Children and Adolescents With Velocardiofacial Syndrome: A Volumetric MRI Study
Abstract
OBJECTIVE: Velocardiofacial syndrome is a common genetic condition often accompanied by mild cognitive impairment. Children and adolescents with velocardiofacial syndrome also are at greater risk for developing serious neuropsychiatric disorders in adulthood, particularly schizophrenia-like disorders. The purpose of this preliminary study was to 1) elucidate through brain imaging the neurobiological basis of cognitive and neuropsychiatric problems in velocardiofacial syndrome, and 2) consider the association between variations in neuroanatomy in velocardiofacial syndrome subjects and the associated neurobehavioral phenotype. METHOD: Fifteen children and adolescents with velocardiofacial syndrome were matched by age and gender with 15 comparison subjects. High-resolution magnetic resonance imaging scans were analyzed to provide quantitative measures of specified brain tissues and regions. Rater-blind morphometric analyses were conducted to examine tissue volumes of the four lobes and the cerebellum. RESULTS: Total brain volume was approximately 11% smaller in the children with velocardiofacial syndrome. Gray matter volume was reduced to a lesser extent (7.5%) than white matter volume (16.3%). Multivariate analyses of variance indicated a distinct pattern of regional morphological variation among the children with velocardiofacial syndrome. Specifically, frontal lobe tissue tended to be enlarged relative to the overall reduction in brain volume. Normal symmetry of parietal lobe tissue observed in the comparison group was not evident in the velocardiofacial syndrome group. This loss of symmetry was attributable to a significant reduction of gray matter in the left parietal lobe. CONCLUSIONS: Aberrant brain morphology is associated with velocardiofacial syndrome. These changes are potentially related to the language and learning deficits associated with the syndrome and may provide clues about neurodevelopmental pathways associated with schizophrenia.
Velocardiofacial syndrome is a congenital, autosomal dominant condition defined by Shprintzen et al. (1). Its prevalence is estimated at 1 per 4,000–4,500 live births (2). In most patients, a de novo deletion at chromosome 22q11.2 is responsible for the syndrome (3–6). The major features of velocardiofacial syndrome include cardiac malformations, cleft palate or velopharyngeal insufficiency, a characteristic facial appearance, and learning disabilities. More than 40 physical anomalies have been observed in association with velocardiofacial syndrome (7, 8).
Although several studies have delineated the physical phenotype associated with velocardiofacial syndrome, few have investigated the neurobehavioral and psychiatric phenotype. Golding-Kushner et al. (9) described linguistic as well as psychological and academic profiles of 26 patients with clinical (i.e., not confirmed by molecular analysis) velocardiofacial syndrome. In this retrospective study, children with velocardiofacial syndrome under 11 years of age had a mean IQ of 80, and the mean IQ of preadolescents and adolescents (between 11 and 18 years of age) with this syndrome was 70. Language and behavioral problems included a limited capacity for abstract reasoning, language delay, paucity of affects, monotonous voice, and difficulties in social interactions that ranged from extreme familiarity to social anxiety and avoidance. More recently, Swillen et al. (10) found that 45% of 37 children and adolescents with velocardiofacial syndrome had mental retardation (IQ<70), with verbal IQs that were significantly superior to performance IQs. These authors also emphasized problems with socialization (manifested principally as withdrawal) as well as attention and concentration problems.
The extant velocardiofacial syndrome literature contains few specific descriptions of speech and language aspects of the disorder. However, components of language functioning have been evaluated (9, 11). The most commonly reported difficulties associated with velocardiofacial syndrome include a nasal tone and problems with articulation (7); the origin of these expressive problems is assumed to be a cleft palate and pharyngeal hypotonia. Other descriptions of expressive speech have noted a shrill (12), weak (13), and monotonous voice, with the capacity to vary pitch on demand (9). Research has also reported developmental delay in language milestones (9) and specific deficits in language syntax, semantics, spelling, written comprehension, and arithmetic (7, 9).
