Cocaine, Ethanol, and Genotype Effects on Human Midbrain Serotonin Transporter Binding Sites and mRNA Levels
Abstract
OBJECTIVE: Earlier platelet and postmortem brain studies have found alterations in serotonin transporter function in ethanol-abusing human subjects. The present investigation tested the hypothesis that brain serotonin transporter function is altered in chronic users of ethanol and cocaine, which might be related to a common serotonin transporter promoter polymorphism. METHOD: Serotonin transporter binding sites, serotonin transporter mRNA levels, and serotonin transporter promoter variants were quantified in postmortem samples from a group of human subjects who had been ethanol users or cocaine users and then compared to those of a matched group of comparison subjects. Quantitative autoradiographic and in situ hybridization assays were performed in midbrain samples that contained the dorsal and median raphe nuclei (the location of serotonin cell bodies that innervate the forebrain). RESULTS: There was a significant overall cocaine-by-ethanol-by-genotype interaction. Dorsal raphe [125I]CIT binding to the serotonin transporter was lower in cocaine users than in comparison subjects. In addition, serotonin transporter binding and serotonin transporter mRNA levels varied significantly by genotype. It was also found that serotonin transporter binding in subjects with either the short or heterozygote genotype was significantly higher in the ethanol-user subjects. CONCLUSIONS: Serotonin transporter binding sites were regulated in a region-specific and substance-specific pattern, which was not simply a local response to functional blockade. Also, a reciprocal relationship appeared to exist between cocaine and ethanol effects in the dorsal raphe, which may have interesting clinical implications for dual-diagnosis patients. It is possible that serotonin transporter promoter genotype may play a complex role in chronic ethanol dependence. (Am J Psychiatry 1998; 155:207–213)
Serotonin neuronal dysfunction is implicated in several substance abuse and clinical disorders, including alcoholism (1), depression (2), suicidality (3), and impulsivity (4). Increased imipramine binding to the serotonin transporter has been detected in hippocampal samples from deceased subjects with evidence of ethanol exposure (5) and considerable evidence suggests that changes occur in platelet serotonin transporter function in alcoholics (6–8). The neurochemical and clinical implications of these findings remain uncertain, but recent evidence indicates that alterations in monoamine transporter function can significantly modulate overall neuronal function (9, 10).
Cocaine acutely blocks the uptake of both serotonin and dopamine by binding to the transporters specific for each neurotransmitter. Although the effect of cocaine on dopamine uptake is critical for reward, animal experiments suggest that acute blockade of serotonin transporter function may also contribute to clinical symptoms such as euphoria, anxiety, and craving (11, 12). Chronic cocaine exposure increases binding sites on the dopamine transporter in the striatum of human users (13, 14), perhaps as a compensation for chronically diminished function. This effect might similarly occur with the serotonin transporter.
Recent experiments have identified a common serotonin transporter genomic variant in humans, located in the serotonin transporter promoter region (15). This polymorphism reportedly decreases expression of serotonin transporter in peripheral lymphoblast cells and might predispose an individual to ethanol- or cocaine-induced alterations in serotonin transporter function, or even to initial ethanol or cocaine abuse.
In the present investigation, we tested the hypothesis that alterations in brain serotonin transporter exist in chronic users of ethanol and cocaine. Serotonin transporter binding sites and serotonin transporter mRNA levels were quantified in postmortem samples from several groups of human subjects who chronically abused ethanol, cocaine, or both. Serotonin transporter binding sites were quantitated by radioligand autoradiography and serotonin transporter mRNA was quantitated by in situ hybridization in midbrain samples containing dorsal and median raphe nuclei (the location of serotonin cell bodies that innervate the forebrain). Serotonin transporter promoter genotype was also determined for each subject.
METHOD
Subjects
Postmortem brain specimens were obtained at autopsy as authorized by the Office of the Wayne County Medical Examiner, Detroit, and stored at –70°C. The same autopsy process was used for each of the 64 subjects, all of whom had died suddenly from various causes (table 1). There were four groups of subjects: drug-free comparison subjects (N=21), chronic cocaine-using subjects (N=17), chronic ethanol-using subjects (N=12), and subjects who used both cocaine and ethanol (N=14). Subjects described as cocaine users or ethanol users met DSM-IV criteria for either dependence or abuse except in a few cases where sufficient diagnostic information was not available, but chronic use was documented. Each cocaine user displayed cocaine on toxicological analyses. In five subjects with a diagnosis of ethanol dependence, ethanol was not detected at autopsy. Conversely, three cocaine-using subjects were found with ethanol on toxicology, but other evidence indicated these subjects were not ethanol dependent. Some cocaine users not diagnosed as ethanol dependent may have occasionally used ethanol. All diagnostic categorizations were assigned before assays were performed. To simplify interpretation, subjects who had committed suicide were not included (3).
