PET Study of [18F]6-Fluoro-l-Dopa Uptake in Neuroleptic- and Mood-Stabilizer-Naive First-Episode Nonpsychotic Mania: Effects of Treatment With Divalproex Sodium
Abstract
OBJECTIVE: Although dopaminergic hyperactivity has been implicated in mania, the precise location in the brain of the abnormality is unclear. This study assessed presynaptic dopamine function in neuroleptic- and mood-stabilizer-naive nonpsychotic first-episode manic patients before and after treatment with divalproex sodium by measuring [18F]6-fluoro-l-dopa ([18F]DOPA) uptake in the striatum with positron emission tomography (PET). METHOD: Thirteen patients with DSM-IV bipolar I disorder, manic episode, and 13 healthy comparison subjects underwent [18F]DOPA PET scans. Ten of the 13 patients had repeat PET scans 2–6 weeks after beginning treatment with divalproex sodium monotherapy. [18F]DOPA uptake rate constants (Ki values) in the striatum were calculated by using graphical analysis with activity from the occipital cortex as the input function. RESULTS: No significant differences in [18F]DOPA uptake rate constants in the striatum were found between the manic patients and the comparison subjects. After treatment with divalproex sodium, [18F]DOPA rate constants were significantly reduced in the patients and were lower in the patients than in the comparison subjects. CONCLUSIONS: Although presynaptic dopamine function as reflected by [18F]DOPA uptake is not altered in mania, presynaptic dopamine function in manic patients was lower after treatment with divalproex sodium.
The dopamine hypothesis of bipolar disorder implicates dopamine hyperactivity in mania. The most robust evidence for this hypothesis is derived mainly from observations of the behavioral effects of dopamine agonists and antagonists. For example, amphetamine, a psychostimulant, produces euphoria and other behavioral effects that are very similar to mania (1–3); these responses can be blocked by dopamine antagonists (4–7). There is evidence that dextroamphetamine-induced arousal and euphoria in humans is mediated by a dopaminergic mechanism, as it is blocked by the selective dopamine blocker pimozide (8). Administration of drugs that increase dopamine transmission, such as l-dopa, d-amphetamine, piribedil, and bromocriptine, has been reported to precipitate mania in patients with bipolar depression (9–14).
Alpha-methyl-para-tyrosine, which blocks tyrosine hydroxylase and decreases dopamine synthesis, is effective in reducing manic symptoms (15), whereas fusaric acid, which inhibits dopamine β-hydroxylase and raises dopamine levels, worsens manic symptoms (16). Conventional neuroleptics such as chlorpromazine, haloperidol, and trifluoperazine are all very effective in treating mania (17), and all conventional neuroleptics share the property of blocking dopamine receptors and thereby decreasing dopamine transmission. That the efficacy of neuroleptics is related to dopamine receptor blockade is suggested by the similar efficacy of pimozide, a more specific dopamine receptor antagonist, in reducing manic symptoms (18, 19). Further support for the dopamine hyperactivity theory of mania is provided by the observation that the cis isomer of clopenthixol, which possesses D2 receptor blocking properties, is effective in treating mania while the trans isomer, which does not block D2 receptors, has no antimanic properties (20).
Studies that have attempted to provide direct evidence for dopamine hyperactivity in mania have yielded inconsistent results. For example, the levels of homovanillic acid (HVA), a dopamine metabolite, have been reported to be higher (21–28) or no different (29, 30) in the CSF of manic patients than in the CSF of comparison subjects. Similarly, probenecid-induced accumulations of HVA have been reported to be no different (27–29, 31) or higher (32) in manic subjects than in comparison subjects. Studies of growth hormone responses to apomorphine challenge have also yielded inconsistent results, with some researchers reporting no difference (33) and others reporting higher (34) or lower levels (35) in manic patients, relative to comparison subjects.
Positron emission tomography (PET) can be used to examine various aspects of dopamine function in humans. This technique has been used widely to assess dopamine function in acute schizophrenia (36, 37). To our knowledge, just one study has used PET to measure dopamine function in acute mania (38), although two recent studies used single photon emission computed tomography (SPECT) (39) and PET (40) to assess aspects of presynaptic dopaminergic function in euthymic bipolar disorder patients. Pearlson and colleagues (38) examined dopamine D2 receptors using PET and [11C]N-methylspiperone in patients with bipolar disorder, compared with normal subjects and neuroleptic-naive schizophrenic patients. D2 receptor density was higher in seven patients with bipolar disorder who were psychotic and in 10 schizophrenic patients than in seven nonpsychotic patients with bipolar disorder and the normal comparison subjects. The authors concluded that the higher number of D2 receptors is a marker of psychosis and is not specific to schizophrenia or other disorders. However, it is noteworthy that this study included only five nonpsychotic manic patients (the other two were depressed), and, of these five, two had Young Mania Rating Scale scores of 9 and 5, suggesting minimal symptoms. Therefore, the question of whether or not higher dopamine D2 receptor density is associated with acute mania is unresolved.