Previous investigations have suggested that individuals with velocardiofacial syndrome are at risk for developing serious psychiatric illnesses. Shprintzen et al. (14) observed that at least 10%–20% of over 100 patients with velocardiofacial syndrome developed a psychiatric illness by the time they reached adulthood. In most cases, the diagnosis was chronic paranoid schizophrenia. In addition, Papolos et al. (15) and Carlson et al. (16) reported a high rate (70%) of manifestations within the bipolar disorder spectrum among 20 children and adolescents (nine of whom were younger than 13 years of age) that had reached 100% by the time the subjects reached adulthood. In summary, there is evidence of both cognitive and psychiatric dysfunction in persons with velocardiofacial syndrome.
Despite the observation of serious neurocognitive and psychiatric symptoms associated with this condition, little information exists concerning the neurobiology and brain development of subjects with velocardiofacial syndrome. To date there is only one published study (17) and a few case studies (18–20) on brain anomalies in subjects with velocardiofacial syndrome. In a qualitative analysis of magnetic resonance imaging (MRI) data, Mitnick et al. (17) concluded that nine of 11 study participants (mean age=9.5 years) had visible brain abnormalities. The most common finding was a small cerebellar vermis in five subjects. Additionally, reduced volume of the posterior fossa was found in four subjects, and cysts adjacent to the anterior horns of the ventricles were found in three.
The goal of the present study was to continue research into the neurobiology of velocardiofacial syndrome by contributing more precise quantitative information on brain development in children and adolescents with this condition. On the basis of results from previous qualitative velocardiofacial syndrome brain imaging studies (17–20) and the abundant literature on brain imaging in schizophrenia (21), we hypothesized volumetric changes in the frontal lobe, cerebellum, and ventricles. Additionally, the findings of temporal lobe abnormalities in schizophrenia (21, 22), combined with the common reporting of language problems in subjects with velocardiofacial syndrome (9, 23), pointed to the temporal lobe as a potential region of interest. The present study reports the first volumetric and morphometric segmentation study of the brain of subjects with velocardiofacial syndrome.
METHOD
Subjects
The study group consisted of 15 children and adolescents with velocardiofacial syndrome (five girls and 10 boys, mean age=10.5 years, SD=3.1) and 15 comparison subjects (mean age=10.8 years, SD=2.7). After providing a complete description of the study to the children with velocardiofacial syndrome and their parents, written informed consent was obtained under protocols approved by the institutional review board of Stanford University. Only subjects with proven velocardiofacial syndrome (determined by means of the fluorescent in situ hybridization technique to have a deletion at chromosome 22q11.2) were included in the study. Children presenting the velocardiofacial syndrome clinical phenotype without this deletion were excluded to increase diagnostic certainty. Recruitment was performed through the Northern California VCFS Support Network and by advertising on our web site. Imaging data for the normal comparison group were drawn from an archive of over 150 normal comparison subjects available in our laboratory. Normal comparison subjects were individually matched for age and gender and had a minimum IQ of 85 (one standard deviation below the mean).
MRI Protocol
Magnetic resonance images of each subject’s brain were acquired with a Signa 1.5-T scanner (GE Medical Systems, Milwaukee). Coronal images were acquired with a three-dimensional volumetric radio frequency spoiled gradient echo with the following scan parameters: TR=35 msec, TE=6 msec, flip angle=45°, number of excitations=1, image matrix=256 × 192 pixels, field of view=24 cm, slice thickness=1.5 mm, 124 slices.
Image Processing and Measurement
The spoiled gradient echo image data were imported into the program BrainImage for semiautomated image processing analysis and quantification as described and validated elsewhere (24–26). Data from this image processing pipeline are in the form of gray matter, white matter, and CSF volumes for the following structures: cerebral lobes, a subcortical region including the striatum and thalamus, the cerebellum, and the lateral ventricles.
To specify regional differences, the brain was divided into lobes with a semiautomated stereotactic-based parcellation method (25, 27, 28). Raters who conducted morphometric analyses were blinded to the group membership of subjects.