Subjects were matched for postmortem interval and age as shown in table 1. The majority of subjects were male (comparison subjects, 67% [N=14]; ethanol users, 92% [N=11]; cocaine users, 82% [N=14]; users of both ethanol and cocaine, 79% [N=11]) and African American (comparison subjects, 67% [N=14]; ethanol users, 67% [N=8]; cocaine users, 88% [N=15]; users of both ethanol and cocaine, 71% [N=10]). Subjects had similar socioeconomic backgrounds, as quantitated by Hollingshead scale (16) scores: comparison subjects, mean=3.2 (SD=0.3); cocaine users, mean=4.4 (SD=0.2), ethanol users, mean=4.0 (SD=0.3), users of both ethanol and cocaine, mean=4.3 (SD=0.3).
Subjects did not have histories of serious medical illnesses, reflecting the fact that medical examiner autopsies are performed in natural deaths only when serious antecedent illnesses have not been previously discovered. Those with substance abuse exhibited a range of typical comorbid psychiatric diagnoses (table 1), including cocaine-induced mood disorder (N=7), ethanol-induced mood disorder (N=1), cocaine-induced psychosis (N=3), schizoaffective disorder (N=1), and history of childhood attention deficit disorder (N=1). In addition, seven cocaine-using subjects were also diagnosed as opioid abusers. Drug-using subjects (N=43) were chosen from a larger pool of 55 potential subjects on the basis of availability of midbrain blocks and clinical information. Comparison subjects (N=21) were chosen from 75 potential comparison subjects, and met the following criteria: 1) suitable cause of death (rapid, not result of chronic condition); 2) appropriate age, sex, and race to match drug-using subjects; and 3) absence of DSM-IV diagnoses.
Clinical Assessment
A research psychiatrist and a social worker (K.Y.L. and C.S.L.) interviewed at least one informant for each subject, usually a relative. Other types of informants included neighbors, physicians, other mental health professionals, fellow workers, police, medical examiners, and newspaper reporters. Family members who participated in the study provided informed consent as approved by the Institutional Review Board of the University of Michigan. The DSM-IV criteria for psychoactive substance abuse disorders, mood disorders, psychotic disorders, and anxiety disorders were explicitly reviewed with informants. Also, the Family History Research Diagnostic Criteria (17) checklists of symptoms for mood disorders, alcoholism and antisocial personality, anxiety disorders, and psychotic disorders, the Hamilton Depression Rating Scale, and the Michigan Alcohol Screening Test (18) were completed. Later, based on all available evidence and relying most heavily on data from relatives and toxicological results, DSM-IV psychiatric diagnoses were assigned at a consensus conference.
Toxicology. In order to provide information for diagnostic categorization, urine or serum from subjects was assayed qualitatively for the presence of stimulants, anxiolytics, marijuana, antidepressants, and antipsychotics, by using a variety of methods including radioimmunoassay, high-performance liquid chromatography, and gas chromatography-mass spectrometry. Because the assays sometimes were performed on different types of samples and were not always performed quantitatively, toxicological levels could not be correlated with neurochemical data.
Laboratory Assays
Autoradiographic binding assays. The autoradiographic assay methods for the high-affinity serotonin transporter radioligand [125I]2β-carboxylic acid methyl ester-3β-(4-iodophenyl)tropane ([125I]CIT), also known as [125I]RTI-55, have been previously described for human brain (19). Incubations were performed at 22°C in TRIS buffer (50 mM, pH 7.4) with 120 mM NaCl for 4 hours, using a saturating concentration (3.5 nM) of [125I]CIT (specific activity 2200 Ci/mmol, New England Nuclear/Dupont). Earlier work in human midbrain found a single binding site with high affinity, Kd=0.37+0.08 nM (19). Because the concentration of [125I]CIT used was about 10 times its single site Kd, binding at this concentration likely reflects the total number of binding sites (Bmax). However, two serotonergic [125I]CIT binding sites have been reported in occipital cortex, one with a low affinity Kd of 4.2 nM. If applicable to midbrain, this suggests that affinity changes could also alter binding at 3.5 nM (20). Nonspecific binding for [125I]CIT was determined by the addition of 100 nM citalopram. Because of its selectivity for the serotonin transporter versus dopamine transporter (about 100,000x), citalopram (100 nM) blocks binding to 99% of serotonin transporter sites, but to only 3% of dopamine transporter sites (based on Ki in reference 19).
