Synaptic Proteome Compensation and Resilience to Psychosis in Alzheimer’s Disease
Abstract
Objective:
The presence of psychosis in Alzheimer’s disease denotes a phenotype with more rapid cognitive deterioration than in Alzheimer’s disease without psychosis. Discovery of novel pharmacotherapies that engage therapeutic targets for prevention or treatment of Alzheimer’s disease with psychosis would benefit from identifying the neurobiology of resilience to psychosis in Alzheimer’s disease. The primary objective of this study was to determine whether alterations in the synaptic proteome were associated with resilience to psychotic symptoms in Alzheimer’s disease and, if present, were independent of neuropathologic burden.
Method:
Quantitative immunohistochemistry was used to measure multiple neuropathologies in dorsolateral prefrontal cortex from subjects with early and middle-stage Alzheimer’s disease who differed in psychosis status. Synaptic proteins were quantified by liquid chromatography–mass spectrometry in gray matter homogenates from these subjects and from neuropathologically unaffected subjects. The synaptic proteome was similarly evaluated in cortical gray matter homogenate and in postsynaptic density fractions from an APPswe/PSEN1dE9 mouse model of amyloidosis with germline reduction in Kalrn, which has been shown to confer resilience to progression of psychosis-associated behaviors relative to APPswe/PSEN1dE9 alone.
Results:
Subjects resilient to psychotic symptoms in Alzheimer’s disease had higher levels of synaptic proteins compared with those with psychosis and unaffected control subjects. Neuropathologic burden predicted less than 20% of the variance in psychosis status and did not account for the synaptic protein level differences between groups. Reduction of Kalrn in APPswe/PSEN1dE9 mice resulted in higher levels of synaptic proteins in cortical homogenate and normalized protein levels in the postsynaptic density.
Conclusions:
Accumulation of synaptic proteins, particularly those that are enriched in the postsynaptic density, is associated with resilience to psychosis in Alzheimer’s disease. One candidate mechanism for this synaptic proteome compensation is alteration in levels of proteins that facilitate the transport of synaptic proteins to and from the postsynaptic density.
Psychotic symptoms occur in ∼40%–60% of individuals with Alzheimer’s disease (1). The occurrence of psychosis in Alzheimer’s disease is heritable (2), and it identifies a phenotype in which patients experience more rapid cognitive decline and elevated mortality compared with patients with Alzheimer’s disease without psychosis (3). In addition, patients with psychotic symptoms in Alzheimer’s disease exhibit greater functional impairment, are more likely to be institutionalized during illness, and experience higher rates of additional neuropsychiatric disturbances, including aggression, agitation, and depression (3). Current empirically developed treatments for psychosis in Alzheimer’s disease have limited efficacy, do not alter the more rapid disease progression, and are associated with substantial toxicity, including excess mortality (3). Because the annual incidence of psychosis in Alzheimer’s disease is only ∼10% (4), there is a window of opportunity to intervene to prevent psychosis onset. However, capitalizing on this opportunity first requires identifying the underlying neurobiology of resilience to psychosis in Alzheimer’s disease.
The hallmark pathologies of Alzheimer’s disease are fibrillar deposits of amyloid beta and phosphorylated tau. Studies have examined the association of these measures with psychosis in Alzheimer’s disease, and most have found that a lower phosphorylated tau burden is protective (3). However, as a group these studies have several important limitations. For example, past investigations have overrelied on subjects with end-stage disease, despite clinical evidence that the most rapid increase in rates of psychosis in Alzheimer’s disease occurs in early to middle stages (5, 6). In addition, studies often did not utilize uniform histologic procedures or unbiased quantitative measures of neuropathologic burden (7). Importantly, additional core processes contributing to Alzheimer’s disease, such as neuroinflammation, microglial activation (8, 9), and synapse dysfunction (10, 11), have been largely unexamined in relationship to Alzheimer’s disease with psychosis. This omission is particularly glaring given that multiple neuroimaging studies have indicated relative preservation of neocortical synaptic structure and function in Alzheimer’s disease without psychosis relative to Alzheimer’s disease with psychosis, including greater perfusion (12–14) and metabolism (15, 16) and higher gray matter density (17). Finally, only a subset of studies have considered the role of common comorbid pathologies, such as the presence of Lewy bodies, 43-kDa TAR DNA-binding protein (TDP-43) inclusions, and vascular lesions.
We therefore used a combination of immunohistochemical and liquid chromatography/tandem mass spectrometry (LC-MS/MS) approaches to assess levels of multiple neuropathologic and synaptic markers in dorsolateral prefrontal cortex from subjects with early and mid-stage Alzheimer’s disease who differed in psychosis status. Our choice to examine dorsolateral prefrontal cortex was informed by prior findings in this brain area demonstrating that, relative to subjects with psychosis, those without psychosis in Alzheimer’s disease contain lower fibrillar tau aggregation as well as elevated perfusion and metabolism (3). We determined that neuropathologic disease burden accounts for less than 20% of the variance in psychosis status in Alzheimer’s disease, but we detected a novel signature of resilience—accumulation of synaptically expressed proteins—in subjects without psychosis compared both to those with psychosis and to unaffected control subjects. Finally, we confirmed our proteome findings in a murine model of amyloid beta overproduction exhibiting resilience to progression of psychosis-associated behaviors.
Method
Subjects
We studied 140 subjects with Alzheimer’s disease and 12 control subjects (Table 1; see also Appendix 1 in the online supplement). Psychosis was defined as the presence of delusions or hallucinations at any visit.