Statistical Analyses
Data were first examined for normality to conform to the assumptions of the parametric statistics employed. Multiple analysis of covariance (MANCOVA) was used to determine if the velocardiofacial syndrome subjects and the comparison group had unique patterns of tissue volume and composition. Analyses of total brain tissue, total gray matter, and total white matter were performed with one-way analyses of variance (ANOVAs), with diagnosis (velocardiofacial syndrome versus no condition) as a between-subject factor. Investigation of brain asymmetry used repeated measures ANOVA, which took diagnostic category as a between-subject factor and side (left versus right) as a within-subject factor. The interaction effect (group by side) was then used to determine group differences in asymmetry. Analysis of covariance (ANCOVA) was used for subregion comparisons to more accurately quantify group differences after statistically adjusting for the effect of total brain or tissue compartment volumes. A p value of 0.05 (two-tailed) was chosen as the significance threshold. The distributions of ventricular volume violated the homogeneity of variance assumption; therefore, nonparametric statistics were used to examine group differences for this variable. A ventricle-to-brain-volume ratio was computed to account for differences in total brain volume.
RESULTS
Group Differences in Tissue Volumes
As shown in table 1 and consistent with previous studies, the total brain tissue volume was approximately 11% smaller in the children with velocardiofacial syndrome than in the comparison subjects. Reduced tissue volume was comparable for the left and right hemispheres when analyzed separately.
To investigate the hypothesis that brain development in subjects with velocardiofacial syndrome differs from that observed in comparison subjects, a MANCOVA was computed with group as a main effect (velocardiofacial syndrome versus no condition) and total brain volume entered as a covariate in order to statistically control for differences in overall brain size. Dependent variables consisted of the combined left and right volumes for each of the four lobes of the brain (i.e., parietal, occipital, temporal, and frontal) as well as the cerebellum. The Wilks’s lambda of 0.51 (F=4.3, df=5, 23, p<0.01) indicated a unique pattern of cerebral morphological variation that distinguished the children with velocardiofacial syndrome from the comparison subjects.
Follow-up ANOVAs were performed to specify the regional differences. After adjustment for differences in total tissue volume, results indicated that frontal lobe tissue volume was relatively conserved in the children with velocardiofacial syndrome (figure 1). Analysis of least squares means indicated that the velocardiofacial syndrome group had proportionately larger frontal lobe volumes (403 cm3, SD=15.7) than the comparison group (378 cm3, SD=15.7). Group differences in parietal lobe volume (left plus right) were nonsignificant (F=3.7, df=1, 27, p=0.07). No group differences were detected when comparing volumes of temporal and occipital lobe tissue. In summary, our initial neuroimaging findings support the hypothesis of neuromorphological variation in brain development of children and adolescents with velocardiofacial syndrome.
Symmetry of Tissue Volume
No group differences in symmetry were observed for hemispheric, frontal lobe, temporal lobe, occipital lobe, or cerebellar volumes. However, differences in symmetry were detected in the parietal lobe (F=5.5, df=1, 28, p=0.02), with velocardiofacial syndrome subjects showing a right-greater-than-left asymmetry that was not present in the comparison subjects (table 1). Thus, it appeared that abnormal parietal tissue asymmetry in the velocardiofacial syndrome group was due to greater volume reduction in the left parietal lobe. This hypothesis also was supported by ANCOVA (with total brain tissue as a covariate) that showed disproportionate reduction in parietal lobe tissue volume in the left parietal lobe (F=6.2, df=1, 27, p=0.02) but not in the right (F=1.4, df=1, 27, p=0.25) in the children with velocardiofacial syndrome.
Group Differences in Tissue Composition
On the basis of our initial findings, further segmentation of gray matter, white matter, and CSF was conducted to explore group differences in total brain volumes and in two regions of interest: the frontal and parietal lobes. The 7.5% reduction of total gray matter volume in the brain (F=4.4, df=1, 28, p<0.05, ANOVA) was less than the 16.3% reduction seen in white matter volume (F=12.4, df=1, 28, p<0.005). The gross level of gray tissue volume in the frontal lobe was smaller in the children with velocardiofacial syndrome than in the comparison subjects. However, ANCOVA results indicated enlarged gray matter volume in this region relative to the general reduction in brain gray matter volume that occurred in individuals with velocardiofacial syndrome (F=4.5, df=1, 27, p<0.05). No differences were detected between groups for total parietal lobe (right plus left) gray matter composition.