In this study, we assessed presynaptic dopamine function in neuroleptic- and mood-stabilizer-naive nonpsychotic manic patients before and after treatment with divalproex sodium by measuring [18F]6-fluoro-l-dopa ([18F]DOPA) uptake in the striatum.
Method
Subjects
Patients who fulfilled DSM-IV criteria for bipolar I disorder, manic episode, without psychotic features (defined as absence of delusions or hallucinations) (N=13) were recruited from the University of British Columbia hospital in- and outpatient departments. The diagnosis of bipolar I disorder, manic episode, was reached on the basis of all the available medical information, which included a clinical interview by a board-certified psychiatrist (L.N.Y. or R.W.L.) and results of a Structured Clinical Interview for DSM-IV. Patients with comorbid substance/alcohol abuse or dependence or other axis I or II diagnoses were excluded. All patients who were recruited had never taken neuroleptics or mood stabilizers, and 11 of the 13 patients were in their first manic episode. The severity of manic symptoms was quantified with the Young Mania Rating Scale (41).
Thirteen healthy subjects similar in age to the manic patients were recruited through advertisements and screened by means of the nonpatient edition of the Structured Clinical Interview for DSM-IV. They had no lifetime history of psychiatric illness and no family history of mood disorders or schizophrenia in first-degree relatives. Both the patients and the comparison subjects were physically healthy and had not taken any psychotropic medication within at least 2 weeks before the baseline PET scan (manic patients were allowed to receive lorazepam as needed for agitation and sedation). Each study subject had a magnetic resonance imaging scan to exclude overt cerebral pathology and for co-registration with functional images to facilitate localization of brain regions on PET images. All subjects gave written informed consent for participation in the study, which was approved by the ethics committee of the University of British Columbia.
PET Procedure
[18F]DOPA was synthesized as previously described (42). Subjects fasted overnight and had a low-protein breakfast on the morning of the scans. PET scans were performed with an ECAT 953B/31 tomograph (CTI/Siemens, Knoxville, Tenn.). Data were acquired in three-dimensional mode and presented in 31 image planes with 3.375-mm plane separation. The transaxial image resolution was 8 mm, and the axial image resolution was 5 mm. Subjects were positioned supine in the gantry with the head centered in the field of view. A thermoplastic mask was molded to fit the subject’s head to minimize head movement, and the same mask was used for the repeat scan after treatment with divalproex sodium for patients. Each subject received 200 mg of carbidopa orally 1 hour before the injection of 185 MBq of [18F]DOPA. A transmission scan was obtained for attenuation correction before [18F]DOPA was injected. After injection of [18F]DOPA, 12 sequential emission scans (12 frames), each lasting 10 minutes, were obtained, for a total duration of 120 minutes.
Treatment With Divalproex Sodium
After the baseline [18F]DOPA scan, manic patients began treatment with divalproex monotherapy. Each subject was started on 500 mg of divalproex sodium b.i.d., and the dose was titrated to bring serum valproic acid levels to the therapeutic range (350–700 μmol/liter). Further dose adjustments were made as clinically indicated. Patients were not allowed to receive neuroleptics or any other psychotropic medication with the exception of lorazepam for agitation and oxazepam or temazepam for nighttime sedation. Three of the 13 patients had worsening of symptoms and required treatment with neuroleptics. The remaining 10 patients had a repeat [18F]DOPA PET scan between 2 and 6 weeks after beginning treatment with divalproex sodium. Repeat PET scans were performed within 5 days of remission of manic symptoms (remission was defined as a Young Mania Rating Scale score of 8 or less) or 6 weeks after commencement of divalproex sodium, whichever was earlier.
Image Analysis
Analysis was performed by using procedures for [18F]DOPA previously well established in our laboratory, as described in detail by our group elsewhere (43). Briefly, frames 7–12 were realigned to produce an integral image that consisted of radioactivity accumulated from 60 to 120 minutes after [18F]DOPA administration. Regions of interest were placed on the integral image on five contiguous planes where the striatum was best seen. One circular region of interest of 61.2 mm2 (diameter=8.8 mm) was placed on the caudate nucleus on each side and adjusted to maximize the average activity in the region of interest. Three circular regions of interest of the same size (61.2 mm2) were placed without overlap on the putamen on each side and adjusted to maximize activity in the region of interest. Three background regions of interest of 297 mm2 (diameter=19.4 mm) were positioned on each side of the temporo-occipital cortex. All of the regions of interest were replicated over 12 time frames. For each frame, regions of interest were averaged to get separate measurements for activity in the left and right caudate, the left and right putamen, and the background. A research assistant who was blind to the subject’s diagnosis and treatment status carried out these procedures.