Immunohistochemistryb | LC-MS/MSc | |||||||||
---|---|---|---|---|---|---|---|---|---|---|
Variable | Alzheimer’s Disease Without Psychosis (N=59) | Alzheimer’s Disease With Psychosis (N=81) | Alzheimer’s Disease Without Psychosis (N=18) | Alzheimer’s Disease With Psychosis (N=38) | Unaffected Controls (N=12) | |||||
Mean | SD | Mean | SD | Mean | SD | Mean | SD | Mean | SD | |
Age (years) | 83.3 | 7.9 | 82.1 | 6.6 | 85.3 | 7.9 | 83.4 | 7.0 | 70.7 | 9.4 |
Age at onset (years) | 74.6 | 13.0 | 72.7 | 10.8 | 77.3 | 8.0 | 75.0 | 7.2 | n/a | n/a |
Duration of illness (years) | 7.6 | 3.2 | 8.6 | 3.6 | 8.0 | 3.2 | 8.4 | 3.4 | n/a | n/a |
Postmortem interval (hours) | 6.6 | 5.1 | 6.5 | 5.3 | 4.9 | 1.5 | 6.9 | 3.9 | 12.2 | 5.9 |
N | % | N | % | N | % | N | % | N | % | |
Sex | ||||||||||
Male | 32 | 54 | 44 | 54 | 10 | 56 | 18 | 47 | 8 | 67 |
Female | 27 | 46 | 37 | 46 | 8 | 44 | 20 | 53 | 4 | 33 |
Braak stage | ||||||||||
0–II | 0 | 0 | 0 | 0 | 0 | 0 | 0 | 0 | 12 | 100 |
III | 9 | 15 | 12 | 15 | 4 | 22 | 2 | 5 | 0 | 0 |
IV | 28 | 47 | 26 | 32 | 7 | 39 | 13 | 34 | 0 | 0 |
V | 22 | 37 | 43 | 53 | 7 | 39 | 23 | 61 | 0 | 0 |
APOE-4 statusd | ||||||||||
Positive | 29 | 49 | 45 | 56 | 8 | 44 | 19 | 50 | n/a | n/a |
Negative | 27 | 46 | 35 | 43 | 10 | 56 | 19 | 50 | n/a | n/a |
Antipsychotic use | ||||||||||
Yes | 4 | 7 | 16 | 20 | 1 | 6 | 9 | 24 | n/a | n/a |
No | 55 | 93 | 65 | 80 | 17 | 94 | 29 | 76 | n/a | n/a |
TABLE 1. Demographic, Clinical, and Tissue Characteristics of Subjects With Alzheimer’s Disease With and Without Psychosis and Unaffected Control Subjects Examined by Immunohistochemistry and LC-MS/MSa
Sample Collection and Neuropathologic Assessment
For all subjects, gray matter samples from the right superior frontal gyrus of the dorsolateral prefrontal cortex were dissected, frozen at −80°C and processed for LC-MS/MS. For Alzheimer’s Disease Research Center subjects, the corresponding left dorsolateral prefrontal cortex was used for immunostaining and further processed for neuropathologic studies (see Appendix 2 in the online supplement). Neuropathologic diagnoses of Alzheimer’s disease were made according to the CERAD (Consortium to Establish a Registry for Alzheimer’s Disease) criteria (18), although all subjects with Alzheimer’s disease also met the National Institute on Aging–Reagan Institute criteria (19) for intermediate to high probability that their dementia was due to Alzheimer’s lesions. For unaffected control subjects, an experienced neuropathologist reviewed sections from the frontal pole, hippocampus, entorhinal cortex, and cerebellum stained with hematoxylin and eosin, Bielschowsky silver stain, and amyloid beta immunohistochemistry to confirm that there was no evidence of neurodegenerative disease (see Appendix 2 in the online supplement).
Quantitative Immunohistochemistry
Formalin-fixed, paraffin-embedded tissue sections 5 μm thick were immunostained on an automated stainer (Discovery Ultra, Ventana Medical Systems, Tucson, Ariz.) using the following primary antibodies (see Appendix 3 in the online supplement): PHF-1, oligomeric tau T22, beta-amyloid NAB228, and microglial markers Iba1 and HLA-DR. All slides were developed using a multimeric HRP/DAB detection system (Ventana Medical Systems). No counterstaining was performed to ease signal quantification.
Microscopy
Whole slide digital images of the immunostained sections were created using a Mirax MIDI slide scanner (Zeiss, Jena, Germany) at 40× resolution (0.116 micron/pixel). Digital image analysis was performed using the NearCyte software program (http://nearcyte.org) (Figure 1; see also Appendix 4 in the online supplement). All analyses were done blind to psychosis status.
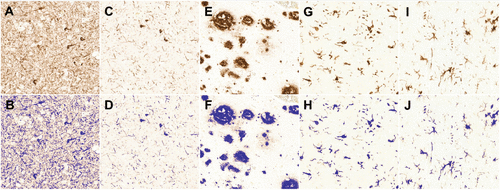
FIGURE 1. Representative Raw (Upper Row) and Masked (Lower Row) Images of Dorsolateral Prefrontal Cortex Sections Labeled With Selected Antibodies From Subjects With Alzheimer’s Diseasea
a PHF-1 tau (panels A, B); oligomeric (T22) tau (panels C, D); fibrillar (NAB228) amyloid beta (panels E, F); Iba1 (panels G, H), and HLA-DR (panels I, J). All images were captured at 40× magnification.
Animals and Tissue Preparation for LC-MS/MS
APPswe/PSEN1dE9 males were mated with Kalrn+/− females at University of Pittsburgh to obtain four genotypes as previously described (20) (see Appendix 5 in the online supplement): APPswe/PSEN1dE9/Kalrn+/+ (APP/PS1/KALRN[+/+]), APPswe/PSEN1dE9/Kalrn+/− (APP/PS1/KALRN[+/−]), Kalrn+/+ (wild type), and Kalrn+/−.
