Regional Deficits in Brain Volume in Schizophrenia: A Meta-Analysis of Voxel-Based Morphometry Studies
Abstract
OBJECTIVE: Voxel-based morphometry is a method for detecting group differences in the density or volume of brain matter. The authors reviewed the literature on use of voxel-based morphometry in schizophrenia imaging research to examine the capabilities of this method for clearly identifying specific structural differences in patients with schizophrenia, compared with healthy subjects. The authors looked for consistently reported results of relative deficits in gray and white matter in schizophrenia and evaluated voxel-based morphometry methods in order to propose a future strategy for using voxel-based morphometry in schizophrenia research. METHOD: The authors reviewed all voxel-based morphometry studies of schizophrenia that were published to May 2004 (15 studies). The studies included a total of 390 patients with a diagnosis of schizophrenia and 364 healthy volunteers. RESULTS: Gray and white matter deficits in patients with schizophrenia, relative to healthy comparison subjects, were reported in a total of 50 brain regions. Deficits were reported in two of the 50 regions in more than 50% of the studies and in nine of the 50 regions in one study only. The most consistent findings were of relative deficits in the left superior temporal gyrus and the left medial temporal lobe. Use of a smaller smoothing kernel (4–8 mm) led to detection of a greater number of regions implicated in schizophrenia. CONCLUSIONS: This review implicates the left superior temporal gyrus and the left medial temporal lobe as key regions of structural difference in patients with schizophrenia, compared to healthy subjects. The diversity of regions reported in voxel-based morphometry studies is in part related to the choice of variables in the automated process, such as smoothing kernel size and linear versus affine transformation, as well as to differences in patient groups. Voxel-based morphometry can be used as an exploratory whole-brain approach to identify abnormal brain regions in schizophrenia, which should then be validated by using region-of-interest analyses.
Given the early demonstration of smaller ventricle size in schizophrenia (1) and the known genetic predisposition to the disorder (2), researchers for the past 25 years have looked for more specific structural abnormalities in schizophrenia. Many brain regions have been implicated in schizophrenia by means of magnetic resonance imaging (MRI) studies; findings have included ventricular enlargement, total brain volume deficits, and deficits in brain volume within the frontal, temporal, and parietal regions (3–6). To date, no single region has been consistently reported to be abnormal.
Several meta-analyses and reviews of brain abnormalities in schizophrenia have been conducted (7–13). Their findings include larger ventricles, smaller mean cerebral volume, reversed asymmetry in the superior temporal gyrus, and smaller volume of the medial temporal lobes in patients with schizophrenia. In a thorough meta-analysis of studies of regional brain volumes in schizophrenia, Wright et al. (7) found that in 58 studies involving a total of 1,588 independent patients with schizophrenia, the mean cerebral volume of the patients was 2% smaller than the mean for the healthy comparison subjects. Another meta-analysis with more strict inclusion criteria showed that leftward asymmetry of the planum temporale (superior temporal gyrus) and the sylvian fissure was significantly less pronounced in schizophrenia patients than in healthy comparison subjects (9). Others have focused on more localized morphometric abnormalities. For instance, Zakzanis et al. (8) evaluated the strength and consistency of study findings published between 1980 and 2000 that reported temporal lobe abnormalities in patients with schizophrenia. They found heterogeneity in the findings, with some studies showing abnormal structure and function and others reporting no difference between patients and healthy comparison subjects. Other meta-analyses focused on medial temporal and diencephalic structures have reported a moderate effect size for findings of relative deficits in gray matter (10–13).
Studies reporting structural abnormalities in schizophrenia have included a variety of patient groups that differ in age at onset, medications received, length of treatment with medication, symptoms, presence of comorbidity, and severity of illness. Because of a possible progression of neuropathology during the illness, age and severity effects could cause variations in brain morphometry over time (14–16). In the following review, we therefore distinguish first-episode and chronic patient groups.
In the imaging literature, the most prominent method used to investigate structural abnormalities is region of interest analysis. Such analyses rely on a priori defined regions of interest and manual outlining or stereological procedures to obtain volumetric measurements. These user-dependent methods could contribute a bias to the results (17, 18). In addition, region of interest analysis tends to focus on specific hypothesized regions, and findings in unexpected or unspecified regions can be overlooked (19). Finally, manual region of interest techniques are laborious, which can hinder efficient processing of large cohorts.
Thus, an automated, efficient whole-brain analysis to detect structural differences would provide a means of identifying areas of cerebral abnormality in a user-independent way. One such method is voxel-based morphometry, which was first reported in a study that used statistical parametric mapping (20). Voxel-based morphometry involves a voxel-wise comparison of the probability of the presence of gray or white brain matter, sometimes described as the density or concentration of gray or white matter, between groups of subjects. It is an unbiased exploration of the whole brain, and it is therefore a useful initial approach to the analysis of structural images. Scans are spatially normalized into the same stereotactic space and segmented into gray matter, white matter, and CSF compartments. The images are smoothed by using a smoothing kernel of a selected size, which should be comparable to the size of the expected regional differences between the groups of brains (20). The relative amounts of each tissue class are compared on a voxel-wise basis between groups. Fifteen studies published to May 2004 applied this technique to MRI datasets of patients with schizophrenia. Several groups have adapted the concepts and steps of these methods, which are based on statistical parametric mapping, for voxel-based morphometry with other software (21–25). We reviewed the voxel-based morphometry studies in schizophrenia in terms of methodological differences and patient populations to identify the brain regions that are most consistently implicated in the disorder.
Method
Studies were considered for the review by using the following inclusion criteria: 1) they were published through May 2004 as an article (rather than a letter or an abstract), 2) they compared a group of subjects with schizophrenia (either first-episode patients or chronic patients) and a healthy comparison group, and 3) they utilized voxel-based morphometry analysis of MRI datasets to investigate differences in whole-brain matter by using an automated procedure, and they followed the general steps of normalization, segmentation, smoothing, and statistical analysis described in the early literature on the method (20, 26). Table 1 lists the articles included in the review and provides information about the subject groups, software used, voxel-based morphometry process (template used, affine registration, and varying methods), and smoothing kernel in each study (18, 21–25, 31–39). For each study, we identified the regions that were reported to be reduced in patients relative to comparison subjects, in order to establish which relative deficits are most consistently reported and whether specific methodological factors contribute to inconsistencies in results. We excluded gender effects and comparisons between additional groups, such as first-degree relatives or subjects with other psychiatric disorders. Therefore, the results included in our review represent the statistical comparison of brain matter volume in patients with that in healthy comparison subjects, regardless of any other comparisons each study might have reported. Hulshoff Pol et al. reported gray and white matter analyses for the same cohort in separate manuscripts (21, 39), and these results were combined for presentation in Figure 1. Voxel-based morphometry data are variously referred to as density, concentration, or volume. For ease of description, we will use the term “volume” to describe results, although we acknowledge that in most cases, these are not true structural volumes.