After covarying for total cerebral white matter, children with velocardiofacial syndrome had proportionally larger frontal lobe white matter volumes than did the comparison subjects (F=16.7, df=1, 27, p<0.01). No group differences were detected in volumes of white matter tissue in the parietal lobe (F=1.9, df=1, 27, p=0.18, ANCOVA).
Symmetry in Tissue Composition
Repeated measures ANOVA tested group differences in symmetry for gray and white matter regions. Compared to the healthy subjects, children with velocardiofacial syndrome showed a rightward predominance of parietal lobe gray matter (F=4.4, df=1, 28, p=0.04) that was due to a disproportionate reduction in left parietal gray matter (F=7.0, df=1, 27, p=0.01, ANCOVA), and a leftward predominance in cerebellar white matter (F=4.9, df=1, 28, p=0.03) that was due to a relative decrease in right cerebellar white matter (F=4.2, df=1, 27, p=0.05).
Ventricular Volume
Because alterations in ventricular fluid volumes are the most common finding in imaging studies on schizophrenia (21), we analyzed group differences for this measure. The distributions violated the assumptions of normality and homogeneity of variance; therefore, a nonparametric test (Mann-Whitney U) was employed. A ventricle-to-brain-volume ratio was computed to account for differences in total brain volume. The results indicated a significant difference between the diagnostic categories (U=33, p=0.001, N=15 in each group), with greater ventricle-to-brain-volume ratios present in the children with velocardiofacial syndrome.
DISCUSSION
This preliminary study is the first to report regional brain volumes and tissue compositions for a cohort of children and adolescents with a diagnosis of velocardiofacial syndrome confirmed by chromosomal deletion at 22q11.2. Our findings indicate that subjects with velocardiofacial syndrome experience 1) a decrease in overall brain volume due to diminution in volumes of both cerebral gray and white matter; 2) a relative enlargement of the frontal lobe after adjustment for total brain volume, 3) a decrease in tissue volume in the left parietal lobe primarily attributable to disproportionate reduction of gray matter in this region, and 4) a decrease in right cerebellar tissue volume due to a disproportionate reduction in white matter for this area.
Consistent with our finding of overall brain volume differences, previous clinical studies have reported a higher rate of microcephaly (40%) in individuals with velocardiofacial syndrome (7). It is of interest that frontal lobe brain volume was enlarged in subjects with velocardiofacial syndrome. Reiss et al. (29) showed that IQ is positively correlated with total cerebral volume in children and, in particular, with the volume of cortical gray matter in the prefrontal region of the brain. This might explain why, despite a significantly lower overall brain volume (11% lower than comparison subjects), the majority of children and adolescents with velocardiofacial syndrome have borderline IQ rather than the mental retardation (10) observed in other disorders associated with microcephaly, e.g., Rett (30, 31) or Williams (32) syndromes.
In contrast to our findings, previous qualitative MRI examinations have not implicated parietal lobe abnormalities in the sensorimotor or cognitive deficits observed in individuals with velocardiofacial syndrome. However, neurocognitive assessments of individuals with this genetic condition have reported learning and language difficulties, as well as lower performance in abstract reasoning tasks such as arithmetic and mathematics (7, 9, 10, 33). Thus, at least part of the cognitive phenotype associated with velocardiofacial syndrome would appear to be consistent with parietal lobe dysfunction. The crucial role of the parietal lobe in memory has been demonstrated in functional imaging studies (34). An increase in activity has been observed in the parietal lobe region during episodic memory retrieval (35), working memory tasks, implicit or explicit recognition memory (36), and long-term memory consolidation (37). Consequently, parietal lobe aberration may damage information storage and retrieval and may contribute to learning difficulties. Specific deficits in language could also be partially explained by alteration in parietal lobe function. Structurally, lesions of the inferior parietal lobe are the most common site that produces conduction aphasia (38). Also, functional imaging studies have demonstrated the involvement of this region of the brain during semantic processing of words (39, 40). However, the temporal lobe, also implicated in speech and language processes through functional MRI studies (41), did not show significant volumetric alteration in the present group of subjects with velocardiofacial syndrome.