Samples from the right cerebral cortex of 5- to 6-month-old mice were homogenized, the postsynaptic density enrichment was obtained (see Appendix 6 in the online supplement), and samples were analyzed by LC-MS/MS.
LC-MS/MS
Tissue from 56 of the subjects with Alzheimer’s disease and 12 control subjects (Table 1) had previously been prepared and examined via LC-MS/MS using a TSQ Quantiva triple-stage quadrupole mass spectrometer (Thermo Scientific, Waltham, Mass.) with a Dionex UltiMate 3000 Nano HPLC (Thermo Scientific) (21). Tissue homogenates were prepared from fresh frozen dorsolateral prefrontal cortex gray matter and total protein was extracted, quantified by BCA, mixed with a lysine-13C6–labeled neuronal proteome standard, and subjected to in-gel trypsin digestion, as previously described (21). Samples were organized in a balanced block distribution throughout processing. Each block included subjects with Alzheimer’s disease with and without psychosis, a control subject, and a pooled technical replicate.
The protein panel used in this study, which has been comprehensively described (22), targets synaptically localized proteins, the majority of which have been demonstrated to be stable for 24 hours postmortem in an animal model. Specifically, the assay detects proteins involved in various aspects of synaptic activity, including vesicular fusion, energy metabolism, protein trafficking, and cytoskeleton scaffolding, as well as glutamate receptors, kinases, and phosphatases.
For human samples, 311 peptides from 190 proteins were assessed. For mouse samples, 274 peptides from 174 proteins were assessed in protein homogenate, and 203 peptides from 138 proteins were assessed from the postsynaptic density fraction. Precision of the assay was high, with a mean peptide quantification coefficient of variation of 8.6% for human samples, and 12.5% in homogenate and 17.7% in postsynaptic density fraction for mouse samples.
Statistical Analysis
A univariate test was performed to compare Alzheimer’s disease with and without psychosis for each demographic, clinical, and neuropathologic measure. Since comorbid pathologies are often correlated, we then conducted a stepwise logistic regression using the presence of psychosis as a binary outcome variable and the demographic and neuropathologic measures as covariates. Variables retained in the final logistic model were considered as covariates for the synaptic proteomics data analysis.
Protein levels were derived by calculating the weighted average of all standardized (centered and scaled) peptide measures mapped to a protein, with weights inverse to the coefficient of variation of the peptide measures (21). Analysis of covariance (adjusted for assay block, postmortem interval, and, when comparing Alzheimer’s disease with and without psychosis directly, variables retained in the stepwise logistic regression) was performed for each protein level to compare Alzheimer’s disease with and without psychosis. The covariate-adjusted protein ratios of Alzheimer’s disease without psychosis compared with Alzheimer’s disease with psychosis were obtained (on log2 scale), and a one-sample t test was performed to detect a significant shift in the distribution of ratios. In addition, protein levels of each Alzheimer’s group were calculated as a ratio to the control group (adjusted for assay block and postmortem interval) and tested for distributional shifts using one-sample t tests. Similar analyses were performed on proteomics data from mouse samples (see Appendix 7 in the online supplement).
Results
Tau and Other Neuropathologic Measures in Alzheimer’s Disease With and Without Psychosis
We first evaluated the association of psychosis in Alzheimer’s disease with measures of neuropathology (Table 2). Compared with Alzheimer’s disease without psychosis, Alzheimer’s disease with psychosis was characterized by significantly greater PHF-1 tau volume fraction (the fraction of gray matter containing PHF-1 tau labeling) and mean intensity. In contrast to PHF-1 tau, we detected only modest, nonsignificant elevations in oligomeric tau volume fraction. Alzheimer’s disease with psychosis did not differ from Alzheimer’s disease without psychosis on measures of amyloid beta volume fraction or intensity or on measures of microglial density (Iba1) or reactivity (HLA-DR). While we found no association of Lewy body pathology with Alzheimer’s disease with psychosis, we found a significant increase in TDP-43 pathology and less severe vascular pathology in subjects with psychotic symptoms in Alzheimer’s disease compared with those without psychosis. Sample distributions for all quantitative immunohistochemistry results are presented in Figure 2, and relationships of the measures to synaptic protein levels are listed in Table S6 in the online supplement.
