, Medline, Google Scholar
24. Regeur L, Pakkenberg B: Optimizing sampling designs for volume measurements of components of human brain using a stereological method. J Microsc 1989; 155:113–121Crossref, Medline, Google Scholar
25. Pakkenberg B: Total nerve cell number in neocortex in chronic schizophrenics and controls estimated using optical dissectors. Biol Psychiatry 1993; 34:768–772Crossref, Medline, Google Scholar
26. Braendgaard H, Evans SM, Howard CV, Gundersen HJG: The total number of neurons in the human neocortex unbiasedly estimated using optical dissectors. J Microsc 1990; 157:285–304Crossref, Medline, Google Scholar
27. West MJ, Gundersen HJG: Unbiased stereological estimation of the number of neurons in the human hippocampus. J Comp Neurol 1990; 296:1–22Crossref, Medline, Google Scholar
28. West MJ: New stereological methods for counting neurons. Neurobiol Aging 1993; 14:275–285Crossref, Medline, Google Scholar
29. Quester R, Schröder R: The shrinkage of the human brain stem during formalin fixation and embedding in paraffin. J Neurosci Methods 1997; 75:81–89Crossref, Medline, Google Scholar
30. Lawrie SM, Abukmeil SS: Brain abnormality in schizophrenia: a systematic and quantitative review of volumetric magnetic resonance imaging studies. Br J Psychiatry 1998; 172:110–120Crossref, Medline, Google Scholar
31. Ward KE, Friedman L, Wise A, Schulz SC: Meta-analysis of brain and cranial size in schizophrenia. Schizophr Res 1996; 22:197–213Crossref, Medline, Google Scholar
32. Highley JR, Esiri MM, Cortina-Borja M, McDonald B, Cooper SJ, Herron B, Crow TJ: Anomalies of cerebral asymmetry in schizophrenia interact with gender and age of onset: a post-mortem study. Schizophr Res 1998; 34:13–25Crossref, Medline, Google Scholar
33. Highley JR, Esiri MM, McDonald B, Cortina-Borja M, Herron B, Cooper SJ, Crow TJ: The size and fibre composition of the corpus callosum with respect to gender and schizophrenia: a post-mortem study. Brain 1999; 122:99–110Crossref, Medline, Google Scholar
34. Penrose LS: Survey of cases of familial mental illness. Eur Arch Psychiatry Neurol Sci 1991; 240:315–324Crossref, Google Scholar
35. Hafner H, Maurer K, Loffler W, Riecher-Rossler A: The influence of age and sex on the onset and early course of schizophrenia. Br J Psychiatry 1993; 162:80–86Crossref, Medline, Google Scholar
36. Lewine RRJ: Ontogenetic implications of sex differences in schizophrenia, in Schizophrenia: A Life-Course Developmental Perspective. Edited by Walker EF. San Diego, Academic Press, 1991, pp 195–211Google Scholar
37. Reveley MA, Reveley AM, Baldy R: Left hemisphere hypodensity in discordant schizophrenic twins: a controlled study. Arch Gen Psychiatry 1987; 44:625–632Crossref, Medline, Google Scholar
38. Crow TJ, Colter N, Frith CD, Johnstone EC, Owens DGC: Developmental arrest of cerebral asymmetries in early onset schizophrenia. Psychiatry Res 1989; 29:247–253Crossref, Medline, Google Scholar
39. Falkai P, Schneider T, Greve B, Klieser E, Bogerts B: Reduced frontal and occipital lobe asymmetry on the CT scans of schizophrenic patients: its specificity and clinical significance. J Neural Transm Gen Sect 1995; 99:63–77Crossref, Medline, Google Scholar
40. DeLisi LE, Sakuma M, Kushner M, Finer DL, Hoff AL, Crow TJ: Anomalous cerebral asymmetry and language processing in schizophrenia. Schizophr Bull 1997; 23:255–271Crossref, Medline, Google Scholar
41. Turetsky B, Cowell PE, Gur RC, Grossman RI, Shtasel DL, Gur RE: Frontal and temporal lobe brain volumes in schizophrenia: relationship to symptoms and clinical subtype. Arch Gen Psychiatry 1995; 52:1061–1070Google Scholar
42. Bilder RM, Wu H, Bogerts B, Degreef G, Ashtari M, Alvir JMJ, Snyder PJ, Lieberman JA: Absence of regional hemispheric volume asymmetries in first-episode schizophrenia. Am J Psychiatry 1994; 151:1437–1447Google Scholar
43. Falkai P, Bogerts B, Greve B, Pfeiffer U, Machus B, Folsch-Reetz B, Majtenyi C, Ovary I: Loss of Sylvian fissure asymmetry in schizophrenia: a quantitative post mortem study. Schizophr Res 1992; 7:23–32Crossref, Medline, Google Scholar
44. Crow TJ, Brown R, Bruton CJ, Frith CD, Gray V: Loss of Sylvian fissure asymmetry in schizophrenia: findings in the Runwell 2 series of brains. Schizophr Res 1992; 6:152–153Crossref, Google Scholar
45. Rossi A, Stratta P, Mattei P, Cupillari M, Bozzao A, Gallucci M, Casacchia M: Planum temporale in schizophrenia: a magnetic resonance study. Schizophr Res 1992; 7:19–22Crossref, Medline, Google Scholar