Besides advancing knowledge of velocardiofacial syndrome, research on this condition may contribute to a better understanding of schizophrenia and related disorders in the general population. Shprintzen (14) and other authors (5, 7) have reported a higher risk of developing schizophrenia in patients with velocardiofacial syndrome. Karayiorgou et al. (42) even suggested that the velocardiofacial syndrome deletion on chromosome 22q11.2 could be encompassing one of the genes responsible for schizophrenia and could be an unrecognized cause. Further, a recent clinical study of 10 velocardiofacial syndrome patients with psychosis (43) described nine out of 10 patients with schizophrenia and one with schizoaffective disorder. Their disorders were severe and associated with behavioral features such as impulsivity, unpredictability, temper outbursts, and physical aggression. The mean age at onset of psychosis in the Bassett et al. study (43) was 19.6 years (SD=4.6), which is younger than that usually reported for schizophrenia. Earlier onset is predictive of more severe illness, is associated with higher genetic risk and cognitive deficits (44, 45), and is more likely to be accompanied by changes in brain structure (46).
Given the overlap in clinical psychiatric features, it is interesting to analyze the similarities between the present imaging findings with velocardiofacial syndrome and earlier reports on the structural changes in the brains of individuals with schizophrenia (21). Previous studies on schizophrenia have reported decrease in overall brain size (27, 47), especially in early-onset schizophrenia (46). Jacobsen et al. (46) hypothesized that “this might reflect a more severe genetic and/or environmental neurodevelopmental insult, leading to earlier onset.” Frontal lobe abnormalities have been observed in persons with schizophrenia and usually involve a slight reduction in this structure (22). Our velocardiofacial syndrome subjects also showed frontal lobe alteration. However, the changes were in a counterintuitive direction, since we found that this region was actually enlarged.
While only a few studies have evaluated parietal lobe morphology in schizophrenia, they have indicated a volume reduction in this region (48, 49). Pearlson et al. (50) observed that the functions impaired in schizophrenic patients (e.g., language, working memory, oculomotor pursuit eye movements, focusing, and execution of attention) may be mediated by parietal lobe regions. Moreover, the most common imaging finding among schizophrenic patients is enlarged ventricles (21). Similarly, we report a significant increase in the ratio of ventricular CSF to brain volume in subjects with velocardiofacial syndrome. We hypothesize that this finding is most likely due to the loss of brain tissue, especially white matter, during brain development in individuals with velocardiofacial syndrome. Because of the conjunction of reported language disabilities in velocardiofacial syndrome and descriptions of temporal lobe reduction in schizophrenia, this structure was considered to be an a priori candidate for morphological alterations that may underlie the neurobehavioral phenotype associated with the deletion at chromosome 22q11.2. However, temporal lobe volume was not significantly altered in our velocardiofacial syndrome subjects. It is also possible that temporal lobe function may still be affected in this condition in the absence of structural abnormalities. Advances in the methodology of functional brain imaging will facilitate our understanding of how specific morphological changes, such as enlarged frontal and reduced parietal lobes, influence functional connectivity and cerebral activation in subjects with velocardiofacial syndrome.
In summary, this brain imaging study adds incremental knowledge and specificity to our understanding of specific neurodevelopmental variations that are likely to be observed in children and adolescents with velocardiofacial syndrome. The overall brain tissue reduction and the more specific loss of parietal gray matter may be associated with a component of the neurobehavioral phenotype observed in patients with velocardiofacial syndrome. Limitations of the current study include a small study group size and multiple statistical comparisons, thereby incurring the risk of an inflated type 1 error rate. Further studies with larger study groups should 1) subdivide the parietal lobe to examine whether particular subregions account for the observed morphological variation; 2) investigate the structure and function of the hippocampus, given its known connections with the parietal lobe and its role in memory and learning; and 3) analyze the structure of white matter tracks, since this tissue is the most affected by the deletion at chromosome 22q11.2.