Alzheimer’s Disease Without Psychosis (N=59) | Alzheimer’s Disease With Psychosis (N=81) | ||||
---|---|---|---|---|---|
Variable | Mean or Total | Range, SD (±), or % of Group Total | Mean or Total | Range, SD (±), or % of Group Total | p |
PHF-1 tau | |||||
Volume fraction | 0.009 | 0.0001, 0.3347 | 0.025 | 0.0002, 0.3294 | 0.01 |
log2(Volume fraction) | –6.86 | ±3.76 | –5.30 | ±2.84 | |
log2(Intensity) | 7.28 | ±0.17 | 7.34 | ±0.11 | 0.03 |
Oligomeric tau | |||||
Volume fraction | 0.002 | 0.0001, 0.0418 | 0.003 | 0.0002, 0.0402 | 0.10 |
log2(Volume fraction) | –9.22 | ±2.37 | –8.57 | ±2.08 | |
log2(Intensity) | 7.35 | ±0.05 | 7.36 | ±0.04 | 0.52 |
Amyloid beta | |||||
Volume fraction | 0.037 | 0.0008, 0.1098 | 0.037 | 0.0018, 0.1298 | 0.99 |
log2(Volume fraction) | –4.76 | ±1.34 | –4.76 | ±1.15 | |
log2(Intensity) | 7.25 | ±0.17 | 7.25 | ±0.12 | 0.87 |
HLA-DR | |||||
Volume fraction | 0.006 | 0.0002, 0.0542 | 0.005 | 0.0001, 0.0552 | 0.46 |
log2(Volume fraction) | –7.40 | ±1.79 | –7.64 | ±2.12 | |
log2(Intensity) | 7.34 | ±0.04 | 7.34 | ±0.06 | 0.65 |
Iba1 | |||||
Volume fraction | 0.025 | 0.0034, 0.088 | 0.028 | 0.0044, 0.0774 | 0.32 |
log2(Volume fraction) | –5.31 | ±0.92 | –5.15 | ±0.92 | |
log2(Intensity) | 7.32 | ±0.05 | 7.31 | ±0.04 | 0.47 |
HLA-DR:Iba1 | |||||
Volume fraction ratio | 0.235 | 0.0072, 7.2941 | 0.177 | 0.0024, 2.2169 | 0.27 |
log2(Volume fraction) | –2.09 | ±2.06 | –2.50 | ±2.20 | |
log2(Intensity) | 0.02 | ±0.06 | 0.02 | ±0.06 | 0.87 |
Lewy body pathology | 0.20 | ||||
Positive | 25 | 43% | 43 | 56% | |
Negative | 33 | 57% | 34 | 44% | |
TDP-43 pathology | 0.04 | ||||
Positive | 25 | 45% | 50 | 65% | |
Negative | 30 | 55% | 27 | 35% | |
Microvascular lesion count | 0.07 | ||||
0 | 37 | 63% | 65 | 80% | |
>0 | 19 | 32% | 15 | 19% | |
Vascular sum score | 4.52 | ±1.60 | 3.99 | ±1.29 | 0.04 |
TABLE 2. Univariate Analyses of Neuropathologic Measures in Alzheimer’s Disease Without and With Psychosisa

FIGURE 2. Levels of Tau, Amyloid Beta, and Microglial Markers in Alzheimer’s Disease With and Without Psychosisa
a Subjects with Alzheimer’s disease with psychosis exhibit significantly elevated PHF-1 tau volume fraction (panel A) and mean intensity (panel B) relative to subjects with Alzheimer’s disease without psychosis. In contrast, subjects with Alzheimer’s disease with psychosis do not differ from those without psychosis on volume fraction of other neuropathologic hallmarks of Alzheimer’s disease, including oligomeric (T22) tau or fibrillar (NAB228) amyloid beta (panels C, E), nor on intensity levels for these measures (panels D, F). Volume fraction and intensity levels of HLA-DR and Iba1, measures of microglial reactivity and density, respectively, also do not differentiate Alzheimer’s disease with psychosis from Alzheimer’s disease without psychosis (panels G, H).
Because these comorbid pathologies are often correlated, we entered the demographic and neuropathologic measures into a stepwise logistic regression model using the presence of psychosis as the outcome variable. Approximately 18% of the variance in psychosis status could be explained by five neuropathologic variables that were retained in the model (Table 3). PHF-1 tau volume fraction was the single most significant predictor of psychosis in Alzheimer’s disease (7% of the variance in psychosis status, p=0.01). Significant independent contributions of TDP-43 pathology also persisted. In contrast, although it contributed to the overall model, vascular pathology was no longer significantly associated with psychosis in Alzheimer’s disease.
Variable | Odds Ratio | p | R2 |
---|---|---|---|
PHF-1 tau, log2(volume fraction) | 1.16 | 0.01 | 0.07 |
TDP-43 pathology, positive | 2.22 | 0.04 | 0.05 |
HLA-DR:Iba1, log2(volume fraction ratio) | 0.85 | 0.08 | 0.01 |
Microvascular lesion count, >0 | 0.56 | 0.21 | 0.04 |
Vascular sum score | 0.80 | 0.11 | 0.04 |
Total variance explained | 0.18 |
TABLE 3. Stepwise Logistic Regression Model of Neuropathologic Predictors of Psychosis in Alzheimer’s Diseasea
Synaptic Protein Levels in Alzheimer’s Disease With and Without Psychosis
Because only a minority of the variance in psychosis status was explained by clinical and pathologic measures, we sought to determine whether there were alterations in the synaptic proteome that would further explain the occurrence of psychosis in Alzheimer’s disease. Few differences in levels of individual synaptic proteins were present in those with psychotic symptoms in Alzheimer’s disease compared with Alzheimer’s disease without psychosis, and none of them were significant after consideration of multiple comparisons, with or without adjusting for covariates (see Table S2 in the online supplement). However, we noted that the majority of synaptic proteins had higher levels in subjects with Alzheimer’s disease without psychosis than in those with psychosis, a significant difference (Figure 3A; one-sample t test of log2 ratio: mean=0.21, SD=0.24, p≤0.001). Further analysis of the synaptic protein levels in each group relative to unaffected control subjects revealed a global increase in synaptic protein levels in Alzheimer’s disease without psychosis (Figure 3B; mean=0.09, SD=0.30, p≤0.001) and no difference in the distribution of synaptic protein levels in Alzheimer’s disease with psychosis (Figure 3C; mean=0.02, SD=0.32, p=0.36). When we used the covariate-adjusted levels of each protein to create a synaptic protein score for each subject, the same trends were evident, although they did not reach statistical significance (Alzheimer’s disease without psychosis, mean=0.065, SD=0.53; Alzheimer’s disease with psychosis, mean=−0.004, SD=0.74; control subjects, mean=−0.024, SD=0.25). Functional annotation analysis of the top 20 proteins that were increased in Alzheimer’s disease without psychosis compared with control subjects revealed enrichment for proteins involved in vesicular function (see Table S3 in the online supplement).