46. Petty RG, Barta PE, Pearlson GD, McGilchrist IK, Lewis RW, Tien AY, Pulver A, Vaughn DD, Casanova MF, Powers RE: Reversal of asymmetry of the planum temporale in schizophrenia. Am J Psychiatry 1995; 152:715–721Link, Google Scholar
47. Falkai P, Bogerts B, Schneider T, Greve B, Pfeiffer U, Pilz K, Gonsiorzcyk C, Majtenyi C, Ovary I: Disturbed planum temporale asymmetry in schizophrenia: a quantitative post-mortem study. Schizophr Res 1995; 14:161–176Crossref, Medline, Google Scholar
48. Johnstone EC, Owens DG, Crow TJ, Frith CD, Alexandropolis K, Bydder G, Colter N: Temporal lobe structure as determined by nuclear magnetic resonance in schizophrenia and bipolar affective disorder. J Neurol Neurosurg Psychiatry 1989; 52:736–741Crossref, Medline, Google Scholar
49. Suddath RL, Christison GW, Torrey EF, Casanova MF, Weinberger DR: Anatomical abnormalities in the brains of monozygotic twins discordant for schizophrenia. N Engl J Med 1990; 322:789–794; correction, 322:1616Google Scholar
50. Crow TJ, Ball J, Bloom SR, Brown R, Bruton CJ, Colter N, Frith CD, Johnstone EC, Owens DG, Roberts GW: Schizophrenia as an anomaly of development of cerebral asymmetry: a postmortem study and a proposal concerning the genetic basis of the disease. Arch Gen Psychiatry 1989; 46:1145–1150Google Scholar
51. Bogerts B, Ashtari M, Degreef G, Alvir JMJ, Bilder RM, Lieberman J: Reduced temporal limbic structure volumes on magnetic resonance images in first episode schizophrenia. Psychiatr Res Neuroimaging 1990; 35:1–13Crossref, Medline, Google Scholar
52. Flaum M, Swayze VW II, O’Leary DS, Yuh WTC, Ehrhardt JC, Arndt SV, Andreasen NC: Effects of diagnosis, laterality, and gender on brain morphology in schizophrenia. Am J Psychiatry 1995; 152:704–714Link, Google Scholar
53. Weinberger DR, Suddath RC, Casanova MF, Torrey EF, Kleinman JE: Crow’s “lateralization hypothesis” for schizophrenia (letter). Arch Gen Psychiatry 1991; 48:85Crossref, Medline, Google Scholar
54. Bartley AJ, Jones DW, Torrey EF, Zigun JR, Weinberger DR: Sylvian fissure asymmetries in monozygotic twins: a test of laterality in schizophrenia. Biol Psychiatry 1993; 34:853–863Crossref, Medline, Google Scholar
55. Kulynych JJ, Vladar K, Fantic BD, Jones DW, Weinberger DR: Normal asymmetry of the planum temporale in patients with schizophrenia: three dimensional cortical morphometry with MRI. Br J Psychiatry 1995; 166:742–749Crossref, Medline, Google Scholar
56. Pearlson GD, Barta PE, Schlaepfer TE, Petty RG, Tien AY, McGilchrist IK: Heteromodal association cortex in schizophrenia—grey matter changes and clinical correlates. Schizophr Res 1995; 15:1–2Crossref, Medline, Google Scholar
57. Highley JR, Esiri MM, McDonald B, Cooper SJ, Crow TJ: Temporal lobe length is reduced, and gyral folding is increased in schizophrenia: a post mortem study. Schizophr Res 1998; 34:1–12Crossref, Medline, Google Scholar
58. Lüders H, Lesser RP, Hahn J, Dinner DS, Morris HH, Wyllie E, Godoy J: Basal temporal language area. Brain 1991; 114:743–754Crossref, Medline, Google Scholar
59. Seeck M, Mainwaring N, Ives J, Blume H, Dubuisson D, Cosgrove R, Mesulam MM, Schomer DL: Differential neural activity in the human temporal lobe evoked by faces of family members and friends. Ann Neurol 1993; 34:369–372Crossref, Medline, Google Scholar
60. Mikami A, Nakamura K, Kubota K: Neuronal responses to photographs in the superior temporal sulcus of the rhesus monkey. Behav Brain Res 1994; 60:1–13Crossref, Medline, Google Scholar
61. Sams M, Hietanen JK, Hari R, Ilmoniemi RJ, Lounasmaa OV: Face-specific responses from the human inferior occipito-temporal cortex. Neuroscience 1997; 77:49–55Crossref, Medline, Google Scholar
62. Sergent J, Signoret JL: Varieties of functional deficit in prosopagnosia. Cereb Cortex 1992; 2:375–388Crossref, Medline, Google Scholar
63. Crow TJ: Sexual selection, Machiavellian intelligence and the origins of psychosis. Lancet 1993; 342:594–598Crossref, Medline, Google Scholar
64. Crow TJ: Sexual selection, timing and the descent of man: a theory of the genetic origins of language. Cahiers de Psychologie Cognitive 1998; 17:1079–1114Google Scholar
65. Netley CT: Sex chromosome aneuploidy and cognitive development. Cahiers de Psychologie Cognitive 1998; 17:1190–1197Google Scholar
66. Corballis MC, Lee K, McManus IC, Crow TJ: Location of the handedness gene on the X and Y chromosomes. Am J Med Genet Neuropsychiatr Genet 1996; 67:50–52Crossref, Medline, Google Scholar