Slides were washed in successive TRIS buffer baths (two for 10 minutes each) and a deionized water bath (2 minutes), then apposed to Kodak SB-5 film for 5–7 days. Optical densities were determined by means of an MCID image analysis system (at the level demonstrated in reference 19 [figure 6]). Midbrain topography was identified by using the atlases of van Domburg and ten Donkelaar (21) and Olszewski and Baxter (22). Optical densities were evaluated by comparison to co-exposed commercial standards (Amersham, 125I microscale standards) and expressed in fmol/mg tissue, based on the conversion data supplied by the manufacturer.
In situ hybridization. The in situ hybridization protocol used for human midbrain has been described previously (23, 24). Briefly, sections were fixed in 4% paraformaldehyde for 1 hour, pretreated with proteinase K to facilitate probe penetration into the tissue, and acetylated with 0.25% acetic anhydride (in 0.1 M triethanolamine, pH 8). cRNA probes were transcribed in vitro from cDNAs cloned into plasmid vectors, using T7 and T3 RNA polymerases to generate 35S-radiolabeled cRNA probes of high specific activity. Following overnight hybridization (55°C), sections were treated with RNase to degrade unhybridized probe, washed under stringent conditions (65°C in low salt buffer—0.1 XSSC), and dehydrated. Sections were apposed to Kodak X-OMAT AR X-ray film, along with 14C-radiolabeled standards (Amersham); the film was developed after 10–12 days. Treatment of control sections with RNase A prior to hybridization or with “sense” strand cRNA probes resulted in minimal signal. Hybridization in the dorsal raphe, median raphe, and B9 regions were quantified at the level demonstrated in McLaughlin et al. (23). To control for nonspecific degradation, mRNA for cyclophillin was measured (24, 25) and serotonin transporter mRNA levels for each individual expressed as a ratio to cyclophillin mRNA levels. Cyclophillin is a ubiquitous cell structural protein not related to serotonin transporter function.
Genotyping. DNA was extracted from postmortem brain tissue by using the Pure Gene extraction kit (Gentra). Oligonucleotide primers flanking the serotonin transporter polymorphic region and corresponding to the nucleotide positions that ranged from –1403 to –1384 and from –973 to –954 of the serotonin transporter regulatory region were used to generate fragments with 406 or 450 base pairs (26, 27). Polymerase chain reaction was then performed in a 10 µl volume containing template, nucleotides, Taq polymerase (AmpliTaq, Perkin Elmer), and polymerization buffer, employing a Perkin Elmer GeneAmp PCR System 9600, through 40 cycles. PCR product was then separated at room temperature on 4% agarose gels.
Data Analysis
[125I]CIT binding and mRNA levels were initially compared among groups by use of multivariate analysis of variance (MANOVA) because data from the dorsal raphe, median raphe, and substantia nigra were related measures within individuals and appeared to demonstrate similar responses to ethanol, cocaine, and genotype. The initial MANOVA also served to direct further lower-order analyses and limit the total number of statistical tests employed (28). A three-factor model was constructed to examine the interactive effects of cocaine, ethanol, and genotype on [125I]CIT binding (dorsal raphe, median raphe, substantia nigra) and serotonin transporter mRNA levels (dorsal raphe, median raphe, B9 region). Serotonin transporter binding was not quantified in the B9 region; serotonin transporter mRNA was not present in the substantia nigra. Simpler models were used to examine two-factor interactions, and then univariate factors were progressively examined, as indicated. Because of inhomogeneity of variance, binding and mRNA levels were log transformed before parametric analysis. The incidence of the various genotypes in the different clinical categories was examined by chi-square.
A one-way analysis of variance (ANOVA) was used to examine the groups for differences in age and postmortem interval. Age and postmortem interval data were also examined for correlation with both binding and mRNA levels. Subjects were examined for race, sex, or genotype differences in binding or mRNA levels across diagnoses by multiway ANOVA. In addition, binding and mRNA levels were examined for correlation with each other by using Pearson's product moment within each region.