Regions with significant differences were identified according to the labeling reported by the authors in each study. Because studies used different smoothing kernels and defined the medial temporal regions in different ways, we did not distinguish subareas within the medial temporal region; in particular, the amygdala, entorhinal cortex, and hippocampus were all considered as part of the medial temporal lobe in this review.
Results
Fifty regions were reported to be reduced in patients with schizophrenia relative to healthy comparison subjects. Figure 2 shows the percentages of studies that found reductions in each of the 50 regions. Two brain regions were found to be reduced in patients, relative to comparison subjects, in more than 50% of the studies: the left medial temporal lobe (nine of 15 studies) and the left superior temporal gyrus (eight of 15 studies). In about 50% of the studies we reviewed, relative deficits in patients were reported in the following regions: left inferior frontal gyrus, left medial frontal gyrus, right superior temporal gyrus, and left parahippocampal gyrus. In contrast, significant relative deficits were reported in the following regions in only one of the 15 studies: left inferior parietal lobe, left and right middle temporal gyrus, left middle occipital gyrus, right fusiform gyrus, right and left posterior cingulate gyrus, right precentral gyrus, and left inferior temporal gyrus.
Figure 1 and Figure 2 summarize the findings of all 15 studies. Only three of the 15 studies in this review used a first-episode patient group; the findings of these studies are summarized in the last three columns in Figure 1. Of the regions that were reported to be significantly smaller in schizophrenia patients in at least six of the 15 studies, two regions showed a disparity between chronic and first-episode patients. Reduced left medial frontal gyrus volume in schizophrenia patients was reported in seven (64%) of 11 studies of chronic patients and in none of the three studies of first-episode patients. Reduced right anterior cingulate volume was reported in all three studies of first-episode patients that included analysis of this region and in only three (27%) of 11 studies of chronic patients.
In Figure 3 the 50 regions implicated were highlighted according to the Talairach demon (40) and displayed using mri3dX (http://www.aston.ac.uk/lhs/research/groups/nrg/mri3dx). The images are colored according to the percentage of studies that reported significant reductions in patients with schizophrenia (Figure 1 and Figure 2). The lateral views in Figure 3 show the relative deficits in the superior temporal and inferior frontal cortex, and the coronal and axial views show the relative deficits in the medial frontal and anterior cingulate cortex and in the medial temporal lobe.
We focused on the regions that were reported to be significantly reduced in patients with schizophrenia in more than 50% of the studies: the superior temporal gyrus (Figure 4, Table 2) and the medial temporal lobe (Figure 5, Table 3). In an analysis of the consistency of the results for each structure, the coordinate of the significant difference reported in each study was plotted as a sphere. The size of the smoothing kernel was used to determine the diameter of the sphere. For instance, if a study used an 8-mm smoothing kernel and reported a coordinate in the superior temporal gyrus, we created a sphere 8 mm in diameter and plotted it by using the reported coordinate. In Figure 4 and Figure 5, the color scale of the spheres is graded by the stringency of the statistics used. Dark red signifies papers with analyses by trend or cluster with no overlap between coordinates; lighter red signifies papers with analyses by voxel peak height. The overlap between the results for the different papers is additive, and areas with greater overlap are nearer to yellow. The coordinates of each reported significant deficit are given for the superior temporal gyrus in Table 2 and for the medial temporal lobe in Table 3.
The smoothing kernel is an important variable in the voxel-based morphometry method, and a range of kernel widths, from 4 mm to 12 mm, was used in these studies. Figure 6 shows the percentage of studies reporting reduced volume in schizophrenia patients in each brain region, according to whether a small (4–8 mm) or large (10–12 mm) smoothing kernel was used in the study. This analysis showed that smaller smoothing kernels were used in a greater number of studies that reported volume reductions in small structures such as the medial temporal lobes, parahippocampal gyrus, thalamus, superior temporal gyrus, medial frontal gyrus, and anterior cingulate.
Discussion
Summary of Results
We reviewed 15 studies that employed voxel-based morphometry in 14 different cohorts of schizophrenia patients and comparison subjects and found that of the 50 regions for which deficits in volume or density were reported, two regions were identified in more than 50% of studies: the left superior temporal gyrus (in 57% of studies) and the left medial temporal lobe (in 69% of studies). Deficits in nine regions were reported in only one of the 15 studies. Use of a smaller smoothing kernel (4–8 mm) tended to yield a greater number of areas with significant deficits, particularly in the smaller structures.
Methodological Considerations
Methodological differences in implementation of voxel-based morphometry may explain why some regions were reported to be reduced in schizophrenia patients in only one of the studies we reviewed. For example, nine studies used cluster-level significance in the statistical analyses, and this method has been reported to cause false positive results (20). To compare statistical procedures and determine an effect size, data on peak location, cluster size, and statistical threshold would be required for each region, and these data were not reported in every study we reviewed. In Figure 4 and Figure 5 we attempted to qualify these parameters for the superior temporal gyrus and medial temporal lobe in terms of the size (smoothing kernel), weight (statistical threshold), and location of the spheres. According to this qualitative representation, no systematic differences between regions identified with cluster-level and voxel-level statistics were detected.
In addition, the segmentation method in voxel-based morphometry requires a clear contrast between the different tissue types to avoid indistinguishability in regions where central gray matter structures have image intensities that most resemble those of white matter. These regions are particularly likely to show partial volume effects, which occur when a single voxel contains a mixture of tissue types instead of the assumed single tissue type. These effects can also arise in white matter regions near the ventricles, where voxels at the interface appear as gray matter. Patients with schizophrenia are particularly vulnerable to such partial volume effects because they have a higher ventricle-to-brain ratio than healthy volunteers (3, 41).