The data were analyzed with a graphical method described elsewhere (44, 45), by using radioactivity time course of the temporo-occipital cortex as input function (46). This method generates values for the uptake rate constant (Kocc) for both caudate and putamen. Kocc (hereafter referred to as Ki) represents k2×k3/k2+k3, where k2 is the rate constant for backflow of fluorodopa from brain to plasma and k3 is the rate constant for describing the trapping of brain fluorodopa. The Ki values reflect the sum of blood-brain barrier transport and the decarboxylation of [18F]DOPA in dopaminergic terminals as well as the subsequent storage of [18F]dopamine into vesicles.
Statistical Analysis
The main aims of this study were to compare presynaptic dopamine function in the striatum in manic patients with that in comparison subjects and to determine if treatment with divalproex sodium altered presynaptic dopamine function. To achieve the first aim, we compared Ki values in the striatum between manic patients and healthy comparison subjects using an independent t test. To achieve the second aim, we compared Ki values in patients in the striatum before and after treatment using a paired t test. Further exploratory subanalyses included comparison of differences in the caudate, putamen, and right and left striatum between patients and comparison subjects and within patients before and after treatment by using independent and paired t tests. Pearson product-moment correlation was used to determine the relationship between Young Mania Rating Scale scores and Ki values before and after treatment.
Results
A total of 13 patients (seven women and six men) and 13 healthy comparison subjects (seven women and six men) participated in the study. Demographic and clinical characteristics of the patients are presented in Table 1. The mean age of the patients was 32.9 years (SD=12.0, range=18–52), and the mean age of the comparison subjects was 31.6 years (SD=11.5, range=20–51). There was no significant difference in age between the patient group and the comparison group (t=0.28, df=24, p=0.77). The patients’ mean Young Mania Rating Scale score was 26.5 (SD=7.2) before treatment and decreased to 5.0 (SD=6.23) after 2–6 weeks of divalproex sodium treatment.
Figure 1 shows scatterplots of Ki values for the striatum in the patients and the comparison subjects. The mean Ki value was 8.04 × 10–3 (SD=0.62 × 10–3) for the patients and 8.34 × 10–3 (SD=0.70 × 10–3) for the comparison subjects. The difference in Ki values between the two groups was not significant (t=1.13, df=24, p=0.27). Further exploratory analysis comparing Ki values in the caudate, putamen, and right and left striatum also showed no significant differences between patients and comparison subjects (data not shown). There was no significant correlation between patients’ Young Mania Rating Scale scores and pre- or posttreatment Ki values for the striatum.
The Ki values for the striatum before and after treatment with divalproex sodium for the 10 patients with complete data are shown in Figure 2. The mean Ki values decreased by 11.3% after treatment (pretreatment: Ki=8.03 × 10–3 [SD=0.62 × 10–3]; posttreatment: Ki=7.12 × 10–3 [SD=0.72 × 10–3]), a significant difference (t=4.38, df=9, p=0.002). Further exploratory analysis showed significant decreases with treatment in Ki values for the left striatum (t=4.44, df=9, p=0.002), right striatum (t=2.83, df=9, p=0.02), putamen (t=4.90, df=9, p=0.001), and caudate (t=2.32, df=9, p<0.05). The putamen had the largest decrease in Ki values (i.e., 13%). Figure 3 shows PET images displaying activity in the striatum before and after treatment with divalproex sodium.
Patients had significantly lower posttreatment Ki values (about 15% lower) than the healthy comparison subjects (t=–4.03, df=21, p=0.001), suggesting that the presynaptic dopamine function in patients after divalproex sodium treatment was lower than in the comparison subjects. There was no correlation between Young Mania Rating Scale change scores and changes in Ki values from baseline to posttreatment. There was also no correlation between the changes in [18F]DOPA uptake and divalproex sodium dose or duration of treatment.
Discussion
To our knowledge, this is the first study to examine [18F]DOPA uptake in neuroleptic- and mood-stabilizer-naive acutely ill manic patients. The lack of studies involving this group of patients is undoubtedly related to difficulty in recruiting medication-free manic patients for research. Recruitment of 13 medication-free patients for this study took nearly 5 years. The most important findings of this study are that the manic patients showed a significant decrease in [18F]DOPA uptake after treatment with divalproex sodium and that [18F]DOPA uptake in the patients after treatment with divalproex sodium was lower than in the healthy comparison subjects. However, there were no differences in baseline [18F]DOPA uptake between the manic patients and the healthy comparison subjects. There was also no correlation between [18F]DOPA uptake and severity of manic symptoms as measured by Young Mania Rating Scale scores.
The uptake rate constant for [18F]DOPA in the brain reflects the sum of the blood-brain barrier transport, the decarboxylation by the aromatic l-amino acid decarboxylase (AADC) to [18F]dopamine as well as vesicular storage of [18F]dopamine (47–49). Although it is unclear whether [18F]dopamine synthesis from exogenous [18F]DOPA reflects endogenous dopamine synthesis, the kinetic constants for 6-fluoro-l-dopa suggest that this is as good a substrate for AADC as l-dopa (50). Furthermore, [18F]dopamine mimics central dopamine metabolism remarkably well. Therefore, the [18F]DOPA rate constant should provide an index of AADC activity and transport and storage of [18F]dopamine into storage vesicles.