RESULTS
Figure 1 (nontransformed values) displays the overall effects of cocaine and ethanol on binding in the three midbrain regions. Significant cocaine-by-ethanol-by-genotype interactions were found in the initial MANOVA analysis by using the raw or log-transformed data (Wilks's lambda=0.86, F=2.66, df=3,49, p=0.03). [125I]β-CIT binding in cocaine users appeared to be lower in all three regions, but reached statistical significance only in the dorsal raphe: dorsal raphe (mean=4.0 fmol/mg, SD=0.6, for comparison subjects, versus mean=2.1 fmol/mg, SD=0.3, for cocaine users) (t=2.51, df=32, p=0.02); median raphe (mean=2.9 fmol/mg, SD=0.6, for comparison subjects, versus mean=1.9 fmol/mg, SD=0.3, for cocaine users) (t=1.76, df=32, p=0.09); and substantia nigra (mean=2.3 fmol/mg, SD=0.5, for comparison subjects, versus mean=1.2 fmol/mg, SD=0.2, for cocaine users) (t=1.80, df=32, p=0.08). The apparent difference between comparison subjects and ethanol users in dorsal raphe was not statistically significant (mean=4.0 fmol/mg, SD=0.6, for comparison subjects, versus mean=6.4 fmol/mg, SD=1.6, for the ethanol users) (t=1.63, df=27, p=0.11), possibly because variability was high and the effect was influenced by genotype. In contrast to serotonin transporter binding sites, there were no drug abuse effects on serotonin transporter mRNA levels.
There was a consistent genotype effect on both serotonin transporter binding and mRNA levels across regions (figure 2). For this part of the study, the ethanol users were excluded because they displayed a genotype-by-ethanol interaction. Serotonin transporter binding varied significantly by genotype and region (two-way ANOVA, genotype-by-region, genotype main effect, F=8.02, df=1,87, p<0.001; region, F=5.75, df=1,87, p=0.005, no significant interaction). Serotonin transporter binding in subjects with the heterozygous genotype was clearly lower than in subjects with the long genotype, while subjects with the short genotype appeared equivalent to long genotype, perhaps because of the small number of subjects.
Serotonin transporter mRNA levels also varied significantly by genotype, but not by region (two-way ANOVA, genotype-by-region, genotype main effect, F=8.28, df=1,67, p<0.001; region, F=1.64, df=1,67, n.s.). Serotonin transporter mRNA levels in subjects with the mixed and short genotypes were clearly lower than in subjects with the long genotype.
There was a statistically significant ethanol-by-genotype interaction found by MANOVA which was limited to the dorsal raphe in the midbrain areas examined for this study. [125I]CIT binding in subjects with either the short or mixed genotype was significantly higher in ethanol-using subjects than in comparison subjects (mean=7.2 fmol/mg, SD=1.5, versus mean=2.7 fmol/mg, SD=0.6) (t=2.97, df=25, p=0.006, two-tailed). Values were similar in ethanol users and comparison subjects with the long variant (mean=3.5 fmol/mg, SD=0.5, versus mean=3.6 fmol/mg, SD=0.6) (n.s.). In subjects without ethanol diagnoses, 8% (N=4) had the short genotype; 17% (N=2) of the ethanol-user subjects had the short genotype. Inspection of the clinical data on the short- versus long-genotype ethanol users did not reveal any distinguishing features. Several individuals were diagnosed with antisocial personality in each group and Hamilton scores were similar for those individuals who scored any points. There were no cocaine interactions with genotype, nor any interactive cocaine effects on mRNA levels.
Genotype frequencies for the four groups were as follows: comparison subjects—three unable to amplify, three short, six mixed, nine long; ethanol-using subjects—two short, five mixed, five long; cocaine-using subjects—one short, seven mixed, nine long; and for subjects who were users of both cocaine and ethanol—no short, six mixed, eight long. The frequency of the long allele ([(homozygous long N×2)+mixed N] divided by (total N×2), where N is the number of subjects) did not differ significantly among the subject groups: comparison subjects, 67% ethanol-using subjects, 62% cocaine-using subjects, 73% and subjects who were users of both cocaine and ethanol, 79% (χ2=1.87, df=3, p=0.60). However, our total numbers were small and our case-control study design is imperfect for testing for possible association between genotype and clinical predilection to either cocaine or ethanol abuse.