Improvements in voxel-based morphometry have been reported. Optimized voxel-based morphometry, described by Good et al. (26), includes use of a study-specific template and nonlinear normalization and takes into account Jacobian determinants. The optimized method involves the following steps: 1) segmenting the MR images in native space; 2) “cleaning” the segmented images by removing nonbrain voxels; 3) estimating normalization parameters to a normalized, averaged brain matter template; 4) reapplying these parameters to the whole-brain MR images; 5) segmenting the normalized image; and 6) cleaning the normalized brain matter image again (36). These steps are followed by the optional reintegration of the volume changes that occurred during nonlinear normalization by modulation with the Jacobian determinant of the deformation matrix, allowing for the evaluation of gray matter volume (in contrast to density) changes. Four studies in this review used this optimized method (22, 23, 31, 42).
The level of sensitivity of voxel-based morphometry, as well as its validation with region of interest analysis, has yet to be fully determined (18, 43). However, in a growing number of studies, voxel-based morphometry was validated by region of interest analysis (18, 44–48). Keller et al. (47) demonstrated methodological consistency between automated voxel-based morphometry and manual analysis of hippocampal volume in patients with temporal lobe epilepsy. In addition, Kubicki et al. (18) replicated a previous region of interest finding (in the same cohort) of smaller gray matter volume in the left posterior superior temporal gyrus in schizophrenia patients, relative to healthy comparison subjects and affective psychosis patients, as well as less density in the left medial temporal lobe in patients with schizophrenia (49). In some cases, voxel-based morphometry is less sensitive than region of interest methods (48), but sensitivity can be increased by using a small smoothing kernel and a small-volume correction, as well as a study-specific template (32).
Wilke et al. (50) sought to substantiate voxel-based morphometry by systematically determining the best combination of processing parameters for examination of malformations in cortical development, based on recommendations related to the optimized processing method (26). Wilke et al. used 99 permutations of normalization procedures and smoothing kernels, as well as density versus volume measurements, to compare 20 patients with known cortical malformation to 53 healthy age- and gender-matched comparison subjects. The results that most accurately matched the known cortical malformations were obtained with an affine-only approach and a 6-mm smoothing kernel. According to the matched filter theorem, the width of the smoothing kernel determines the scale at which morphological changes are most sensitively detected (51). The studies we reviewed used a wide range of smoothing kernels, as reported in the last column of Table 1. Figure 6 displays the study findings in terms of the size of the smoothing kernel used by each study. Among the most consistently reported abnormalities, medial temporal lobe abnormalities were more often reported in studies that used a smaller smoothing kernel. Superior temporal gyrus abnormalities were reported equally as often in studies that used large smoothing kernels and in those that used small smoothing kernels. Therefore, as the findings of Wilke et al. suggested, this variable should be chosen by using a hypothesis-driven approach for a specific region of interest, and it should be noted that use of a small smoothing kernel can possibly lead to false positive results in studies of schizophrenia patients.
Medial Temporal Lobe Findings
Left medial temporal lobe deficits in patients with schizophrenia, relative to comparison subjects, were found in nine of 13 studies, and right medial temporal lobe deficits in patients were reported in only three of 13 studies. (The remaining studies examined white matter only.) Our definition of the medial temporal lobe did not include the parahippocampal gyrus, which was reported to be relatively smaller in the left hemisphere in schizophrenia patients in seven of the 15 studies and relatively smaller in the right hemisphere in three of the 15 studies. This finding of a lateralized deficit in brain matter supports previous suggestions of less hemispheric dominance in brains of patients with schizophrenia (52).
Changes in brain density or volume in the limbic region in patients with schizophrenia have been reported for several years (7, 14, 19, 53–55). In a meta-analysis of 58 region of interest studies, in which a volume of 100% in the comparison group was assumed, patients with schizophrenia were found to have an overall volume of 94% in the left and right amygdala, 94% in the left hippocampus/amygdala, 95% in the right hippocampus/amygdala, and 93% in the left and 95% in the right parahippocampus (7). The majority of voxel-based morphometry studies reviewed here reported volume deficits in similar areas, including the left amygdala, left hippocampus, and left parahippocampal gyrus. Some postmortem studies agree with these imaging findings (55–58), although others found no volume changes in patients with schizophrenia (12, 59, 60). Several postmortem studies suggested that researchers who have reported volume deficits in the amygdala and hippocampus may actually have misinterpreted volume changes in the parahippocampal gyrus, because the boundaries of the hippocampus and amygdala are difficult to determine (61–63). In imaging research, the limbic regions could be susceptible to partial volume effects in the adjacent temporal or inferior horns of the lateral ventricles, as discussed previously. Nevertheless, the overall consistency of the MRI findings, including the findings of the automated voxel-based morphometry studies, implicates the limbic regions in schizophrenia. Future voxel-based morphometry research should use a small smoothing kernel, small-volume corrections, careful segmentation, and validation with region of interest analysis to further clarify these limbic region findings.
Superior Temporal Gyrus Findings
Reduced superior temporal gyrus volume in schizophrenia patients was the most frequent bilateral finding across the 15 studies; 57% of studies found this deficit in the left hemisphere, and 50% found it in the right hemisphere. This finding is supported by reports of reduced volumes of temporal lobe structures in recent MRI and postmortem investigations in the anterior (64–66), posterior (49, 67, 68), and total area of the superior temporal gyrus (69, 70). Other groups have not found morphological abnormalities in the superior temporal gyrus in schizophrenia (71, 72). In some studies, the deficit is reported to be lateralized to the left (64, 66, 68, 69, 73), but in other studies, the difference is bilateral (49, 65, 70, 71). In one study, the volume deficit in the planum temporale was bilateral, and there was loss of asymmetry of the surface area (67). In addition, two reviews (4, 6) reported superior temporal gyrus abnormalities in 67%–81% of studies (when the analysis was not restricted to gray matter), compared with the 50%–57% reported here, suggesting that the sensitivity of voxel-based morphometry may not be equivalent to that of region of interest studies.
The range of peak superior temporal gyrus coordinates reported by the studies included in this review is shown in Table 2. The locations of these coordinates include areas of the left arcuate fasciculus (24), Heschl’s gyrus (18), uncinate fasciculus (23, 36), Brodmann’s area 21 (37), and Brodmann’s area 22 (22, 23, 35). In the right hemisphere, the coordinates are located in the right arcuate fasciculus (24), primary auditory cortex (18), Brodmann’s area 38 (35, 37), Brodmann’s area 22 (36, 37), and Brodmann’s area 41 (31). The variety of volume deficits across the region should be considered in light of the known morphology of the superior temporal gyrus: not only is it subdivided both structurally and functionally, but it also has potentially varying and abundant connections with the parietal, prefrontal, and superior temporal regions. These connections have been defined by tracer projections in rhesus monkeys (73).