Finally, longitudinal imaging studies of brain structure and function in individuals with velocardiofacial syndrome may provide an important model for understanding the neurobiology and neurodevelopmental course of schizophrenia. Ideally, functional imaging studies in the velocardiofacial syndrome population at high risk for developing schizophrenia will reveal activation patterns predictive of future disorder.
Received Feb. 4, 1999; revisions received July 14 and Sept. 30, 1999; accepted Oct. 1, 1999From the Department of Psychiatry and Behavioral Sciences, Stanford University School of Medicine. Address reprint requests to Dr. Eliez, Department of Psychiatry and Behavioral Sciences, Stanford University School of Medicine, 401 Quarry Rd., Stanford, CA 94305-5719; [email protected] (e-mail). Supported by a grant from the National Swiss Research Fund (Dr. Eliez); and grants from NIMH (MH-01142), the National Institute of Child Health and Human Development (HD-31715), and a Medical Investigation of Neurodevelopmental Disorders grant (Dr. Reiss). The authors thank Christine Blasey, Ph.D., for assistance with statistical analysis and editing, and Carol A. Lin, Anil Patwardhan, and Ilana Warsofsky for their image processing work.
![]() |
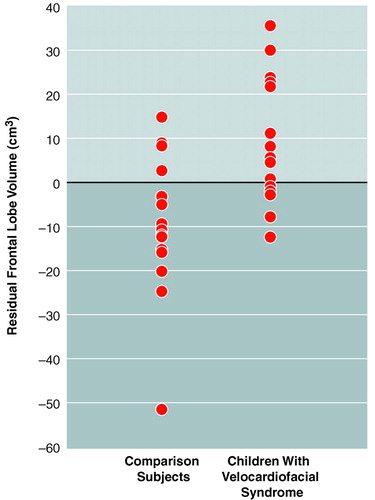
FIGURE 1. Residual Frontal Lobe Volumesa of 15 Children With and Without Velocardiofacial Syndrome
aFrontal lobe volume after adjustment for total brain volume.
1. Shprintzen RJ, Goldberg RB, Lewin ML, Sidoti EJ, Berkman MD, Argamaso RV, Young D: A new syndrome involving cleft palate, cardiac anomalies, typical facies, and learning disabilities: velo-cardio-facial syndrome. Cleft Palate J 1978; 15:56–62Medline, Google Scholar
2. Tezenas Du Montcel S, Mendizabai H, Ayme S, Levy A, Philip N: Prevalence of 22q11 microdeletion (letter). J Med Genet 1996; 33:719Crossref, Medline, Google Scholar
3. Scambler PJ, Kelly D, Lindsay E, Williamson R, Goldberg R, Shprintzen R, Wilson DI, Goodship JA, Cross IE, Burn J: Velo-cardio-facial syndrome associated with chromosome 22 deletions encompassing the DiGeorge locus. Lancet 1992; 339:1138–1139Google Scholar
4. Driscoll DA, Salvin J, Sellinger B, Budarf ML, McDonald-McGinn DM, Zackai EH, Emanuel BS: Prevalence of 22q11 microdeletions in DiGeorge and velocardiofacial syndromes: implications for genetic counselling and prenatal diagnosis. J Med Genet 1993; 30:813–817Crossref, Medline, Google Scholar
5. Lindsay EA, Greenberg F, Shaffer LG, Shapira SK, Scambler PJ, Baldini A: Submicroscopic deletions at 22q11.2: variability of the clinical picture and delineation of a commonly deleted region. Am J Med Genet 1995; 56:191–197Crossref, Medline, Google Scholar
6. Carlson C, Sirotkin H, Pandita R, Goldberg R, McKie J, Wadey R, Patanjali SR, Weissman SM, Anyane-Yeboa K, Warburton D, Scambler P, Shprintzen R, Kucherlapati R, Morrow BE: Molecular definition of 22q11 deletions in 151 velo-cardio-facial syndrome patients. Am J Hum Genet 1997; 61:620–629Crossref, Medline, Google Scholar
7. Goldberg R, Motzkin B, Marion R, Scambler PJ, Shprintzen RJ: Velo-cardio-facial syndrome: a review of 120 patients. Am J Med Genet 1993; 45:313–319Crossref, Medline, Google Scholar