Binding and mRNA levels were not significantly correlated with each other in the same regions within individuals of the same group. There were no significant differences in age or postmortem interval among the various groups, and neither binding nor mRNA levels correlated with these parameters. No significant race, socioeconomic, or sex differences were discovered. Neither binding nor mRNA levels were different in subjects with mood disorder or psychotic diagnoses, or in subjects with opioid abuse diagnosis, but the statistical power for these analyses was limited.
DISCUSSION
The present findings are consistent with one earlier postmortem human study (5), which found increased [3H]imipramine binding in hippocampal dentate and pyramidal cell layers from subjects who had died after acute ethanol exposure. Animal experiments in alcohol-preferring strains of rats have reported diffusely increased binding to the serotonin transporter, although not all studies found this effect (29, 30). One report found no basal differences between strains, but noted that ethanol exposure induced increased serotonin transporter binding in several brain regions (31). Together, these earlier experiments suggest that ethanol use or a predisposition to use may involve brain serotonin transporter.
No previous studies have explored cocaine effects in postmortem human brain. Cunningham et al. (32) reported increased [3H]imipramine binding in medial prefrontal cortex, frontal cortex, sulcal prefrontal cortex, and dorsal raphe nuclei of rats treated with cocaine (15 mg i.p. b.i.d. for 7 days). In addition, Belej et al. (33) reported that oral self-administration of cocaine in rats increased [3H]paroxetine binding in three of 53 regions, including by 21% in the accumbens. These rat studies, in view of our results, tentatively suggest that cocaine effects on the serotonin transporter may be different in humans; however, dosing amounts and schedules may also contribute to differing results. This is clearly the case with cocaine's effect on the dopamine transporter (13, 34, 35).
Several anatomical issues complicate interpretation of the present results. Serotonin transporter function in the substantia nigra and the two raphe nuclei may have distinct overall effects on serotonergic signaling. In the substantia nigra, serotonin transporter molecules are axonally located and might influence postsynaptic signaling by modulating synaptic serotonin concentrations. In contrast, serotonin transporter molecules in the raphe are somatodendritic and represent collateral innervation from proximal and distal serotonergic neurons. Somatodendritic transporters might be expected to contribute to inhibition and synchronization of cell firing rates. Because of this, an increase in somatodendritic serotonin transporter function, suggested by increased dorsal raphe [125I]CIT binding in alcoholics with the short genotype, might serve to increase serotonin firing rates by diminishing self-inhibition. Conversely, in cocaine-using subjects, a decrease in dorsal raphe serotonin transporter activity might decrease serotonergic firing, through increased self-inhibition, while a similar decrease in serotonin uptake in the substantia nigra would tend to promote serotonergic signaling by delaying the clearance of synaptic serotonin. To better reconcile these issues considerably more information is needed about the status of other perisynaptic factors also critically involved, especially release rates and pre- and postsynaptic receptor sensitivities.
The data from this study represent the second reciprocal relationship we have detected between dopamine and serotonin parameters in cocaine users. In earlier experiments, we detected increased striatal dopamine transporter binding, and in a different group of subjects, higher frontal cortex serotonin and lower dopamine levels, than in comparison subjects (36). In the future it would be desirable to measure both monoamine transporter binding levels and neurotransmitter levels from the same groups. A useful feature of postmortem studies is the possibility of examining a number of parameters in samples from contiguous sections in the same individual.
The present genotype results appear generally consistent with those of Lesch et al. (15), who examined binding, mRNA levels, and uptake in lymphoblasts from nine individuals. Lesch et al. found that heterozygote genotypes were best classified with the short variants, which was also the case for serotonin transporter mRNA levels (but not serotonin transporter binding) in the present study. However, the apparently elevated binding among those subjects with the short variant was influenced by one very high value, and might simply reflect too small a sample. It is also possible, however, that there is a true difference between heterozygotes and either type of homozygote. Unlike Lesch et al., we were not able to examine actual serotonin uptake, which we have found is not satisfactorily recoverable in frozen samples because of variable postmortem degradation.
The serotonin transporter polymorphism may be important clinically and one can anticipate an interest in further screening of alcoholic patients and depressed patients, particularly those refractory to treatment, as well as screening of other populations suspected of serotonin transporter abnormalities, such as those with autistic disorder (37). Both genotype and peripheral serotonin transporter binding can be fairly easily explored in peripheral tissue, although it is possible that critical adaptations only occur in certain brain regions.