FIGURE 3. Distribution of 190 Synaptic Protein Levels in Subjects With Alzheimer’s Disease With and Without Psychosis and Unaffected Control Subjectsa
a Distributions of log2 ratios are shown for all 190 proteins, adjusted for covariates from Table 3, postmortem interval, and assay block (panel A) or, for comparison to unaffected control subjects, postmortem interval and assay block alone (panels B, C). The dashed vertical line represents no difference in the ratio of protein levels between groups. Alzheimer’s disease without psychosis is characterized by a significant rightward shift in the distribution of synaptic protein levels compared with Alzheimer’s disease with psychosis (panel A). Subjects with Alzheimer’s disease without psychosis also exhibit elevated synaptic protein levels compared with control subjects (panel B), whereas those with psychosis exhibit no difference from control subjects (panel C), suggesting a lack of synaptic protein compensation in the Alzheimer’s disease with psychosis group.
We then restricted our analysis to only those synaptic proteins whose levels are enriched in the postsynaptic density, a cellular compartment whose functional integrity directly contributes to maintenance of synaptic transmission (see Table S4 in the online supplement). We found that the compensatory increase in postsynaptic density proteins in Alzheimer’s disease without psychosis was significantly greater than for the other synaptic proteins (ratio in subjects with Alzheimer’s disease without psychosis to those with psychosis: postsynaptic density protein, mean=1.25, SD=0.22; other synaptic proteins, mean=1.15, SD=0.19; p=0.01).
Synaptic Protein Distributions in APPswe/PSEN1dE9 Mice With and Without Protection From Psychosis-Associated Behavior
We recently reported (20) that genetic reduction of Kalrn in APPswe/PSEN1dE9 mice (APP/PS1/KALRN[+/−]) confers resilience to psychosis-associated behaviors that otherwise increase between 6 and 12 months of age in APP/PS1/KALRN(+/+) mice. We examined whether similar evidence of resilience in the synaptic proteome was present in APP/PS1/KALRN(+/−) mice at 6 months, a time point that precedes their behavioral divergence from APP/PS1/KALRN(+/+) mice. Six-month-old APP/PS1/KALRN(+/−) mice had elevated synaptic protein levels from cerebral cortex homogenates compared with APP/PS1/KALRN(+/+) mice (Figure 4A; one-sample t test of log2 ratio: mean=0.09, SD=0.016, p≤0.001) and compared with wild-type mice (Figure 4C; mean=0.12, SD=0.13, p≤0.001). In contrast, APP/PS1/KALRN(+/+) mice had only a modest shift in synaptic protein levels compared with wild-type mice (Figure 4E; mean=0.03, SD=0.17, p=0.03). The synaptic proteins elevated in APP/PS1/KALRN(+/−) mice relative to APP/PS1/KALRN(+/+) mice significantly overlapped with the proteins elevated in Alzheimer’s disease without psychosis relative to Alzheimer’s disease with psychosis (χ2=33.83, df=1, p=6.0×10−9; see Table S7 in the online supplement).

FIGURE 4. Distribution of Synaptic Protein Levels in Cerebral Cortex Homogenates and Postsynaptic Density Fractions From APP/PS1/KALRN(+/+), APP/PS1/KALRN(+/−), and Wild-Type Micea
a Distributions of log2 ratios are shown for all 174 synaptic proteins assayed from cerebral cortex homogenates (panels A, C, E) and for all 138 synaptic proteins assayed from postsynaptic density fractions (panels B, D, F) in APP/PS1/KALRN(+/+) (N=5), APP/PS1/KALRN(+/−) (N=5), and wild-type mice (N=4). The dashed vertical line represents no difference in the ratio of protein levels between groups. The summary panels plot all (panel G) and postsynaptic density–enriched (panel H) synaptic protein levels from whole cell homogenates and postsynaptic density fractions from APP/PS1/KALRN(+/+) and APP/PS1/KALRN(+/−) mice, expressed as percent change from wild-type mean. Error bars represent standard error of the mean.
We then asked whether synaptic protein compensation in cortical homogenates of APP/PS1/KALRN(+/−) mice conferred protection from loss of these proteins in the postsynaptic density. APP/PS1/KALRN(+/−) mice with increased synaptic protein levels in cortical homogenate were able to maintain synaptic proteins at wild-type levels in the postsynaptic density compartment (Figure 4D; mean=0.00, SD=0.24, p=0.82) and had elevated synaptic protein levels compared with APP/PS1/KALRN(+/+) mice (Figure 4B; mean=0.07, SD=0.32, p=0.01). In contrast, APP/PS1/KALRN(+/+) mice had overall deficient levels of these proteins in the postsynaptic density compared with wild-type mice (Figure 4F; mean=−0.07, SD=0.32, p=0.02). The effects of mouse genotype and cellular compartment on overall synaptic protein levels are summarized in Figure 4G (effect of genotype, F=20.83, df=1, 623, p<0.001; effect of compartment, F=34.77, df=1, 623, p<0.001; genotype by compartment, F=0.33, df=1, 623, p=0.57). When we restricted these analyses to postsynaptic density–specific proteins, such as glutamate receptors and postsynaptic density–95, which are normally enriched in the postsynaptic density fraction (defined here as a ratio of postsynaptic density to homogenate protein level greater than 1.5 in wild-type mice [see Table S4 in the online supplement]), the same pattern was present (Figure 4H) (effect of genotype, F=6.22, df=1, 151, p=0.01; effect of compartment, F=9.16, df=1, 151, p=0.003; genotype by compartment, F=0.11, df=1, 151, p=0.74). Finally, in an effort to ascertain whether other proteins in homogenate could contribute to the observed postsynaptic density preservation, we analyzed the top 20 non–postsynaptic density–enriched proteins that were increased in homogenates of APP/PS1/KALRN(+/−) mice. We found significant enrichment for proteins involved in endocytic/vesicular protein transport (see Table S5 in the online supplement), although the specific proteins contributing to this enrichment differ from those in Alzheimer’s disease without psychosis.