8. Ryan AK, Goodship JA, Wilson DI, Philip N, Levy A, Seidel H, Schuffenhauer S, Oechsler H, Belohradsky B, Prieur M, Aurias A, Raymond FL, Clayton-Smith J, Hatchwell E, McKeown C, Beemer FA, Dallapiccola B, Novelli G, Hurst JA, Ignatius J, Green AJ, Winter RM, Brueton L, Brondum-Nielsen K, Scambler PJ: Spectrum of clinical features associated with interstitial chromosome 22q11 deletions: a European collaborative study. J Med Genet 1997; 34:798–804Crossref, Medline, Google Scholar
9. Golding-Kushner KJ, Weller G, Shprintzen RJ: Velo-cardio-facial syndrome: language and psychological profiles. J Craniofac Genet Dev Biol 1985; 5:259–266Medline, Google Scholar
10. Swillen A, Devriendt K, Legius E, Eyskens B, Dumoulin M, Gewillig M, Fryns JP: Intelligence and psychosocial adjustment in velocardiofacial syndrome: a study of 37 children and adolescents with VCFS. J Med Genet 1997; 34:453–458Crossref, Medline, Google Scholar
11. Meinecke P, Beemer FA, Schinzel A, Kushnick T: The velo-cardio-facial (Shprintzen) syndrome: clinical variability in eight patients. Eur J Pediatr 1986; 145:539–544Crossref, Medline, Google Scholar
12. Shprintzen RJ: Birth Defects Encyclopedia. Edited by Boyce ML. Dover, Mass, Center for Birth Defects Information Services, 1990, pp 1744–1745Google Scholar
13. Chow EW, Bassett AS, Weksberg R: Velo-cardio-facial syndrome and psychotic disorders: implications for psychiatric genetics. Am J Med Genet 1994; 54:107–112Crossref, Medline, Google Scholar
14. Shprintzen RJ, Goldberg R, Golding-Kushner KJ, Marion RW: Late-onset psychosis in the velo-cardio-facial syndrome (letter). Am J Med Genet 1992; 42:141–142Crossref, Medline, Google Scholar
15. Papolos DF, Faedda GL, Veit S, Goldberg R, Morrow B, Kucherlapati R, Shprintzen RJ: Bipolar spectrum disorders in patients diagnosed with velo-cardio-facial syndrome: does a hemizygous deletion of chromosome 22q11 result in bipolar affective disorder? Am J Psychiatry 1996; 153:1541–1547Google Scholar
16. Carlson C, Papolos D, Pandita RK, Faedda GL, Veit S, Goldberg R, Shprintzen R, Kucherlapati R, Morrow B: Molecular analysis of velo-cardio-facial syndrome patients with psychiatric disorders. Am J Hum Genet 1997; 60:851–859Medline, Google Scholar
17. Mitnick RJ, Bello JA, Shprintzen RJ: Brain anomalies in velo-cardio-facial syndrome. Am J Med Genet 1994; 54:100–106Crossref, Medline, Google Scholar
18. Altman DH, Altman NR, Mitnick RJ, Shprintzen RJ: Further delineation of brain anomalies in velo-cardio-facial syndrome. Am J Med Genet 1995; 60:174–175Crossref, Medline, Google Scholar
19. Devriendt K, Thienen MN, Swillen A, Fryns JP: Cerebellar hypoplasia in a patient with velo-cardio-facial syndrome. Dev Med Child Neurol 1996; 38:949–953Crossref, Medline, Google Scholar
20. Lynch DR, McDonald-McGinn DM, Zackai EH, Emanuel BS, Driscoll DA, Whitaker LA, Fischbeck KH: Cerebellar atrophy in a patient with velocardiofacial syndrome. J Med Genet 1995; 32:561–563Crossref, Medline, Google Scholar
21. Shenton ME, Wible CG, McCarley RW: A Review of Magnetic Resonance Imaging Studies of Brain Abnormalities in Schizophrenia. New York, Marcel Dekker, 1997Google Scholar
