Serial CSF Corticotropin-Releasing Hormone Levels and Adrenocortical Activity in Combat Veterans With Posttraumatic Stress Disorder
Abstract
OBJECTIVE: The authors sought to carefully test, by using a technique of continuous CSF sampling, the hypothesis that basal elevations in CSF corticotropin-releasing hormone (CRH) concentrations exist in patients with posttraumatic stress disorder (PTSD). They also sought to assess the relationship among PTSD symptoms, adrenocortical activity, and CSF CRH levels. METHOD: CSF was withdrawn by means of a flexible, indwelling subarachnoid catheter over a 6-hour period, and hourly CSF concentrations of CRH were determined for 11 well-characterized combat veterans with PTSD and 12 matched normal volunteers. Twenty-four-hour urinary-free cortisol excretion was also determined. PTSD and depressive symptoms were correlated with the neuroendocrine data. RESULTS: Mean CSF CRH levels were significantly greater in PTSD patients than in normal subjects (55.2 [SD=16.4] versus 42.3 pg/ml [SD=15.6]). No correlation was found between CSF CRH concentrations and PTSD symptoms. While there was no significant difference between groups in 24-hour urinary-free cortisol excretion, the correlation between 24-hour urinary-free cortisol excretion and PTSD symptoms was negative and significant. CONCLUSIONS: By using a serial CSF sampling technique, the authors found high basal CSF CRH concentrations and normal 24-hour urinary-free cortisol excretion in combat veterans with PTSD, a combination that appears to be unique among psychiatric conditions studied to date.
The development of posttraumatic stress disorder (PTSD) is intrinsically related to the experience of overwhelming trauma. Because corticotropin-releasing hormone (CRH) is one of the principal central nervous system (CNS) effectors of the organismic response to stress, the regulation of this neurohormone in PTSD has been of interest, and CNS CRH hypersecretion has been hypothesized (1). Indeed, preliminary results from a study using lumbar puncture indicate that CSF CRH concentrations are elevated in combat veterans with PTSD (2). We sought to more closely examine the hypothesis that CRH is hypersecreted into the CNS of patients with PTSD by using a technique of serial CSF sampling in which CSF aliquots were collected over a 6-hour period by means of an indwelling, flexible subarachnoid catheter.
Use of continuous or serial CSF sampling confers several advantages over standard lumbar puncture in the examination of CSF CRH concentrations. First of all, CRH appears to be both secreted into human CSF in a pulsatile manner (3) and rapidly transported out of the CNS (4), rendering a single time assessment of its concentration of limited value. In addition, human CSF CRH levels exhibit a diurnal variation in concentration (5). Moreover, the procedure of lumbar puncture is associated with significant anxiety in most human subjects. We have shown that this procedure is associated with acute increases in levels of CSF CRH (6). To control for these problems, we have used serial CSF sampling in combat veterans with PTSD and normal comparison subjects in order to determine basal levels of CRH.
METHOD
Subjects
The study was approved by the Institutional Review Board of the University of Cincinnati Medical Center and the Research Committee of the Cincinnati Veterans Affairs (VA) Medical Center. Written informed consent was obtained from each patient and volunteer before their participation.
We studied 12 healthy men who were carefully screened through use of the Structured Clinical Interview for DSM-III-R (SCID) and unstructured exploratory clinical interviews to exclude those with a current or past psychiatric disorder, current substance abuse, or a history of these disorders in first-degree relatives. The volunteers had a mean age of 41.4 years (SD=8.2, range=23–50) and had a mean body mass index of 25.9 kg/m2 (SD=4.1). All subjects were medication free and without health problems.