The detection of genotype, ethanol, and cocaine effects in this relatively small and complex population suggests that each of the effects may be fairly robust. Fortuitously, cocaine and ethanol alterations were in opposite directions, allowing us to distinguish their differing effects. However, the size of these groups was rather small and caution should be exercised in extending these findings until further replication occurs. Confidence in the findings would be increased if the number of subjects had been larger, more extensive sampling were done in these regions, and other brain regions were examined. Also, our clinical classification may contain some errors because of the nature of the clinical data. In particular, it may be that ethanol dependence could have been missed in some cocaine users, although efforts were made to prevent this. An ideal reclassification might actually increase the apparent cocaine effect since there were some high values in subjects using only cocaine who might have been more accurately included in the users of both cocaine and ethanol category.
In conclusion, it appears that regulation of serotonin transporter binding sites is a dynamic process, with distinct region- and substance-specific patterns that are more than local responses to functional blockade. There may be a reciprocal relationship between cocaine and ethanol effects in the dorsal raphe, perhaps clinically important for patients that are dually dependent. In sum, the present data suggest that a better understanding is needed of 1) the time course and doses involved in cocaine- and ethanol-induced changes; 2) the range of brain regions involved in cocaine, ethanol, and genotype effects; and 3) the clinical features associated with cocaine- and ethanol-regulated serotonin transporter binding, and various serotonin transporter genotypes.
![]() |
Presented in part at the 26th annual meeting of the Society for Neuroscience, Washington, D.C., Nov. 16–21, 1996. Received March 13, 1997; revision received Aug. 7, 1997; accepted Aug. 18, 1997. From the Department of Psychiatry, University of Michigan, Ann Arbor, and the Ann Arbor VA Medical Center. Address reprint requests to Dr. Little at the Psychobiology Laboratory/116-A, Ann Arbor VA Medical Center, 2215 Fuller Rd., Ann Arbor, MI 48105; [email protected] (e-mail). Supported by National Institute on Drug Abuse grants DA-09491 (D r. Little) and DA-02265 (Dr. Watson) and NIMH grant MH-4225 1 and the Scottish Rite Schizophrenia Foundation (Drs. McLaughlin and Watson). The authors thank Yung A. Chung, Suwait KanLuen, Laning Davidson, and Carl Schmidt of the Office of the Wayne County Medical E xaminer, Detroit, and Joe Kontuly and Sherry Becker for their assi stance.
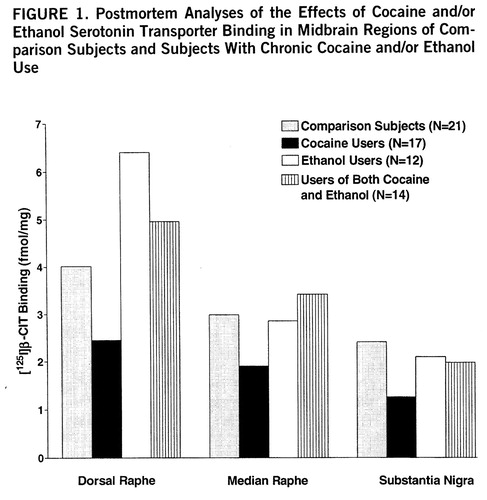
FIGURE 1. Postmortem Analyses of the Effects of Cocaine and/or Ethanol Serotonin Transporter Binding in Midbrain Regions of Comparison Subjects and Subjects With Chronic Cocaine and/or Ethanol Use
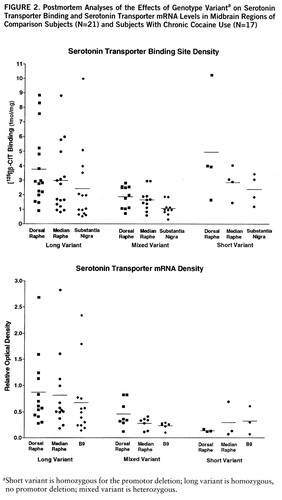
FIGURE 2. Postmortem Analyses of the Effects of Genotype Varianta on Serotonin Transporter Binding and Serotonin Transporter mRNA Levels in Midbrain Regions of Comparison Subjects (N=21) and Subjects With Chronic Cocaine Use (N=17)
aShort variant is homozygous for the promotor deletion; long variant is homozygous, no promotor deletion; mixed variant is heterozygous.