Morphometric abnormalities in this region have been linked to symptom type and severity. For instance, Barta et al. (65) found a significant correlation between the severity of auditory hallucinations and volume deficits in the left superior temporal gyrus. Kim et al. (74) found that abnormalities of the anterior and posterior regional matter in the superior temporal gyrus were associated with psychotic and negative symptoms, depending on the side of the brain in which the abnormalities occurred. In addition to comparing voxel-based morphometry results for patients and healthy subjects, Shapleske et al. (23) compared results for patients with and without a history of verbal auditory hallucinations. They found that patients with a history of hallucinations had volume deficits in the left insula and adjacent temporal lobe. These studies demonstrated that more specific morphological abnormalities can be illuminated in schizophrenia when patients are grouped according to specific prominent symptoms. Future voxel-based morphometry studies should take this approach to reduce patient heterogeneity and further delineate the functional deficits associated with morphological abnormalities in the superior temporal gyri.
Conclusions
We reviewed 15 studies that used voxel-based morphometry to identify structural abnormalities in the brains of patients with schizophrenia. Of the 50 regions investigated, the areas reported to be significantly reduced in schizophrenia patients in more than 50% of the studies were the left superior temporal gyrus and the left medial temporal lobe. Fifty percent of the studies reported volumetric decreases in the left parahippocampal gyrus, right superior temporal gyrus, left inferior frontal gyrus, and left medial frontal gyrus.
It is interesting to note that at least 31% of the studies did not find volume deficits in the left medial temporal lobe. Several explanations could account for this result. First, the methods varied considerably between studies. The most critical factor may be the size of the smoothing kernel. We found that the discriminatory power for detecting changes in small areas was lower with smoothing kernels of greater width. A second factor is heterogeneity of the disease process. Voxel-based morphometry produces clearer results in studies where the patient group has been carefully chosen to be homogeneous. For example, Krams et al. (75) studied patients with mirror movements due to Kallmann’s syndrome, Abell et al. (76) studied a group of subjects with Asperger’s syndrome who were selected on the basis of their performance on tests of theory of mind, and Watkins et al. (77) studied members of a four-generation family with an inherited speech disorder whose results on tests of speech had no overlap with the results for nonaffected members of the family. Thus, it is likely that more consistent abnormalities in schizophrenia would be reported if voxel-based morphometry were applied to clearly defined patient groups characterized by symptom profile, age, and disease duration.
![]() |
![]() |
![]() |
Received Sept. 12, 2004; revision received Dec. 14, 2004; accepted Feb. 9, 2005. From the Prince of Wales International Centre for SANE Research, Department of Psychiatry, and the Department of Experimental Psychology, University of Oxford. Address correspondence and reprint requests to Ms. Honea, Prince of Wales International Centre for SANE Research, Department of Psychiatry, University of Oxford, Warneford Hospital, Headington, Oxford, U.K. OX3 7JX; [email protected] (e-mail). Supported by SANE and the T.J. Crow Psychosis Trust. The authors thank Neil Roberts for comments on early presentations of this review, suggestions for graphic display, and comments on the final manuscript and Charles Cabral, Jr., for editorial assistance.
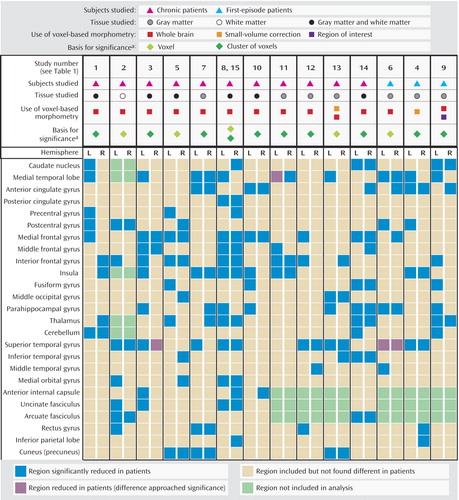
Figure 1. Overview of Findings of Reduced Volume of Gray or White Matter in Patients With Schizophrenia in Voxel-Based Morphometry Studies Published to May 2004
aThe following values were used to determine statistical significance: for studies 1 and 7, p<0.005; for study 2, t>5.29; for studies 3, 12, and 9, p<0.001; for studies 5, 13, 14, 6, and 4, p<0.05; for studies 8 and 15, t>5.00; and for studies 10 and 11, p<0.01.
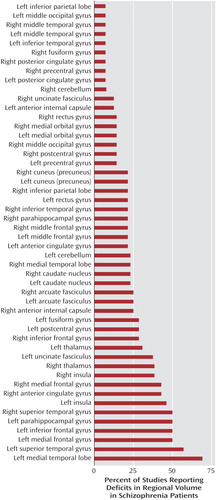
Figure 2. Percentage of Voxel-Based Morphometry Studies (N=15) With Significant Findings of Volume Deficits in Specific Brain Regions in Patients With Schizophreniaa
aThe total Ns for the percentages do not include studies in which the specific region was not investigated.
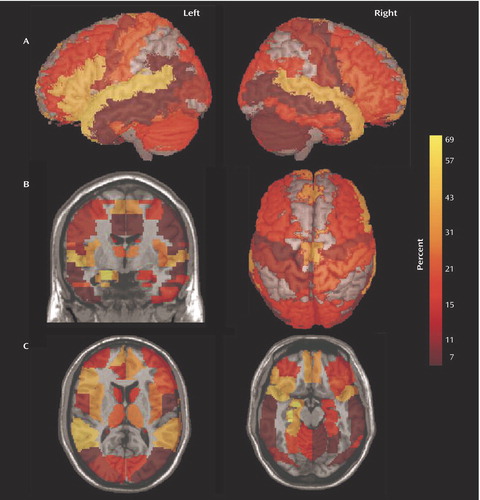
Figure 3. Brain Regions in Which Significant Volume Deficits in Patients With Schizophrenia Were Reported in Voxel-Based Morphometry Studies (N=15), by Percentage of Studies Reporting the Deficita
aIn row A, left and right whole-brain three-dimensional images are overlaid with all regions in which significant volume deficits in patients with schizophrenia were reported. In row B, a coronal view and an axial three-dimensional image are shown. In row C, axial views are shown. Figure 2 lists the regions with significant volume deficits and graphically presents the percentages of studies reporting the deficit.