The 11 patients with PTSD, who were also administered the SCID, had a mean age of 42.5 years (SD=9.2, range=23–49) and a mean body mass index of 29.1 kg/m2 (SD=3.6). All patients had suffered severe combat-related trauma, nine in Vietnam and two in Iraq during the Gulf war (data on file). Six of the 11 patients had histories of alcohol and drug abuse but had been abstinent from substances of abuse (excluding tobacco) for, respectively, 15, 10, 7, 2.5, 1.4, and 0.5 years before the study. Abstinence was documented by self-report and urine toxicology screens. Six of the 11 patients with PTSD and four of the 12 normal volunteers smoked tobacco until the evening before the study. None of the patients was clinically depressed at the time of the study, but six had histories of major depression (all unipolar, with the exception of one patient with bipolar II disorder). Seven patients had taken psychoactive medication in the past but had discontinued all medications at least five half-lives before the study. The patients’ mean score on the Clinician-Administered PTSD Scale (7) was 82.1 (SD=20.4).
Procedure
Continuous collection of CSF
Subjects followed a controlled low-monoamine diet for at least 3 days before admission to the Psychobiology Research Unit at the VA Medical Center, Cincinnati. A standard 666-calorie preload meal (20% protein, 24% fat, and 56% carbohydrate) was consumed at 8:00 p.m. the evening before the study began. Participants fasted following the meal, with the exception of being allowed to drink water and to smoke cigarettes until midnight. The next morning, a 20-gauge catheter was placed in the lumbar subarachnoid space at approximately 8:00 a.m., as previously described (8, 9). At 11:00 a.m., approximately 3 hours after the subarachnoid catheter placement, continuous CSF withdrawal was begun into iced test tubes at a rate of 0.1 ml/minute, 25%–33% of the normal CSF production rate (10). CSF samples were frozen on dry ice at the bedside. Blood was drawn at intervals from a heparin lock that had been placed in a vein in the dominant arm the previous evening. Normal saline solution was infused (100 ml/hour) throughout the experiment by means of a catheter that had been inserted the night before into an antecubital vein in the nondominant arm.
Collection of 24-hour urine for determination of urinary-free cortisol levels
Urine was collected for 24 hours beginning at 6:00 a.m. the day of the CSF withdrawal procedure. The urine was preserved on ice during the collection. Immediately after the 24-hour collection was completed, the urine was transported to the Cincinnati VA Medical Center Clinical Laboratory for assay of free cortisol.
Hormone assays
All CSF samples were stored at –80°C until immediately before hormone radioimmunoassay. Hourly CSF samples were assayed for CRH in duplicate for each patient. CRH was assayed by modifications of the method of Nicholson et al. (11), as previously described (3). The intra-assay coefficient of variation for the CRH radioimmunoassay was 7.2%, and the interassay coefficient of variation was 11.9%. Cortisol was also quantified by radioimmunoassay. The interassay coefficient of variation for urinary-free cortisol levels was 21%.
Statistical Analysis
Statistics were computed on Statistica software (StatSoft, Tulsa, Okla.). Repeated measures analysis of variance (ANOVA), paired and unpaired t tests, and regression analyses were used, as appropriate. Pearson coefficients were used in all correlation analyses; p values of <0.05 were considered to be significant.
RESULTS
By using a three-way repeated measure ANOVA (diagnostic group by smoking status by CRH concentrations at seven time points), we found no interaction effects. There were two statistically significant main effects. CSF CRH concentrations were significantly higher in the patients with PTSD than in the normal volunteers (55.2 pg/ml, SD=16.4, versus 42.3, SD=15.6) (F=4.74, df=1, 16, p<0.04). Mean CSF CRH concentrations were lower in nonsmokers than in smokers (43.7 pg/ml, SD=13.0, versus 52.1, SD=19.2), but the difference was not significant (F=–1.12, df=1, 16, p<0.31).
In addition, there was a significant CSF CRH time main effect (figure 1), which remained significant after the Huynh-Feldt correction was used (F=2.39, df=5.23, 83.67, p<0.05). Three subjects, one comparison subject and two patients, were not included in the ANOVA because of missing time point concentrations. Conclusions did not change when all 23 subjects were used in a simple two-way factorial (diagnosis by smoking) in which concentration responses were averaged over time.