The lack of a significant difference in the [18F]DOPA rate constants between the manic patients and the comparison subjects in this study suggests that this component of presynaptic dopamine function was not increased in the manic patients. Because of the small size of the study group, the possibility of a type II error can not be excluded. Nonetheless, this study had 80% power to detect a 9% difference in [18F]DOPA rate constants between patients and comparison subjects, and it is unlikely that differences smaller that 9% are clinically meaningful. The mean Ki value for the patients was numerically lower than that for the comparison subjects (i.e., mean difference=–2.96 × 10–4), but the 95% confidence interval for the mean difference was –8.38 × 10–4 to 2.44 × 10–4. The [18F]DOPA rate constants reflect the sum of activity of AADC as well as transport and storage of [18F]dopamine into storage vesicles. Thus, our results suggest that these processes are intact in manic patients. However, our findings cannot exclude the possibility of enhanced dopamine release from presynaptic neurons, with consequent increase in dopaminergic transmission in mania. To our knowledge, no study has directly assessed the magnitude of dopamine release in acute mania. However, a recent SPECT study of change in D2 receptor availability after amphetamine challenge in euthymic bipolar disorder patients failed to demonstrate enhanced dopamine release (39). This study nevertheless revealed a higher level of behavioral responses (i.e., manic/hypomanic-like symptoms) to amphetamine challenge in the patients, compared to the healthy subjects. These results suggest enhanced postsynaptic dopamine receptor sensitivity and/or second messenger signaling pathway activity in euthymic bipolar disorder patients that may have led to induction of manic/hypomanic symptoms.
If enhancing dopamine transmission induces or worsens manic symptoms, it is plausible that effective antimanic treatments dampen dopamine hyperactivity by decreasing dopamine synthesis, release, or blockade of dopamine receptors or by dampening second messenger signaling pathways. Although the manic patients in this study did not have an abnormality in [18F]DOPA rate constants at baseline, they showed a significant reduction in [18F]DOPA rate constants after treatment with divalproex sodium. We did not have a comparison group that had the second scan after placebo or no treatment, and the normal volunteers in this study did not have a second scan. Therefore, one could argue that the reduction in Ki values in the patients could be due simply to scan-to-scan variation in these values and not to a treatment effect. However, the decrease in Ki values observed in the patients was greater than the scan-to-scan variation in Ki values found in normal healthy subjects in a previous study (43). Furthermore, the decrease in Ki values occurred in nine of the 10 patients. In addition, the decrease occurred in all areas of the striatum, but the largest decrease was noted in the putamen. Also, [18F]DOPA rate constants were lower in patients after divalproex sodium treatment than in the healthy comparison subjects. Taken together, these findings suggest that the reduction in [18F]DOPA rate constants was due to the effect of treatment with divalproex sodium or was a correlate of symptomatic change in the manic patients.
We did not find a correlation between changes in Young Mania Rating Scale scores and changes in Ki values between baseline and posttreatment scans in the patients. This result may suggest that the decrease in [18F]DOPA rate constants in the patients after divalproex sodium treatment may not be related to symptom change per se but instead may represent a nonspecific effect of divalproex sodium. On the other hand, the negative finding could be due to the fact that we did not separately measure [18F]DOPA uptake in the ventral striatum (due to the limited resolution of the PET camera). The separate measurement may have correlated better with symptom change (because mesolimbic dopamine transmission is more likely related to symptom change) than the sum of [18F]DOPA uptake in the dorsal and in the ventral striatum.
The results of this study cannot tell us whether the reduction in [18F]DOPA rate constants after treatment in the manic patients was due to a decrease in AADC activity or interference with the vesicular monoamine transporter 2 (VMAT2) site that mediates transport of dopamine from cytoplasm to storage vesicles. To our knowledge, no previous studies have directly assessed the effects of divalproex sodium on AADC activity or VMAT2 concentrations either in animals or in humans. However, previous evidence suggests that VMAT2 concentrations are not modulated by short- or long-term administration of drugs that affect monoamine function or metabolism (51). Furthermore, a recent SPECT study reported no alteration in VMAT2 concentrations, as measured by carbon 11-labeled dihydrotetrabenazine binding, in the caudate nuclei of euthymic bipolar disorder patients (40). Hence, the reduction in [18F]DOPA rate constants after treatment with divalproex sodium in the current study was more likely to be due to a decrease in AADC activity.
Decarboxylation of DOPA to dopamine by AADC is not rate limiting under the physiological conditions of dopamine synthesis. However, AADC is the catalyst for the first irreversibly committed step in the dopamine synthesis pathway and hence plays an important role in dopamine homeostasis (52). Indeed, studies have shown that AADC activity is enhanced by dopamine denervation or by dopamine D2 and D1 antagonists and is reduced by D2 agonists (53, 54). Similarly, AADC is regulated by endogenous dopamine levels, as depletion of dopamine by reserpine was shown to enhance AADC activity (53). Therefore, reduction in AADC activity with divalproex sodium treatment in this study would be expected to result in a reduction in dopamine synthesis, leading to a lower amount available for release and consequent reduction in dopamine hyperactivity.