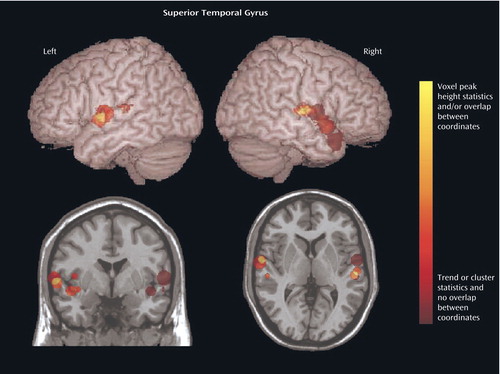
Figure 4. Location of Voxel-Based Morphometry Findings of Significant Volume Deficits in the Superior Temporal Gyrus in Patients With Schizophreniaa
aThe location (Talairach coordinates) of the significant difference reported in each study was plotted as a sphere. (The coordinates of each reported significant deficit are given in Table 2.) The size of the smoothing kernel used in the study was used to determine the diameter of the sphere. The top images are left and right three-dimensional images, respectively; the bottom left image is a coronal view, and the bottom right image is an axial view. The color scale depicts the stringency of the statistics used in the studies. Dark red signifies papers with analyses by trend or cluster with no overlap between coordinates; lighter red signifies papers with analyses by voxel peak height. The overlap between the results for the different papers is additive, and areas with greater overlap are nearer to yellow.
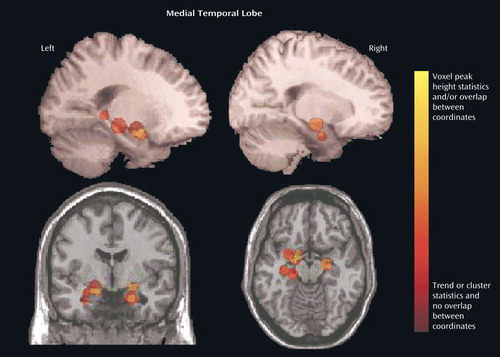
Figure 5. Location of Voxel-Based Morphometry Findings of Significant Volume Deficits in the Medial Temporal Lobe (Including the Amygdala and Hippocampus) in Patients With Schizophreniaa
aThe location (Talairach coordinates) of the significant difference reported in each study was plotted as a sphere. (The coordinates of each reported significant deficit are given in Table 3.) The size of the smoothing kernel used in the study was used to determine the diameter of the sphere. The top images are left and right three-dimensional images, respectively; the bottom left image is a coronal view, and the bottom right image is an axial view. The color scale depicts the stringency of the statistics used in the studies. Dark red signifies papers with analyses by trend or cluster with no overlap between coordinates; lighter red signifies papers with analyses by voxel peak height. The overlap between the results for the different papers is additive, and areas with greater overlap are nearer to yellow.
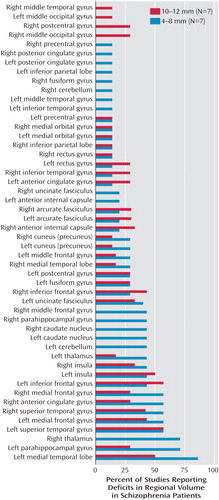
Figure 6. Percentage of Voxel-Based Morphometry Studies (N=15) With Significant Findings of Volume Deficits in Specific Brain Regions in Patients With Schizophrenia, by Size of the Smoothing Kernel Used in the Studya
aThe total Ns for the percentages do not include studies in which the specific region was not investigated. Two studies by Hulshoff Pol et al. (21, 39) (study 8 and study 15 in Table 1) were considered as one study in the calculation of percentages.
1. Johnstone EC, Crow TJ, Frith CD, Husband J, Kreel L: Cerebral ventricular size and cognitive impairment in chronic schizophrenia. Lancet 1976; 2:924–926Crossref, Medline, Google Scholar
2. Farmer AE, McGuffin P, Gottesman II: Twin concordance for DSM-III schizophrenia: scrutinizing the validity of the definition. Arch Gen Psychiatry 1987; 44:634–641Crossref, Medline, Google Scholar
3. Lawrie SM, Abukmeil SS: Brain abnormality in schizophrenia: a systematic and quantitative review of volumetric magnetic resonance imaging studies. Br J Psychiatry 1998; 172:110–120Crossref, Medline, Google Scholar
4. McCarley RW, Wible CG, Frumin M, Hirayasu Y, Levitt JJ, Fischer IA, Shenton ME: MRI anatomy of schizophrenia. Biol Psychiatry 1999; 45:1099–1119Crossref, Medline, Google Scholar
5. Ward KE, Friedman L, Wise A, Schulz SC: Meta-analysis of brain and cranial size in schizophrenia. Schizophr Res 1996; 22:197–213Crossref, Medline, Google Scholar
6. Shenton ME, Dickey CC, Frumin M, McCarley RW: A review of MRI findings in schizophrenia. Schizophr Res 2001; 49:1–52Crossref, Medline, Google Scholar
7. Wright IC, Rabe-Hesketh S, Woodruff PWR, David AS, Murray RM, Bullmore ET: Meta-analysis of regional brain volumes in schizophrenia. Am J Psychiatry 2000; 157:16–25Link, Google Scholar
8. Zakzanis KK, Poulin P, Hansen KT, Jolic D: Searching the schizophrenic brain for temporal lobe deficits: a systematic review and meta-analysis. Psychol Med 2000; 30:491–504Crossref, Medline, Google Scholar
9. Sommer I, Ramsey N, Kahn R, Aleman A, Bouma A: Handedness, language lateralisation and anatomical asymmetry in schizophrenia: meta-analysis. Br J Psychiatry 2001; 178:344–351Crossref, Medline, Google Scholar
10. Konick LC, Friedman L: Meta-analysis of thalamic size in schizophrenia. Biol Psychiatry 2001; 49:28–38Crossref, Medline, Google Scholar
11. Nelson MD, Saykin AJ, Flashman LA, Riordan HJ: Hippocampal volume reduction in schizophrenia as assessed by magnetic resonance imaging: a meta-analytic study. Arch Gen Psychiatry 1998; 55:433–440Crossref, Medline, Google Scholar
12. Chance SA, Esiri MM, Crow TJ: Amygdala volume in schizophrenia: post-mortem study and review of magnetic resonance imaging findings. Br J Psychiatry 2002; 180:331–338Crossref, Medline, Google Scholar
13. Shapleske J, Rossell SL, Woodruff PW, David AS: The planum temporale: a systematic, quantitative review of its structural, functional and clinical significance. Brain Res Brain Res Rev 1999; 29:26–49Crossref, Medline, Google Scholar
14. Gur RE, Turetsky BI, Bilker WB, Gur RC: Reduced gray matter volume in schizophrenia. Arch Gen Psychiatry 1999; 56:905–911Crossref, Medline, Google Scholar