No significant difference in 24-hour urinary-free cortisol excretion was evident between normal volunteers and patients with PTSD. Mean urinary-free cortisol excretion was 76.2 µg/24 hours (SD=19.7) in normal subjects and 84.4 µg/24 hours (SD=55.3) in patients with PTSD (t=–0.40, df=17). Although the mean 24-hour urinary-free cortisol excretion was higher in the smokers (97.5 pg/ml, SD=57.3) than the nonsmokers (67.4 pg/ml, SD=19.7), the difference was not significant (t=–1.56, df=17).
No significant relationship between mean CSF CRH concentrations and mean 24-hour urinary-free cortisol excretion was observed (r=–0.08, df=17).
There was no significant correlation found between mean CSF CRH concentrations and overall PTSD severity, as measured by total scores on the Clinician-Administered PTSD Scale (r=–0.04, df=10), in the PTSD patients. Similarly, no significant correlations were observed between CSF CRH and the intrusion (r=–0.35, df=10), avoidance (r=–0.08, df=10), or hyperarousal (r=0.39, df=9) subscales of the Clinician-Administered PTSD Scale. The correlation between CSF CRH concentrations and depression, as measured by the Hamilton Depression Rating Scale, was positive but not significant (r=0.35, df=10). However, with removal of an outlier (a dysthymic patient with a Hamilton depression score of 22), a significant positive correlation was observed (r=0.63, df=9, p=0.05).
The correlation between 24-hour urinary-free cortisol excretion and PTSD symptoms, as measured by the total score on the Clinician-Administered PTSD Scale, was negative and significant (r=–0.72, df=9, p<0.02). Correlations between the 24-hour excretion of urinary-free cortisol and subscale scores on the Clinician-Administered PTSD Scale were negative and significant for the intrusion (r=–0.66, df=9, p<0.05) and hyperarousal (r=–0.65, df=9, p<0.05) subscales and were negative and approaching significance for the avoidance subscale (r=–0.55, df=9, p=0.10). There was no significant correlation between 24-hour urinary-free cortisol and Hamilton depression scores (r=–0.10, df=9).
DISCUSSION
We obtained serial CSF samples over 6 hours and observed higher CSF CRH concentrations in combat veterans with PTSD than in healthy subjects. None of our patients was depressed at the time of the study, all had been abstinent from drugs of abuse and alcohol for at least 6 months before the study, and six of the 11 had never abused drugs or alcohol. That CSF CRH levels were elevated in our patients with a history of alcoholism is all the more striking because we had previously noted very low CSF CRH levels in abstinent male alcoholics (9). Thus, our finding supports the recent results of Bremner et al. (2), who obtained CSF by means of a single morning lumbar puncture and also found elevated CSF CRH levels in a similar cohort of patients with PTSD, and extends them by controlling for the stress of needle insertion and by sampling over a period of 6 hours.
Our past study (6) suggests that smokers have lower CSF CRH concentrations than nonsmokers after 11–17 hours of abstinence. The data from the present study are generally consistent with these previous findings (although few normal volunteer cigarette smokers were included in the present cohort). It is of interest that carbon monoxide (found in cigarette smoke) has been shown to be a negative regulator of hypothalamic CRH in vitro (12). Accordingly, direct comparison of nonsmoker normal subjects with nonsmoker PTSD patients was performed. When only nonsmokers were compared, normal subjects had CSF CRH concentrations of only 42.5 pg/ml (SD=15.0), whereas the PTSD patients had CSF CRH concentrations of 67.5 pg/ml (SD=15.1).
CSF CRH concentrations presumably reflect the integrated effects of CRH synthesis and release, enzymatic degradation, and clearance into the plasma from CSF (which is unidirectional in the case of CRH) (4). We cannot determine the status of these respective influences on CSF CRH levels in our patients and normal subjects from the data at hand.
CRH in the lumbar spinal canal is largely extrahypothalamic in origin, and its concentrations have not shown significant correlations with plasma cortisol concentrations (5, 6). Therefore, the inability in the present study to discern a significant relationship between mean CSF CRH levels and 24-hour urinary-free cortisol excretion was not surprising.