The mechanism by which divalproex sodium reduces AADC activity is unknown. The divalproex sodium may alter the activity of AADC through pre- or posttranslational regulation. Administration of valproate for up to 32 days had no effect on mRNA levels of AADC in the rat (55). AADC protein contains a number of recognition motifs for phosphorylation by cAMP-dependent kinase, protein kinase C, calcium calmodulin-dependent protein kinase II, and proline-directed protein kinase (56), and it is possible that divalproex sodium may decrease the activity of AADC through inhibition of phosphorylation. However, this possibility needs to be tested directly in future studies.
Neuroleptics—such as the three standard mood stabilizers lithium, valproate, and carbamazepine—have proven antimanic properties. It is widely recognized that dopamine D2 receptor blockade contributes to the antimanic properties of neuroleptics, whereas the mechanism (or mechanisms) by which mood stabilizers exert an antimanic effect is currently unknown. Recent theories postulate that the effects of these drugs on second messenger signaling pathways are critical to the mood stabilizing properties, although to our knowledge, there is no direct evidence linking the two. Waldmeier (57), surveying the effects of mood stabilizers on various neurotransmitter pathways, concluded that the effects of these drugs are most similar with respect to reducing dopaminergic transmission, suggesting that this action may represent a common basis for the antimanic effect of these drugs. Reduction in AADC activity in manic patients after treatment with divalproex sodium in this study is consistent with such hypothesis.
In conclusion, the results of this study suggest no difference between acutely ill manic patients and healthy comparison subjects in the [18F]DOPA rate constant that provides an index of a component of presynaptic dopamine function. Treatment with divalproex sodium, however, led to a significant reduction in presynaptic dopamine function in manic patients, suggesting that reduction in AADC activity is associated with improvement in manic symptoms.
![]() |
Received July 19, 2001; revision received Oct. 15, 2001; accepted Oct. 18, 2001. Presented at the 4th International Conference on Bipolar Disorder, Pittsburgh, June 14–16, 2001. From the Division of Mood Disorders and the Division of Schizophrenia, Department of Psychiatry, and the Division of Neurology, Department of Medicine, University of British Columbia; TRIUMF PET Program, Vancouver, B.C.; and the Department of Psychiatry, Tri-Service General Hospital, National Defense Medical Center, Taipei, Taiwan.Address reprint requests to Dr. Yatham, Mood Disorders Clinical Research Unit, University of British Columbia, 2255 Wesbrook Mall, Vancouver, BC, Canada V6T 2A1; [email protected] (e-mail). Supported by the Canadian Institute of Health Research, the Stanley Foundation, and a Michael Smith Foundation Senior Scholar Award to Dr. Yatham.
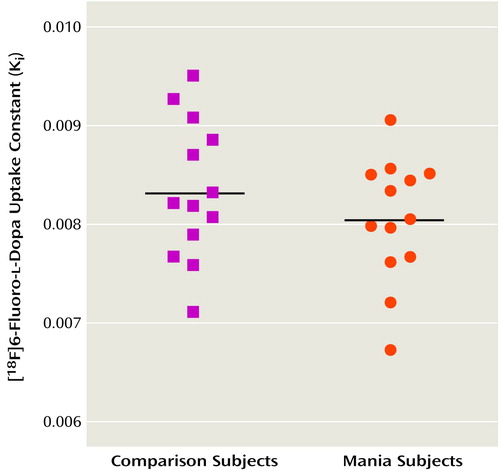
Figure 1. [18F]6-Fluoro-l-Dopa Uptake Rate Constants in Healthy Comparison Subjects (N=13) and in Patients With Acute Mania (N=13)
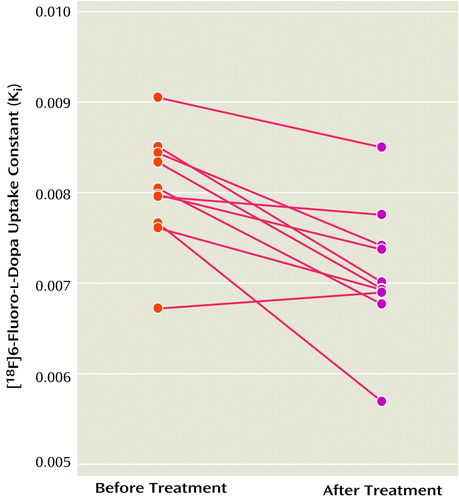
Figure 2. [18F]6-Fluoro-l-Dopa Uptake Rate Constants in Patients With Acute Mania (N=10) Before and After 2–6 Weeks of Treatment With Divalproex Sodium
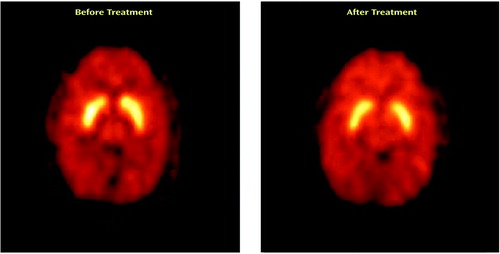
Figure 3. PET Transverse Image Showing [18F]6-Fluoro-l-Dopa Uptake in the Striatum of a Manic Patient Before and After Treatment With Divalproex Sodium
1. Silverstone T, Wells B, Trenchard E: Differential dose-response effects of dexamphetamine sulphate on hunger, arousal and mood in human volunteers. Psychopharmacology (Berl) 1983; 79:242-245Crossref, Medline, Google Scholar
2. Johanson CE, Uhlenhuth EH: Drug preference and mood in humans: d-amphetamine. Psychopharmacology (Berl) 1980; 71:275-279Crossref, Medline, Google Scholar