Discussion
In this study, we combined quantitative neuropathology and LC-MS/MS to identify significant predictors of the resilient Alzheimer’s disease without psychosis phenotype. We detected significant contributions from PHF-1 tau burden and from the presence of TDP-43 pathology to the prediction of psychosis status in Alzheimer’s disease. Independent of these neuropathologic contributions, we then demonstrated that subjects with Alzheimer’s disease without psychosis have increased levels of synaptic proteins, particularly those that are enriched in the postsynaptic density, relative to those with psychosis. Finally, we recapitulated our human postmortem finding of elevated synaptic protein levels in Alzheimer’s disease without psychosis in a mouse model of amyloid beta overexpression that is protected from psychosis-associated behaviors that would otherwise progress between 6 and 12 months of age. Within the homogenate fraction, APP/PS1/KALRN(+/−) mice had elevated levels of postsynaptic density–enriched proteins compared with wild-type and APP/PS1/KALRN(+/+) mice, an effect that was associated with normalization of postsynaptic density–enriched proteins in the postsynaptic density compartment.
Previous studies exploring the neuropathologic correlates of psychosis in Alzheimer’s disease yielded variable results and were often limited by low case numbers, focus on single pathologies, or retrospective database review designs. To our knowledge, this study is the most comprehensive evaluation of neuropathologic factors to date, based on a large, well-characterized cohort from a single center and using unbiased quantitative approaches to measure disease burden. We confirmed the association of psychosis in Alzheimer’s disease with higher phosphorylated tau burden, as previously reported by our group and others (3). While previous studies suggested that psychosis status was linked with cerebrovascular disease and microinfarcts (23, 24), we did not detect a significant association between any of our vascular disease markers and psychosis in a logistic regression model. Contrary to previous reports, we detected less severe cerebrovascular disease in subjects with Alzheimer’s disease with psychosis in univariate analyses. The significance of this observation is unclear. We report, for the first time, a significant association between the presence of TDP-43 pathology and psychosis status. Concurrent TDP-43 is a frequent finding in brains of subjects with Alzheimer’s disease, seen in >50% of cases (25). While the presence of TDP-43 has been associated with worse cognition and memory impairment (25), no association with clinical subtypes has previously emerged (26). Our study provides the first link of TDP-43 pathology with a specific clinical phenotype.
The presence of a compensated synaptic proteome has not, to our knowledge, been previously associated with psychosis status in Alzheimer’s disease. Perhaps the most replicated clinical correlate of Alzheimer’s disease without psychosis is a slower rate of cognitive impairment, and therefore our findings of synaptic proteome compensation in Alzheimer’s disease without psychosis are congruent with previous studies suggesting that clinically evident onset of Alzheimer’s disease is delayed by a period of synaptic compensation, during which cognitive ability is relatively maintained despite significant plaque and neurofibrillary tangle pathology (27). Indeed, elevated synaptic markers during this period of mild cognitive impairment relative to both mild and severe Alzheimer’s disease have been described previously (28). Others have demonstrated in hippocampal tissue that the synaptic proteome has a biphasic expression pattern across pathologic stage (29), which is likely a compensatory effect early in disease. Further ultrastructural studies have suggested that there is morphologic evidence of compensation in the context of mild Alzheimer’s pathology, one of which demonstrated larger axospinous synapses within stratum radiatum and stratum lacunosum-moleculare, an effect that Nicholson and colleagues suggested represents increased synapse strength (30). Our findings extend these earlier observations, revealing that synaptic compensations may persist into more advanced disease stages in a subgroup of individuals defined by the resilient Alzheimer’s disease without psychosis phenotype.
An important question is what mechanism(s) might underlie the synaptic proteome compensation in Alzheimer’s disease without psychosis. A model in which resilience to psychosis in Alzheimer’s disease results from a shift in the proteostasis of forward and back-trafficking of synaptic proteins to and from the postsynaptic density for function and degradation (Figure 5) would be consistent with our data indicating that Kalrn reduction protects the synaptic proteome in APPswe/PSEN1dE9 mice. Kalirin protein has been shown to regulate actin transport motors and trafficking of endosomes (31). Indeed, we found evidence of accumulation of endocytic vesicle trafficking proteins in the homogenates of APP/PS1/KALRN(+/−) mice in conjunction with normalization of postsynaptic density protein levels. Furthermore, we recently identified a set of common single-nucleotide polymorphisms (SNPs) that are associated with resilience to psychosis in Alzheimer’s disease (32). Among the consistently protective SNPs was rs8082590 on chromosome 17, the protective allele of which is associated with reduced expression of TOM1L2 (33), the gene encoding Target Of Myb1 Like 2 Membrane Trafficking Protein. TOM1L2 protein is required for maturation of autophagosomes and subsequent fusion with lysosomes (34). Autophagy pathways that regulate the turnover of endosomal material from the synapse have strong evidence for involvement in Alzheimer’s disease (35). Therefore, reduced TOM1L2 expression conferred by a common SNP may be a novel mechanism for increased synaptic protein levels and resilience to psychosis in Alzheimer’s disease. Autophagy pathways have also been shown to modulate the phosphorylation state of tau (36, 37), providing a putative link to the findings in this and previous studies of reduced fibrillar tau in Alzheimer’s disease without psychosis (38) and others’ similar findings of reduced PHF-1 tau in synaptosomes from subjects protected from Alzheimer’s disease despite sufficient neuropathology for a postmortem Alzheimer’s disease diagnosis (39). Studies that examine the synaptic, morphologic, and behavioral effects of reduced TOM1L2 expression may provide further insight into mechanisms underlying a resilient Alzheimer’s disease phenotype.