1. Nevo E, Hamon M: Neurotransmitter and neuromodulatory mechanisms involved in alcohol abuse and alcoholism. Neurochem Int 1995; 26:305–336Crossref, Medline, Google Scholar
2. Meltzer HY, Lowy MT: The serotonin hypothesis of depression, in Psychopharmacology: The Third Generation of Progress. Edited by Meltzer HY. New York, Raven Press, 1987, pp 513–526Google Scholar
3. Mann JJ, Underwood MD, Arango V: Postmortem studies of suicide victims, in Biology of Schizophrenia and Affective Disease. Edited by Watson SJ. Washington, DC, American Psychiatric Press, 1996, pp 197–221Google Scholar
4. Virkkunen M, Linnoila M: Brain serotonin, type II alcoholism and impulsive violence. J Study Alcohol 1993; 11:163–169Google Scholar
5. Gross-Isseroff R, Biegon A: Autoradiographic analysis of [3H]imipramine binding in the human brain postmortem: effects of age and alcohol. J Neurochem 1988; 51:528–534Crossref, Medline, Google Scholar
6. Ernouf D, Compagnon P, Lothion P, Narcisse G, Benard JY, Daoust M: Platelets [3H]5-HT uptake in descendants from alcoholic patients: a potential risk factor for alcohol dependence? Life Sci 1993; 52:989–995Google Scholar
7. Bailly D, Vignau J, Racadot N, Beuscart R, Servant D, Parquet P: Platelet serotonin levels in alcoholic patients: changes related to physiological and pathological factors. Psychiatry Res 1993; 47:57–68Crossref, Medline, Google Scholar
8. Rausch JL, Monteiro MG, Marc MA: Platelet serotonin uptake in men with family histories of alcoholism. Neuropsychopharmacology 1991; 4:83–86Medline, Google Scholar
9. Zahniser NR, Gerhardt GA, Cass WA: Chronic cocaine action on the dopamine transporter, in The Neurobiology of Cocaine. Edited by Hammer RP. Boca Raton, Fla, CRC Press, 1995, pp 181–191Google Scholar
10. Giros B, Jaber M, Jones SR, Wightman RM, Caron MG: Hyperlocomotion and indifference to cocaine and amphetamine in mice lacking the dopamine transporter. Nature 1996; 379:606–612Crossref, Medline, Google Scholar
11. Elsworth JD, Taylor JR, Jatlow P, Roth RH: Serotonin involvement in cocaine sensitization: clues from studies with cocaine analogs. Drug Development Res 1993; 30:189–200Crossref, Google Scholar
12. Carroll ME, Lac ST, Asencio M, Kragh R: Intravenous cocaine self-administration in rats is reduced by dietary L-tryptophan. Psychopharmacology (Berl) 1990; 100:293–300Crossref, Medline, Google Scholar
13. Little KY, Kirkman JA, Carroll FI, Clark TB, Duncan GE: Cocaine use increases [3H]WIN 35428 binding sites in human striatum. Brain Res 1993; 628:17–25Crossref, Medline, Google Scholar
14. Staley JK, Hearn L, Ruttenber J, Wetli CV, Mash DC: High affinity cocaine recognition sites on the dopamine transporter are elevated in fatal cocaine overdose victims. J Pharmacol Exp Ther 1994; 271:1678–1685Google Scholar
15. Lesch KP, Bengel S, Heils A, Sabol SZ, Greenberg BD, Petri S, Benjamin J, Muller CR, Hamer DH, Murphy DL: Association of anxiety-related traits with a polymorphism in the serotonin transporter gene regulatory region. Science 1996; 274:1527–1531Google Scholar
16. Hollingshead AB: Two-Factor Index of Social Position. New Haven, Yale University, 1957Google Scholar
17. Endicott J, Andreasen N, Spitzer RL: Family History Research Diagnostic Criteria, 3rd ed. New York, New York State Psychiatric Institute, Biometrics Research, 1978Google Scholar
18. Selzer M: The Michigan Alcoholism Screening Test: the quest for a new diagnostic instrument. Am J Psychiatry 1971; 127:1653–1658Google Scholar
19. Little KY, Kirkman JA, Carroll FI, Breese GR, Duncan GE: [125I]RTI-55 binding to cocaine-specific dopaminergic and serotonergic uptake sites in the human brain. J Neurochem 1993; 61:1996–2006Google Scholar