3. Halbreich U, Asnis G, Ross D, Endicott J: Amphetamine-induced dysphoria in postmenopausal women. Br J Psychiatry 1981; 138:470-473Crossref, Medline, Google Scholar
4. Anggard E, Jonsson LE, Hogmark AL, Gunne LM: Amphetamine metabolism in amphetamine psychosis. Clin Pharmacol Ther 1973; 14:870-880Medline, Google Scholar
5. Jonsson J, Gunne LM: Interaction of fenfluramine with d-amphetamine-induced excitatory behaviour and hyperthermia. Eur J Pharmacol 1972; 19:52-55Crossref, Medline, Google Scholar
6. Nurnberger JI, Gershon ES, Simmons S, Ebert M, Kessler LR, Dibble ED, Jimerson SS, Brown GM, Gold P, Jimerson DC, Guroff JJ, Storch FI: Behavioral, biochemical and neuroendocrine responses to amphetamine in normal twins and “well-state” bipolar patients. Psychoneuroendocrinology 1982; 7:163-176Crossref, Medline, Google Scholar
7. Wald D, Ebstein RP, Belmaker RH: Haloperidol and lithium blocking of the mood response to intravenous methylphenidate. Psychopharmacology (Berl) 1978; 57:83-87Crossref, Medline, Google Scholar
8. Silverstone T, Fincham J, Wells B, Kyriakides M: The effect of the dopamine receptor blocking drug pimozide on the stimulant and anorectic actions of dextroamphetamine in man. Neuropharmacology 1980; 19:1235-1237Crossref, Medline, Google Scholar
9. Murphy DL: l-Dopa, behavioral activation and psychopathology. Res Publ Assoc Res Nerv Ment Dis 1972; 50:472-493Medline, Google Scholar
10. van Kammen DP, Murphy DL: Attenuation of the euphoriant and activating effects of d- and l-amphetamine by lithium carbonate treatment. Psychopharmacologia 1975; 44:215-224Crossref, Medline, Google Scholar
11. Gerner RH, Post RM, Bunney WE Jr: A dopaminergic mechanism in mania. Am J Psychiatry 1976; 133:1177-1180Link, Google Scholar
12. Silverstone T: Response to bromocriptine distinguishes bipolar from unipolar depression. Lancet 1984; 1:903-904Crossref, Medline, Google Scholar
13. Brook NM, Cookson IB: Bromocriptine-induced mania? (letter). Br Med J 1978; 1:790Crossref, Medline, Google Scholar
14. Vlissides DN, Gill D, Castelow J: Bromocriptine-induced mania? (letter). Br Med J 1978; 1:510Crossref, Medline, Google Scholar
15. Brodie HK, Murphy DL, Goodwin FK, Bunney WE Jr: Catecholamines and mania: the effect of alpha-methyl-para-tyrosine on manic behavior and catecholamine metabolism. Clin Pharmacol Ther 1971; 12:218-224Medline, Google Scholar
16. Sack RL, Goodwin FK: Inhibition of dopamine-β-hydroxylase in manic patients: a clinical trial and fusaric acid. Arch Gen Psychiatry 1974; 31:649-654Crossref, Medline, Google Scholar
17. Chou JC: Recent advances in treatment of acute mania. J Clin Psychopharmacol 1991; 11:3-21Medline, Google Scholar
18. Post RM, Jimerson DC, Bunney WE Jr, Goodwin FK: Dopamine and mania: behavioral and biochemical effects of the dopamine receptor blocker pimozide. Psychopharmacology (Berl) 1980; 67:297-305Crossref, Medline, Google Scholar
19. Cookson J, Silverstone T, Wells B: Double-blind comparative clinical trial of pimozide and chlorpromazine in mania: a test of the dopamine hypothesis. Acta Psychiatr Scand 1981; 64:381-397Crossref, Medline, Google Scholar
20. Nolen WA: Dopamine and mania: the effects of trans- and cis-clopenthixol in a double-blind pilot study. J Affect Disord 1983; 5:91-96Crossref, Medline, Google Scholar
21. Redmond DE, Katz MM, Maas JW, Swann A, Casper R, Davis JM: Cerebrospinal fluid amine metabolites: relationships with behavioral measurements in depressed, manic, and healthy control subjects. Arch Gen Psychiatry 1986; 43:938-947Crossref, Medline, Google Scholar
22. Gerner RH, Fairbanks L, Anderson GM, Young JG, Scheinin M, Linnoila M, Hare TA, Shaywitz BA, Cohen DJ: CSF neurochemistry in depressed, manic, and schizophrenic patients compared with that of normal controls. Am J Psychiatry 1984; 141:1533-1540Link, Google Scholar