FIGURE 5. Model of Resilience to Psychosis in Alzheimer’s Diseasea
a The normal process of maintaining postsynaptic function involves forward trafficking of synaptic proteins into spines where they can insert into the postsynaptic density. This process is counterbalanced by back-trafficking to the dendrite compartments. Alzheimer’s pathology and a mouse model of amyloid beta overproduction are associated with an imbalance in the processes contributing to maintaining protein levels at the synapse (shown in red). Resilience to psychosis is hypothesized to result from processes that increase dendritic spine levels of synaptic proteins, for example, reduced back-trafficking for degradation due to reduced TOM1L2.
We have interpreted our synaptic findings as representing resilience in the context of equivalent pathologic stage, based on several methodologic considerations that deserve discussion. We used an automated immunostaining method to provide uniform measures of multiple pathologies across subjects. Nevertheless, mean intensity values for these pathologies were skewed to the right, an effect that was particularly evident for detection of PHF-1 tau intensity. This limitation may have hindered our ability to identify even greater increases in PHF-1 tau burden in our Alzheimer’s disease with psychosis cohort, possibly affecting the degree of correction of our synaptic measures for this pathology. In addition, we note that we did not use this parametric approach to quantify the presence of comorbid Lewy body and TDP-43 pathology, as the burden of these pathologies within dorsolateral prefrontal cortex was too low and would have resulted in nearly all subjects being quantified at floor values.
Another potential concern regarding our synaptic findings relates to the control cohort used for LC-MS/MS. First, the control group was significantly younger than our groups of subjects with Alzheimer’s disease with and without psychosis. This discrepancy resulted from our effort to obtain a neuropathologically unaffected cohort, minimizing the possibility of Alzheimer’s disease–related pathologies influencing the synaptic proteome within subjects who appeared clinically unaffected. Several considerations, however, make this unlikely to be cause for concern. A recent proteomics study of hippocampal tissue from subjects 22–96 years of age demonstrated an effect of age on only 60 of 4,582 proteins assayed; only two of these 60 proteins, hippocalcin and vimentin, were contained in our synaptic assay (40). Moreover, we detected profound differences in global synaptic protein levels when only our precisely age-matched subjects with Alzheimer’s disease with and without psychosis were considered. A second concern is that postmortem intervals were longer in our control group. However, we previously demonstrated that the majority of the synaptic peptides assayed exhibit stable detection for periods longer than the postmortem intervals of our subjects (22), and therefore the differences we observed are unlikely to be an effect of postmortem interval.
In summary, we have demonstrated that increased levels of synaptic proteins are associated with protection from a more severe Alzheimer’s disease phenotype, Alzheimer’s disease with psychosis. This putative compensation exhibited by the synaptic proteome was independent of burden from multiple neuropathologies. While we have identified and described an analogous mouse model of Alzheimer’s disease without psychosis, both behaviorally and synaptically, further studies of animal models of resilience are necessary to detect protective mechanisms for psychosis in Alzheimer’s disease. One such future model is advised by our recent genetic findings suggesting an association between reduced TOM1L2 expression and protection from psychosis in Alzheimer’s disease. Neuropathologic, synaptic, and behavioral assessment of this model may inform prospective development of therapeutic targets to confer resilience to psychosis in Alzheimer’s disease.
1 : Psychotic symptoms in Alzheimer disease: evidence for a distinct phenotype. Mol Psychiatry 2003; 8:383–392Crossref, Medline, Google Scholar
2 : Genetics of psychosis of Alzheimer disease. Am J Med Genet B Neuropsychiatr Genet 2017; 174:27–35Crossref, Medline, Google Scholar
3 : Psychosis in Alzheimer’s disease. Biol Psychiatry 2014; 75:542–552Crossref, Medline, Google Scholar
4 : Incident psychosis in subjects with mild cognitive impairment or Alzheimer’s disease. J Clin Psychiatry 2016; 77:e1564–e1569Crossref, Medline, Google Scholar
5 : Epidemiology of and risk factors for psychosis of Alzheimer’s disease: a review of 55 studies published from 1990 to 2003. Am J Psychiatry 2005; 162:2022–2030Link, Google Scholar
6 : Psychiatric symptoms vary with the severity of dementia in probable Alzheimer’s disease. J Neuropsychiatry Clin Neurosci 2003; 15:346–353Crossref, Medline, Google Scholar
7 : Psychotic symptoms in Alzheimer’s disease are not associated with more severe neuropathologic features. Int Psychogeriatr 2000; 12:547–558Crossref, Medline, Google Scholar
8 : Microglial dysfunction in brain aging and Alzheimer’s disease. Biochem Pharmacol 2014; 88:594–604Crossref, Medline, Google Scholar
9 : Microglia actions in Alzheimer’s disease. Acta Neuropathol 2013; 126:461–477Crossref, Medline, Google Scholar
10 : Alzheimer’s disease is a synaptic failure. Science 2002; 298:789–791Crossref, Medline, Google Scholar