15. Hulshoff Pol HE, Schnack HG, Bertens MGBC, van Haren NEM, van der Tweel I, Staal WG, Baaré WFC, Kahn RS: Volume changes in gray matter in patients with schizophrenia. Am J Psychiatry 2002; 159:244–250Link, Google Scholar
16. DeLisi LE, Sakuma M, Tew W, Kushner M, Hoff AL, Grimson R: Schizophrenia as a chronic active brain process: a study of progressive brain structural change subsequent to the onset of schizophrenia. Psychiatry Res 1997; 74:129–140Crossref, Medline, Google Scholar
17. Wolkin A, Rusinek H, Vaid G, Arena L, Lafargue T, Sanfilipo M, Loneragan C, Lautin A, Rotrosen J: Structural magnetic resonance image averaging in schizophrenia. Am J Psychiatry 1998; 155:1064–1073Link, Google Scholar
18. Kubicki M, Shenton ME, Salisbury DF, Hirayasu Y, Kasai K, Kikinis R, Jolesz FA, McCarley RW: Voxel-based morphometric analysis of gray matter in first episode schizophrenia. Neuroimage 2002; 17:1711–1719Crossref, Medline, Google Scholar
19. Goldstein JM, Goodman JM, Seidman LJ, Kennedy DN, Makris N, Lee H, Tourville J, Caviness VS Jr, Faraone SV, Tsuang MT: Cortical abnormalities in schizophrenia identified by structural magnetic resonance imaging. Arch Gen Psychiatry 1999; 56:537–547Crossref, Medline, Google Scholar
20. Ashburner J, Friston KJ: Voxel-based morphometry—the methods. Neuroimage 2000; 11(6, part 1):805–821Google Scholar
21. Hulshoff Pol HE, Schnack HG, Mandl RC, van Haren NE, Koning H, Collins DL, Evans AC, Kahn RS: Focal gray matter density changes in schizophrenia. Arch Gen Psychiatry 2001; 58:1118–1125Crossref, Medline, Google Scholar
22. Marcelis M, Suckling J, Woodruff P, Hofman P, Bullmore E, van Os J: Searching for a structural endophenotype in psychosis using computational morphometry. Psychiatry Res 2003; 122:153–167Crossref, Medline, Google Scholar
23. Shapleske J, Rossell SL, Chitnis XA, Suckling J, Simmons A, Bullmore ET, Woodruff PW, David AS: A computational morphometric MRI study of schizophrenia: effects of hallucinations. Cereb Cortex 2002; 12:1331–1341Crossref, Medline, Google Scholar
24. Sigmundsson T, Suckling J, Maier M, Williams SCR, Bullmore ET, Greenwood KE, Fukuda R, Ron MA, Toone BK: Structural abnormalities in frontal, temporal, and limbic regions and interconnecting white matter tracts in schizophrenic patients with prominent negative symptoms. Am J Psychiatry 2001; 158:234–243Link, Google Scholar
25. Spalletta G, Tomaiuolo F, Marino V, Bonaviri G, Trequattrini A, Caltagirone C: Chronic schizophrenia as a brain misconnection syndrome: a white matter voxel-based morphometry study. Schizophr Res 2003; 64:15–23Crossref, Medline, Google Scholar
26. Good CD, Johnsrude IS, Ashburner J, Henson RN, Friston KJ, Frackowiak RS: A voxel-based morphometric study of ageing in 465 normal adult human brains. Neuroimage 2001; 14(1, part 1):21–36Google Scholar
27. Suckling J, Sigmundsson T, Greenwood K, Bullmore ET: A modified fuzzy clustering algorithm for operator independent brain tissue classification of dual echo MR images. Magn Reson Imaging 1999; 17:1065–1076Crossref, Medline, Google Scholar
28. Press W, Teukolsky S, Vetterling W, Flannery B: Numerical Recipes in C: The Art of Scientific Computing, 2nd Ed. New York, Cambridge University Press, 1992Google Scholar
29. Brammer M, Bullmore E, Simmons A, Williams S, Grasby P, Howard R, Woodruff P, Rabe-Hesketh S: Generic brain activation mapping in functional magnetic resonance imaging: a nonparametric approach. Magn Reson Imaging 1997; 15:763–770Crossref, Medline, Google Scholar
30. Bullmore E, Brammer M, Rouleau G, Everitt B, Simmons A, Sharma T, Frangou S, Murray RM, Dunn G: Computerized brain tissue classification of magnetic resonance images: a new approach to the problem of partial volume artifact. Neuroimage 1995; 2:133–147Crossref, Medline, Google Scholar
31. Ananth H, Popescu I, Critchley HD, Good CD, Frackowiak RSJ, Dolan RJ: Cortical and subcortical gray matter abnormalities in schizophrenia determined through structural magnetic resonance imaging with optimized volumetric voxel-based morphometry. Am J Psychiatry 2002; 159:1497–1505Link, Google Scholar
32. Job DE, Whalley HC, McConnell S, Glabus M, Johnstone EC, Lawrie SM: Structural gray matter differences between first-episode schizophrenics and normal controls using voxel-based morphometry. Neuroimage 2002; 17:880–889Crossref, Medline, Google Scholar
33. Salgado-Pineda P, Baeza I, Perez-Gomez M, Vendrell P, Junque C, Bargallo N, Bernardo M: Sustained attention impairment correlates to gray matter decreases in first episode neuroleptic-naive schizophrenic patients. Neuroimage 2003; 19(2, part 1):365–375Google Scholar
34. Paillere-Martinot M, Caclin A, Artiges E, Poline JB, Joliot M, Mallet L, Recasens C, Attar-Levy D, Martinot JL: Cerebral gray and white matter reductions and clinical correlates in patients with early onset schizophrenia. Schizophr Res 2001; 50:19–26Crossref, Medline, Google Scholar
35. Wright IC, Ellison ZR, Sharma T, Friston KJ, Murray RM, McGuire PK: Mapping of grey matter changes in schizophrenia. Schizophr Res 1999; 35:1–14Crossref, Medline, Google Scholar