As a group, our PTSD patients had normal 24-hour urinary-free cortisol excretion, a finding compatible with the normal to low 24-hour urinary-free cortisol levels reported in all previous studies (13–16) except one (17). In addition, we observed a significant negative correlation between 24-hour urinary-free cortisol excretion and PTSD symptoms, a finding consistent with the results of most prior investigations. While Smith et al. (1) found a positive correlation between 8:00 a.m. plasma cortisol levels and PTSD symptoms, other studies, including those that quantified urinary-free cortisol excretion (15), 8:00 a.m. plasma cortisol (16, 18), or salivary cortisol (19, 20), all demonstrated an inverse correlation between cortisol concentrations and PTSD symptoms.
In summary, using serial CSF sampling, we found high basal CSF CRH concentrations and normal 24-hour urinary-free cortisol excretion in combat veterans with PTSD, a combination of findings that appears to be unique among psychiatric conditions studied to date. It will prove of interest to characterize the responsivity of CSF CRH to provocative challenge (evocation of symptoms) in patients with PTSD.
Received Nov. 26, 1997; revisions received June 9 and Sept. 30, 1998; accepted Nov. 19, 1998. From the Psychiatry Service, Cincinnati VA Medical Center; the Department of Psychiatry, University of Cincinnati College of Medicine; and the Department of Medicine, Vanderbilt University Medical Center, Nashville, Tenn. Address reprint requests to Dr. Baker, PTSD Unit-7E, Cincinnati VA Medical Center, 3200 Vine St., Cincinnati, OH 45220; [email protected] (e-mail). Supported by VA Central Office Research Funds (Dr. Geracioti). The authors thank Renee Hanna, Matthew Wortman, and the Cincinnati VA Medical Center Psychiatric Nursing Staff for their technical support and Eugene C. Somoza, M.D., and Rose A. Maxwell, Ph.D., for statistical consultation.
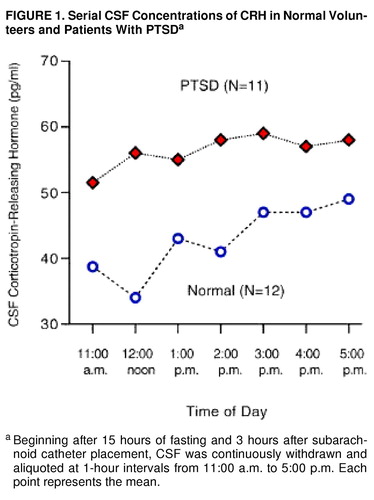
FIGURE 1. Serial CSF Concentrations of CRH in Normal Volunteers and Patients With PTSDa
aBeginning after 15 hours of fasting and 3 hours after subarachnoid catheter placement, CSF was continuously withdrawn and aliquoted at 1-hour intervals from 11:00 a.m. to 5:00 p.m. Each point represents the mean.
1. Smith MA, Davidson J, Ritchie JC, Kudler H, Lipper C, Nemeroff CB: The corticotropin-releasing hormone test in patients with posttraumatic stress disorder. Biol Psychiatry 1989; 26:349–355Crossref, Medline, Google Scholar
2. Bremner JD, Licinio J, Darnell A, Krystal JH, Owens MJ, Southwick SM, Nemeroff CB, Charney DS: Elevated CSF corticotropin-releasing factor concentrations in posttraumatic stress disorder. Am J Psychiatry 1997; 154:624–629Link, Google Scholar
3. Geracioti TD, Orth DN, Ekhator NN, Blumenkoph B, Loosen PT: Serial cerebrospinal fluid corticotropin-releasing hormone concentrations in healthy and depressed humans. J Clin Endocrinol Metab 1992; 74:1325–1330Medline, Google Scholar
4. Martins JM, Banks WA, Kastin J: Acute modulation of active carrier-mediated brain-to-blood transport of corticotropin-releasing hormone. Am J Physiol 1997; 272:E312–E319Google Scholar
5. Kling MA, DeBellis MD, O’Rourke DK, Listwak SJ, Geracioti TD, McCutcheon IE, Kalogeras KT, Oldfield EH, Gold PW: Diurnal variation of cerebrospinal fluid immunoreactive corticotropin-releasing hormone levels in healthy volunteers. J Clin Endocrinol Metab 1994; 79:233–239Medline, Google Scholar