11 : Physical basis of cognitive alterations in Alzheimer’s disease: synapse loss is the major correlate of cognitive impairment. Ann Neurol 1991; 30:572–580Crossref, Medline, Google Scholar
12 : SPECT findings on psychosis in Alzheimer’s disease. Am J Psychiatry 1995; 152:1470–1475Link, Google Scholar
13 : Cerebral correlates of psychotic symptoms in Alzheimer’s disease. J Neurol Neurosurg Psychiatry 2000; 69:167–171Crossref, Medline, Google Scholar
14 : Psychosis of Alzheimer’s disease: gender differences in regional perfusion. Neurobiol Aging 2008; 29:1218–1225Crossref, Medline, Google Scholar
15 : Abnormal brain glucose metabolism in the delusional misidentification syndromes: a positron emission tomography study in Alzheimer disease. Biol Psychiatry 1995; 38:438–449Crossref, Medline, Google Scholar
16 : Delusional thoughts and regional frontal/temporal cortex metabolism in Alzheimer’s disease. Am J Psychiatry 2003; 160:341–349Link, Google Scholar
17 : Neuroanatomical correlates of neuropsychiatric symptoms in Alzheimer’s disease. Brain 2008; 131:2455–2463Crossref, Medline, Google Scholar
18 : The Consortium to Establish a Registry for Alzheimer’s Disease (CERAD), part II: standardization of the neuropathologic assessment of Alzheimer’s disease. Neurology 1991; 41:479–486Crossref, Medline, Google Scholar
19 : Consensus recommendations for the postmortem diagnosis of Alzheimer disease from the National Institute on Aging and the Reagan Institute Working Group on diagnostic criteria for the neuropathological assessment of Alzheimer disease. J Neuropathol Exp Neurol 1997; 56:1095–1097Crossref, Medline, Google Scholar
20 : Kalirin reduction rescues psychosis-associated behavioral deficits in APPswe/PSEN1dE9 transgenic mice. Neurobiol Aging 2017; 54:59–70Crossref, Medline, Google Scholar
21 : Apolipoprotein E*4 (APOE*4) genotype is associated with altered levels of glutamate signaling proteins and synaptic coexpression networks in the prefrontal cortex in mild to moderate Alzheimer disease. Mol Cell Proteomics 2016; 15:2252–2262Crossref, Medline, Google Scholar
22 : Biochemical fractionation and stable isotope dilution liquid chromatography–mass spectrometry for targeted and microdomain-specific protein quantification in human postmortem brain tissue. Mol Cell Proteomics 2012; 11:1670–1681Crossref, Medline, Google Scholar
23 : Lewy bodies, vascular risk factors, and subcortical arteriosclerotic leukoencephalopathy, but not Alzheimer pathology, are associated with development of psychosis in Alzheimer’s disease. J Alzheimers Dis 2016; 50:283–295Crossref, Medline, Google Scholar
24 : Clinicopathological correlation of psychosis and brain vascular changes in Alzheimer’s disease. Sci Rep 2016; 6:20858Crossref, Medline, Google Scholar
25 : TDP-43 is a key player in the clinical features associated with Alzheimer’s disease. Acta Neuropathol 2014; 127:811–824Crossref, Medline, Google Scholar
26 : TDP-43 in Alzheimer’s disease is not associated with clinical FTLD or Parkinsonism. J Neurol 2014; 261:1344–1348Crossref, Medline, Google Scholar
27 : Differential expression of synaptic proteins in the frontal and temporal cortex of elderly subjects with mild cognitive impairment. J Neuropathol Exp Neurol 2006; 65:592–601Crossref, Medline, Google Scholar
28 : Loss of precuneus dendritic spines immunopositive for spinophilin is related to cognitive impairment in early Alzheimer’s disease. Neurobiol Aging 2017; 55:159–166Crossref, Medline, Google Scholar
29 : Profiling the human hippocampal proteome at all pathologic stages of Alzheimer’s disease. Alzheimers Dement 2016; 12:654–668Crossref, Medline, Google Scholar
30 : Evidence for Alzheimer’s disease-linked synapse loss and compensation in mouse and human hippocampal CA1 pyramidal neurons. Brain Struct Funct 2015; 220:3143–3165Crossref, Medline, Google Scholar
31 : Kalirin12 interacts with dynamin. BMC Neurosci 2009; 10:61Crossref, Medline, Google Scholar
32 : Genetic risk for schizophrenia and psychosis in Alzheimer disease. Mol Psychiatry 2018; 23:963–972Crossref, Medline, Google Scholar
33 : Gene expression elucidates functional impact of polygenic risk for schizophrenia. Nat Neurosci 2016; 19:1442–1453Crossref, Medline, Google Scholar
34 : Autophagy receptors link myosin VI to autophagosomes to mediate Tom1-dependent autophagosome maturation and fusion with the lysosome. Nat Cell Biol 2012; 14:1024–1035Crossref, Medline, Google Scholar
35 : Endo-lysosomal and autophagic dysfunction: a driving factor in Alzheimer’s disease? J Neurochem 2017; 140:703–717Crossref, Medline, Google Scholar
36 : Autophagic-lysosomal perturbation enhances tau aggregation in transfectants with induced wild-type tau expression. Eur J Neurosci 2008; 27:1119–1130Crossref, Medline, Google Scholar
37 : Macroautophagy deficiency mediates age-dependent neurodegeneration through a phospho-tau pathway. Mol Neurodegener 2012; 7:48Crossref, Medline, Google Scholar
38 : Hyperphosphorylated tau is elevated in Alzheimer’s disease with psychosis. J Alzheimers Dis 2014; 39:759–773Crossref, Medline, Google Scholar
39 : Dissecting phenotypic traits linked to human resilience to Alzheimer’s pathology. Brain 2013; 136:2510–2526Crossref, Medline, Google Scholar
40 : Quantitative protein profiling of hippocampus during human aging. Neurobiol Aging 2016; 39:46–56Crossref, Medline, Google Scholar