20. Staley JK, Basile M, Flynn DD, Mash DC: Visualizing dopamine and serotonin transporters in the human brain with the potent cocaine analogue [125I]RTI-55: in vitro binding and autoradiographic characterization. J Neurochem 1994; 62:549–556Crossref, Medline, Google Scholar
21. van Domburg PHMF, ten Donkelaar HJ: The human substantia nigra and ventral tegmental area: a neuroanatomical study with notes on aging and aging diseases. Adv Anat Embryol Cell Biol 1991; 121:1–132Crossref, Medline, Google Scholar
22. Olszewski J, Baxter D: Cytoarchitecture of the Human Brain Stem, 2nd ed. Basel, Switzerland, S Karger, 1982Google Scholar
23. McLaughlin DP, Little KY, Lopez JP, Watson SJ: Expression of serotonin transporter mRNA in human brainstem raphe nuclei. Neuropsychopharmacology 1996; 15:523–529Crossref, Medline, Google Scholar
24. Little KY, McLaughlin DP, Ranc J, Gilmore J, Lopez JF, Watson SJ, Carroll FI, Butts JD: Serotonin transporter binding sites and mRNA levels in depressed persons committing suicide. Biol Psychiatry 1997; 41:1156–1164Google Scholar
25. Danielson PE, Forss-Petter S, Brow MA, Calavetta L, Douglass J, Milner RJ, Sutcliffe JG: p1B15: a cDNA clone of the rat mRNA encoding cyclophillin. DNA 1988; 7:261–267Crossref, Medline, Google Scholar
26. Heils A, Teufel A, Petri S, Seemann M, Bengel D, Balling U, Riederer P, Lesch KP: Functional promoter and polyadenylation site mapping of the human serotonin (5-HT) transporter gene. J Neural Transm 1995; 102:247–254Crossref, Medline, Google Scholar
27. Heils A, Teufel A, Petri S, Stöber G, Riederer P, Bengel D, Lesch KP: Allelic variation of human serotonin transporter gene expression. J Neurochem 1996; 66:2621–2624Google Scholar
28. Hand DJ, Taylor CC: Multivariate Analysis of Variance and Repeated Measures. London, Chapman & Hall, 1987Google Scholar
29. Daoust M, Compagnon P, Legrand E, Boucly P: Ethanol intake and 3H-serotonin uptake, I: a study in fawn-hooded rats. Life Sci 1991; 48:1969–1976Google Scholar
30. Murphy JM, McBride WJ, Lumeng L, Li TK: Contents of monoamines in forebrain regions of alcohol-preferring (P) and non-preferring (NP) lines of rats. Pharmacol Biochem Behav 1987; 26:389–392Crossref, Medline, Google Scholar
31. Daoust M, Chretien P, Moore N, Saligaut C, Lhuintre JP, Boismare F: Isolation and striatal [3H]serotonin uptake: role in the voluntary intake of ethanol by rats. Pharmacol Biochem Behav 1985; 22:205–208Crossref, Medline, Google Scholar
32. Cunningham KA, Paris JM, Goeders NE: Chronic cocaine enhances serotonin autoregulation and serotonin uptake binding. Synapse 1992; 11:112–123Crossref, Medline, Google Scholar
33. Belej T, Manji D, Sioutis S, Barros HMT, Nobrega JN: Changes in serotonin and norepinephrine uptake sites after chronic cocaine: pre- vs post-withdrawal effects. Brain Res 1996; 736:287–296Crossref, Medline, Google Scholar
34. Hitri A, Little KY, Ellinwood EH: Effect of cocaine on dopamine transporter receptors depends on routes of chronic cocaine administration. Neuropsychopharmacology 1996; 14:205–210Crossref, Medline, Google Scholar
35. Koff JM, Shuster L, Miller LG: Chronic cocaine administration is associated with behavioral sensitization and time-dependent changes in striatal dopamine transporter binding. J Pharmacol Exp Ther 1994; 268:277–282Medline, Google Scholar
36. Little KY, Patel UN, Clark TB, Butts JD: Alteration of brain dopamine and serotonin levels in cocaine users: a preliminary study. Am J Psychiatry 1996; 153:1216–1218Google Scholar
37. Cook EH: Autism: review of neurochemical investigations. Synapse 1990; 6:292–308Crossref, Medline, Google Scholar