23. Swann AC, Secunda S, Davis JM, Robins E, Hanin I, Koslow SH, Maas JW: CSF monoamine metabolites in mania. Am J Psychiatry 1983; 140:396-400Link, Google Scholar
24. Koslow SH, Maas JW, Bowden CL, Davis JM, Hanin I, Javaid J: CSF and urinary biogenic amines and metabolites in depression and mania: a controlled, univariate analysis. Arch Gen Psychiatry 1983; 40:999-1010Crossref, Medline, Google Scholar
25. Vestergaard P, Sorensen T, Hoppe E, Rafaelsen OJ, Yates CM, Nicolaou N: Biogenic amine metabolites in cerebrospinal fluid of patients with affective disorders. Acta Psychiatr Scand 1978; 58:88-96Crossref, Medline, Google Scholar
26. Banki CM: Correlation between cerebrospinal fluid amine metabolites and psychomotor activity in affective disorders. J Neurochem 1977; 28:255-257Crossref, Medline, Google Scholar
27. Sjostrom R, Roos BE: 5-Hydroxyindolacetic acid and homovanillic acid in cerebrospinal fluid in manic-depressive psychosis. Eur J Clin Pharmacol 1972; 4:170-176Crossref, Medline, Google Scholar
28. Sjostrom R: 5-Hydroxyindole acetic acid and homovanillic acid in cerebrospinal fluid in manic-depressive psychosis and the effect of probenecid treatment. Eur J Clin Pharmacol 1973; 6:75-80Crossref, Medline, Google Scholar
29. Goodwin FK, Post RM, Dunner DL, Gordon EK: Cerebrospinal fluid amine metabolites in affective illness: the probenecid technique. Am J Psychiatry 1973; 130:73-79Link, Google Scholar
30. Wilk S, Shopsin B, Gershon S, Suhl M: Cerebrospinal fluid levels of MHPG in affective disorders. Nature 1972; 235:440-441Crossref, Medline, Google Scholar
31. Post RM, Fink E, Carpenter WT Jr, Goodwin FK: Cerebrospinal fluid amine metabolites in acute schizophrenia. Arch Gen Psychiatry 1975; 32:1063-1069Crossref, Medline, Google Scholar
32. Bowers MB: CSF acid monoamine metabolites as a possible reflection of central MAO activity in chronic schizophrenia. Biol Psychiatry 1976; 11:245-249Medline, Google Scholar
33. Casper RC, Davis JM, Pandey GN, Garver DL, Dekirmenjian H: Neuroendocrine and amine studies in affective illness. Psychoneuroendocrinology 1977; 2:105-113Crossref, Medline, Google Scholar
34. Ansseau M, von Frenckell R, Cerfontaine JL, Papart P, Franck G, Timsit-Berthier M, Geenen V, Legros JJ: Neuroendocrine evaluation of catecholaminergic neurotransmission in mania. Psychiatry Res 1987; 22:193-206Crossref, Medline, Google Scholar
35. Garver DL, Pandey GN, Hengeveld C, Davis JM: Growth hormone response and central aminergic systems in affective diseases (proceedings). Psychopharmacol Bull 1977; 13:61-63Medline, Google Scholar
36. Farde L, Wiesel FA, Stone-Elander S, Halldin C, Nordstrom AL, Hall H, Sedvall G: D2 dopamine receptors in neuroleptic-naive schizophrenic patients: a positron emission tomography study with [11C]raclopride. Arch Gen Psychiatry 1990; 47:213-219Crossref, Medline, Google Scholar
37. Wong DF, Wagner HN, Tune LE, Dannals RF, Pearlson GD, Links JM, Tamminga CA, Broussolle EP, Ravert HT, Wilson AA: Positron emission tomography reveals elevated D2 dopamine receptors in drug-naive schizophrenics. Science 1986; 234:1558-1563Crossref, Medline, Google Scholar
38. Pearlson GD, Wong DF, Tune LE, Ross CA, Chase GA, Links JM, Dannals RF, Wilson AA, Ravert HT, Wagner HN Jr: In vivo D2 dopamine receptor density in psychotic and nonpsychotic patients with bipolar disorder. Arch Gen Psychiatry 1995; 52:471-477Crossref, Medline, Google Scholar
39. Anand A, Verhoeff P, Seneca N, Zoghbi SS, Seibyl JP, Charney DS, Innis RB: Brain SPECT imaging of amphetamine-induced dopamine release in euthymic bipolar disorder patients. Am J Psychiatry 2000; 157:1108-1114Link, Google Scholar
40. Zubieta J-K, Huguelet P, Ohl LE, Koeppe RA, Kilbourn MR, Carr JM, Giordani BJ, Frey KA: High vesicular monoamine transporter binding in asymptomatic bipolar I disorder: sex differences and cognitive correlates. Am J Psychiatry 2000; 157:1619-1628Link, Google Scholar