36. Wilke M, Kaufmann C, Grabner A, Putz B, Wetter TC, Auer DP: Gray matter-changes and correlates of disease severity in schizophrenia: a statistical parametric mapping study. Neuroimage 2001; 13:814–824Crossref, Medline, Google Scholar
37. Moorhead TW, Job DE, Whalley HC, Sanderson TL, Johnstone EC, Lawrie SM: Voxel-based morphometry of comorbid schizophrenia and learning disability: analyses in normalized and native spaces using parametric and nonparametric statistical methods. Neuroimage 2004; 22:188–202Crossref, Medline, Google Scholar
38. Salgado-Pineda P, Junque C, Vendrell P, Baeza I, Bargallo N, Falcon C, Bernardo M: Decreased cerebral activation during CPT performance: structural and functional deficits in schizophrenic patients. Neuroimage 2004; 21:840–847Crossref, Medline, Google Scholar
39. Hulshoff Pol HE, Schnack HG, Mandl RC, Cahn W, Collins DL, Evans AC, Kahn RS: Focal white matter density changes in schizophrenia: reduced inter-hemispheric connectivity. Neuroimage 2004; 21:27–35Crossref, Medline, Google Scholar
40. Lancaster JL, Woldorff MG, Parsons LM, Liotti M, Freitas CS, Rainey L, Kochunov PV, Nickerson D, Mikiten SA, Fox PT: Automated Talairach atlas labels for functional brain mapping. Hum Brain Mapp 2000; 10:120–131Crossref, Medline, Google Scholar
41. Van Horn JD, McManus IC: Ventricular enlargement in schizophrenia: a meta-analysis of studies of the ventricle:brain ratio (VBR). Br J Psychiatry 1992; 160:687–697Crossref, Medline, Google Scholar
42. Bagary MS, Symms MR, Barker GJ, Mutsatsa SH, Joyce EM, Ron MA: Gray and white matter brain abnormalities in first-episode schizophrenia inferred from magnetization transfer imaging. Arch Gen Psychiatry 2003; 60:779–788Crossref, Medline, Google Scholar
43. Tisserand DJ, Pruessner JC, Sanz Arigita EJ, van Boxtel MP, Evans AC, Jolles J, Uylings HB: Regional frontal cortical volumes decrease differentially in aging: an MRI study to compare volumetric approaches and voxel-based morphometry. Neuroimage 2002; 17:657–669Crossref, Medline, Google Scholar
44. Maguire EA, Gadian DG, Johnsrude IS, Good CD, Ashburner J, Frackowiak RS, Frith CD: Navigation-related structural change in the hippocampi of taxi drivers. Proc Natl Acad Sci USA 2000; 97:4398–4403Crossref, Medline, Google Scholar
45. Luders E, Gaser C, Jancke L, Schlaug G: A voxel-based approach to gray matter asymmetries. Neuroimage 2004; 22:656–664Crossref, Medline, Google Scholar
46. Sowell ER, Levitt J, Thompson PM, Holmes CJ, Blanton RE, Kornsand DS, Caplan R, McCracken J, Asarnow R, Toga AW: Brain abnormalities in early-onset schizophrenia spectrum disorder observed with statistical parametric mapping of structural magnetic resonance images. Am J Psychiatry 2000; 157:1475–1484Link, Google Scholar
47. Keller SS, Mackay CE, Barrick TR, Wieshmann UC, Howard MA, Roberts N: Voxel-based morphometric comparison of hippo-campal and extrahippocampal abnormalities in patients with left and right hippocampal atrophy. Neuroimage 2002; 16:23–31Crossref, Medline, Google Scholar
48. Good CD, Scahill RI, Fox NC, Ashburner J, Friston KJ, Chan D, Crum WR, Rossor MN, Frackowiak RS: Automatic differentiation of anatomical patterns in the human brain: validation with studies of degenerative dementias. Neuroimage 2002; 17:29–46Crossref, Medline, Google Scholar
49. Hirayasu Y, Shenton ME, Salisbury DF, Dickey CC, Fischer IA, Mazzoni P, Kisler T, Arakaki H, Kwon JS, Anderson JE, Yurgelun-Todd D, Tohen M, McCarley R: Lower left temporal lobe MRI volumes in patients with first-episode schizophrenia compared with psychotic patients with first-episode affective disorder and normal subjects. Am J Psychiatry 1998; 155:1384–1391Link, Google Scholar
50. Wilke M, Kassubek J, Schulze-Bonhage A, Huppertz HJ: Automated detection of gray matter malformations using optimized voxel-based morphometry: a systematic approach. Neuroimage 2003; 20:330–343Crossref, Medline, Google Scholar
51. White T, O’Leary D, Magnotta V, Arndt S, Flaum M, Andreasen NC: Anatomic and functional variability: the effects of filter size in group fMRI data analysis. Neuroimage 2001; 13:577–588Crossref, Medline, Google Scholar
52. Crow TJ: Schizophrenia as failure of hemispheric dominance for language. Trends Neurosci 1997; 20:339–343Crossref, Medline, Google Scholar
53. Schlaepfer TE, Harris GJ, Tien AY, Peng LW, Lee S, Federman EB, Chase GA, Barta PE, Pearlson GD: Decreased regional cortical gray matter volume in schizophrenia. Am J Psychiatry 1994; 151:842–848Link, Google Scholar
54. Heckers S, Konradi C: Hippocampal neurons in schizophrenia. J Neural Transm 2002; 109:891–905Crossref, Medline, Google Scholar
55. Heckers S, Heinsen H, Geiger B, Beckmann H: Hippocampal neuron number in schizophrenia: a stereological study. Arch Gen Psychiatry 1991; 48:1002–1008Crossref, Medline, Google Scholar
56. Jeste DV, Lohr JB: Hippocampal pathologic findings in schizophrenia: a morphometric study. Arch Gen Psychiatry 1989; 46:1019–1024Crossref, Medline, Google Scholar
57. Falkai P, Bogerts B, Rozumek M: Limbic pathology in schizophrenia: the entorhinal region—a morphometric study. Biol Psychiatry 1988; 24:515–521Crossref, Medline, Google Scholar