6. Geracioti TD, Loosen PT, Orth DN: Low cerebrospinal fluid corticotropin-releasing hormone concentrations in eucortisolemic depression. Biol Psychiatry 1997; 42:166–174Crossref, Google Scholar
7. Blake DD, Weathers FW, Nagy LN, Kaloupek DG, Gusman FD, Charney DS, Keane TM: The development of a clinician-administered PTSD scale. J Trauma Stress 1995; 8:75–90Crossref, Medline, Google Scholar
8. Geracioti TD, Nicholson WE, Orth DN, Ekhator NN, Loosen PT: Cholecystokinin in human cerebrospinal fluid: concentration, dynamics, molecular forms, and relationship to fasting and feeding in health, depression and alcoholism. Brain Res 1993; 692:260–268Crossref, Google Scholar
9. Geracioti TD, Loosen PT, Ebert MH, Ekhator N, Burns D, Nicholson WE, Orth DN: Concentrations of corticotropin-releasing hormone, norepinephrine, MHPG, 5-hydroxyindoleacetic acid, and tryptophan in the cerebrospinal fluid of alcoholic patients: serial sampling studies. Neuroendocrinology 1994; 60:635–642Crossref, Medline, Google Scholar
10. White RJ, Dakters JG, Yashon D, Albin MS: Temporary control of cerebrospinal fluid volume and pressure by means of an externalized valve-drainage system. J Neurosurg 1969; 30:264–269Crossref, Medline, Google Scholar
11. Nicholson WE, Davis DR, Sherrel BJ, Orth DN: Rapid radioimmunoassay for corticotropin-releasing factor. Pharmacol Rev 1991; 43:425–473Medline, Google Scholar
12. Parkes D, Kasckow JW, Vale WW: Carbon monoxide modulates secretion of corticotropin-releasing factor from rat hypothalamic cultures. Brain Res 1994; 646:315–318Crossref, Medline, Google Scholar
13. Mason JW, Giller EL, Kosten TR, Ostroff RB, Podd L: Urinary free cortisol levels in post-traumatic stress disorder patients. J Nerv Ment Dis 1986; 174:145–149Crossref, Medline, Google Scholar
14. Yehuda R, Southwick SM, Nussbaum G, Wahby V, Giller EL, Mason JW: Low urinary cortisol excretion in patients with posttraumatic stress disorder. J Nerv Ment Dis 1990; 178:366–369Crossref, Medline, Google Scholar
15. Yehuda R, Kahana B, Binder-Brynes K, Southwick SM, Mason JW, Giller EL: Low urinary cortisol excretion in Holocaust survivors with posttraumatic stress disorder. Am J Psychiatry 1995; 152:982–986Link, Google Scholar
16. Yehuda R, Teicher MH, Trestman RL, Levengood RA, Siever LJ: Cortisol regulation in posttraumatic stress disorder and major depression: a chronobiological analysis. Biol Psychiatry 1996; 40:79–88Crossref, Medline, Google Scholar
17. Pitman RK, Orr SP: Twenty-four hour cortisol and catecholamine excretion in combat-related posttraumatic stress disorder. Biol Psychiatry 1990; 27:245–247Crossref, Medline, Google Scholar
18. Boscarino JA: Posttraumatic stress disorder, exposure to combat, and lower plasma cortisol among Vietnam veterans: findings and clinical implications. J Consult Clin Psychology 1996; 64:191–201Crossref, Medline, Google Scholar
19. Geonjian AK, Yehuda R, Pynoos RS, Steinberg AM, Tashjian M, Yang RK, Najarian LM, Fairbanks LA: Basal cortisol, dexamethasone suppression of cortisol, and MHPG in adolescents after the 1988 earthquake in Armenia. Am J Psychiatry 1996; 153:929–934Link, Google Scholar
20. Kellner M, Baker DG, Yehuda R: Salivary cortisol in Operation Desert Storm returnees. Biol Psychiatry 1997; 42:849–850Crossref, Medline, Google Scholar