41. Young RC, Biggs JT, Ziegler VE, Meyer DA: A rating scale for mania: reliability, validity and sensitivity. Br J Psychiatry 1978; 133:429-435Crossref, Medline, Google Scholar
42. Adam MJ, Ruth TJ, Grierson JR, Abeysekera B, Pate BD: Routine synthesis of l-[18F]6-fluorodopa with fluorine-18 acetyl hypofluorite. J Nucl Med 1986; 27:1462-1466Medline, Google Scholar
43. Vingerhoets FJ, Snow BJ, Schulzer M, Morrison S, Ruth TJ, Holden JE, Cooper S, Calne DB: Reproducibility of fluorine-18-6-fluorodopa positron emission tomography in normal human subjects. J Nucl Med 1994; 35:18-24Medline, Google Scholar
44. Patlak CS, Blasberg RG: Graphical evaluation of blood-to-brain transfer constants from multiple-time uptake data: generalizations. J Cereb Blood Flow Metab 1985; 5:584-590Crossref, Medline, Google Scholar
45. Martin WR, Palmer MR, Patlak CS, Calne DB: Nigrostriatal function in humans studied with positron emission tomography. Ann Neurol 1989; 26:535-542Crossref, Medline, Google Scholar
46. Brooks DJ, Salmon EP, Mathias CJ, Quinn N, Leenders KL, Bannister R, Marsden CD, Frackowiak RS: The relationship between locomotor disability, autonomic dysfunction, and the integrity of the striatal dopaminergic system in patients with multiple system atrophy, pure autonomic failure, and Parkinson’s disease, studied with PET. Brain 1990; 113(part 5):1539-1552Google Scholar
47. Garnett ES, Firnau G, Chan PK, Sood S, Belbeck LW: [18F]Fluoro-dopa, an analogue of dopa, and its use in direct external measurements of storage, degradation, and turnover of intracerebral dopamine. Proc Natl Acad Sci USA 1978; 75:464-467Crossref, Medline, Google Scholar
48. Deep P, Gjedde A, Cumming P: On the accuracy of an [18F]FDOPA compartmental model: evidence for vesicular storage of [18F]fluorodopamine in vivo. J Neurosci Methods 1997; 76:157-165Crossref, Medline, Google Scholar
49. Barrio JR, Huang SC, Phelps ME: Biological imaging and the molecular basis of dopaminergic diseases. Biochem Pharmacol 1997; 54:341-348Crossref, Medline, Google Scholar
50. Cumming P, Hausser M, Martin WR, Grierson J, Adam MJ, Ruth TJ, McGeer EG: Kinetics of in vitro decarboxylation and the in vivo metabolism of 2-18F- and 6-18F-fluorodopa in the hooded rat. Biochem Pharmacol 1988; 37:247-250Crossref, Medline, Google Scholar
51. Vander BT, Kilbourn M, Desmond T, Kuhl D, Frey K: The vesicular monoamine transporter is not regulated by dopaminergic drug treatments. Eur J Pharmacol 1995; 294:577-583Crossref, Medline, Google Scholar
52. Gjedde A, Leger GC, Cumming P, Yasuhara Y, Evans AC, Guttman M, Kuwabara H: Striatal l-dopa decarboxylase activity in Parkinson’s disease in vivo: implications for the regulation of dopamine synthesis. J Neurochem 1993; 61:1538-1541Crossref, Medline, Google Scholar
53. Hadjiconstantinou M, Wemlinger TA, Sylvia CP, Hubble JP, Neff NH: Aromatic l-amino acid decarboxylase activity of mouse striatum is modulated via dopamine receptors. J Neurochem 1993; 60:2175-2180Crossref, Medline, Google Scholar
54. Zhu MY, Juorio AV, Paterson IA, Boulton AA: Regulation of aromatic l-amino acid decarboxylase by dopamine receptors in the rat brain. J Neurochem 1992; 58:636-641Crossref, Medline, Google Scholar
55. Buckland PR, Spurlock G, McGuffin P: Amphetamine and vigabatrin down regulate aromatic l-amino acid decarboxylase mRNA levels. Brain Res Mol Brain Res 1996; 35:69-76Crossref, Medline, Google Scholar
56. Kemp BE, Pearson RB: Protein kinase recognition sequence motifs. Trends Biochem Sci 1990; 15:342-346Crossref, Medline, Google Scholar
57. Waldmeier PC: Is there a common denominator for the antimanic effect of lithium and anticonvulsants? Pharmacopsychiatry 1987; 20:37-47Crossref, Medline, Google Scholar