58. Bogerts B, Falkai P, Haupts M, Greve B, Ernst S, Tapernon-Franz U, Heinzmann U: Post-mortem volume measurements of limbic system and basal ganglia structures in chronic schizophrenics: initial results from a new brain collection. Schizophr Res 1990; 3:295–301Crossref, Medline, Google Scholar
59. Walker MA, Highley JR, Esiri MM, McDonald B, Roberts HC, Evans SP, Crow TJ: Estimated neuronal populations and volumes of the hippocampus and its subfields in schizophrenia. Am J Psychiatry 2002; 159:821–828Link, Google Scholar
60. Heckers S, Heinsen H, Heinsen YC, Beckmann H: Limbic structures and lateral ventricle in schizophrenia: a quantitative postmortem study. Arch Gen Psychiatry 1990; 47:1016–1022Crossref, Medline, Google Scholar
61. McDonald B, Highley JR, Walker MA, Herron BM, Cooper SJ, Esiri MM, Crow TJ: Anomalous asymmetry of fusiform and parahippocampal gyrus gray matter in schizophrenia: a postmortem study. Am J Psychiatry 2000; 157:40–47Link, Google Scholar
62. Brown R, Colter N, Corsellis JA, Crow TJ, Frith CD, Jagoe R, Johnstone EC, Marsh L: Postmortem evidence of structural brain changes in schizophrenia: differences in brain weight, temporal horn area, and parahippocampal gyrus compared with affective disorder. Arch Gen Psychiatry 1986; 43:36–42Crossref, Medline, Google Scholar
63. Altshuler LL, Bartzokis G, Grieder T, Curran J, Jimenez T, Leight K, Wilkins J, Gerner R, Mintz J: An MRI study of temporal lobe structures in men with bipolar disorder or schizophrenia. Biol Psychiatry 2000; 48:147–162Crossref, Medline, Google Scholar
64. Pearlson GD, Barta PE, Powers RE, Menon RR, Richards SS, Aylward EH, Federman EB, Chase GA, Petty RG, Tien AY: Ziskind-Somerfeld Research Award 1996: medial and superior temporal gyral volumes and cerebral asymmetry in schizophrenia versus bipolar disorder. Biol Psychiatry 1997; 41:1–14Crossref, Medline, Google Scholar
65. Barta PE, Pearlson GD, Powers RE, Richards SS, Tune LE: Auditory hallucinations and smaller superior temporal gyral volume in schizophrenia. Am J Psychiatry 1990; 147:1457–1462Link, Google Scholar
66. Barta PE, Pearlson GD, Brill LB II, Royall R, McGilchrist IK, Pulver AE, Powers RE, Casanova MF, Tien AY, Frangou S, Petty RG: Planum temporale asymmetry reversal in schizophrenia: replication and relationship to gray matter abnormalities. Am J Psychiatry 1997; 154:661–667Link, Google Scholar
67. Shenton ME, Kikinis R, Jolesz FA, Pollak SD, LeMay M, Wible CG, Hokama H, Martin J, Metcalf D, Coleman M, McCarley RW: Abnormalities of the left temporal lobe and thought disorder in schizophrenia: a quantitative magnetic resonance imaging study. N Engl J Med 1992; 327:604–612Crossref, Medline, Google Scholar
68. Sanfilipo M, Lafargue T, Rusinek H, Arena L, Loneragan C, Lautin A, Feiner D, Rotrosen J, Wolkin A: Volumetric measure of the frontal and temporal lobe regions in schizophrenia: relationship to negative symptoms. Arch Gen Psychiatry 2000; 57:471–480Crossref, Medline, Google Scholar
69. Matsumoto H, Simmons A, Williams S, Hadjulis M, Pipe R, Murray R, Frangou S: Superior temporal gyrus abnormalities in early-onset schizophrenia: similarities and differences with adult-onset schizophrenia. Am J Psychiatry 2001; 158:1299–1304Link, Google Scholar
70. Gur RE, Turetsky BI, Cowell PE, Finkelman C, Maany V, Grossman RI, Arnold SE, Bilker WB, Gur RC: Temporolimbic volume reductions in schizophrenia. Arch Gen Psychiatry 2000; 57:769–775Crossref, Medline, Google Scholar
71. Zipursky RB, Marsh L, Lim KO, DeMent S, Shear PK, Sullivan EV, Murphy GM, Csernansky JG, Pfefferbaum A: Volumetric MRI assessment of temporal lobe structures in schizophrenia. Biol Psychiatry 1994; 35:501–516Crossref, Medline, Google Scholar
72. Highley JR, McDonald B, Walker MA, Esiri MM, Crow TJ: Schizophrenia and temporal lobe asymmetry: a post-mortem stereological study of tissue volume. Br J Psychiatry 1999; 175:127–134Crossref, Medline, Google Scholar
73. Seltzer B, Pandya DN: Parietal, temporal, and occipital projections to cortex of the superior temporal sulcus in the rhesus monkey: a retrograde tracer study. J Comp Neurol 1994; 343:445–463Crossref, Medline, Google Scholar
74. Kim JJ, Crespo-Facorro B, Andreasen NC, O’Leary DS, Magnotta V, Nopoulos P: Morphology of the lateral superior temporal gyrus in neuroleptic naive patients with schizophrenia: relationship to symptoms. Schizophr Res 2003; 60:173–181Medline, Google Scholar
75. Krams M, Quinton R, Ashburner J, Friston KJ, Frackowiak RS, Bouloux PM, Passingham RE: Kallmann’s syndrome: mirror movements associated with bilateral corticospinal tract hypertrophy. Neurology 1999; 52:816–822Crossref, Medline, Google Scholar
76. Abell F, Krams M, Ashburner J, Passingham R, Friston K, Frac-kowiak R, Happe F, Frith C, Frith U: The neuroanatomy of autism: a voxel-based whole brain analysis of structural scans. Neuroreport 1999; 10:1647–1651Crossref, Medline, Google Scholar
77. Watkins KE, Vargha-Khadem F, Ashburner J, Passingham RE, Connelly A, Friston KJ, Frackowiak RS, Mishkin M, Gadian DG: MRI analysis of an inherited speech and language disorder: structural brain abnormalities. Brain 2002; 125(part 3):465–478Google Scholar