22. Lawrie SM, Abukmeil SS: Brain abnormality in schizophrenia: a systematic and quantitative review of volumetric magnetic resonance imaging studies. Br J Psychiatry 1998; 172:110–120Crossref, Medline, Google Scholar
23. Moss EM, Batshaw ML, Solot CB, Gerdes M, McDonald-McGinn DM, Driscoll DA, Emanuel BS, Zackai EH, Wang PP: Psychoeducational profile of the 22q11.2 microdeletion: a complex pattern. J Pediatr 1999; 134:193–198Crossref, Medline, Google Scholar
24. Reiss AL, Hennessey JG, Rubin M, Beach L, Abrams MT, Warsofsky IS, Liu AM, Links JM: Reliability and validity of an algorithm for fuzzy tissue segmentation of MRI. J Comput Assist Tomogr 1998; 22:471–479Crossref, Medline, Google Scholar
25. Kaplan DM, Liu AM, Abrams MT, Warsofsky IS, Kates WR, White CD, Kaufmann WE, Reiss AL: Application of an automated parcellation method to the analysis of pediatric brain volumes. Psychiatry Res 1997; 76:15–27Crossref, Medline, Google Scholar
26. Subramaniam B, Hennessey JG, Rubin MA, Beach LS, Reiss AL: Software and methods for quantitative imaging in neuroscience: the Kennedy Krieger Institute Human Brain Project, in Neuroinformatics: An Overview of the Human Brain Project. Edited by Koslow SH, Huerta MF. Mahwah, NJ, Lawrence Erlbaum Associates, 1997, pp 335–360Google Scholar
27. Andreasen NC, Rajarethinam R, Cizadlo T, Arndt S, Swayze VW II, Flashman LA, O’Leary DS, Ehrhardt JC, Yuh WTC: Automatic atlas-based volume estimation of human brain regions from MR images. J Comp Assist Tomogr 1996; 20:98–106Crossref, Medline, Google Scholar
28. Talairach J, Tournoux P: Co-Planar Stereotaxic Atlas of the Human Brain. New York, Thieme Medical, 1988Google Scholar
29. Reiss AL, Abrams MT, Singer HS, Ross JL, Denckla MB: Brain development, gender and IQ in children: a volumetric imaging study. Brain 1996; 119(part 5):1763–1774Google Scholar
30. Reiss AL, Faruque F, Naidu S, Abrams M, Beaty T, Bryan RN, Moser H: Neuroanatomy of Rett syndrome: a volumetric imaging study. Ann Neurol 1993; 34:227–234Crossref, Medline, Google Scholar
31. Subramaniam B, Naidu S, Reiss AL: Neuroanatomy in Rett syndrome: cerebral cortex and posterior fossa. Neurology 1997; 48:399–407Crossref, Medline, Google Scholar
32. Lenhoff HM, Wang PP, Greenberg F, Bellugi U: Williams syndrome and the brain. Sci Am 1997; 277:68–73Crossref, Medline, Google Scholar
33. Kok LL, Solman RT: Velocardiofacial syndrome: learning difficulties and intervention. J Med Genet 1995; 32:612–618Crossref, Medline, Google Scholar
34. Ungerleider LG: Functional brain imaging studies of cortical mechanisms for memory. Science 1995; 270:769–775Crossref, Medline, Google Scholar
35. Shallice T, Fletcher P, Frith CD, Grasby P, Frackowiak RS, Dolan RJ: Brain regions associated with acquisition and retrieval of verbal episodic memory. Nature 1994; 368:633–635Crossref, Medline, Google Scholar
36. Rugg MD, Mark RE, Walla P, Schloerscheidt AM, Birch CS, Allan K: Dissociation of the neural correlates of implicit and explicit memory. Nature 1998; 392:595–598Crossref, Medline, Google Scholar
37. Shadmehr R, Holcomb HH: Neural correlates of motor memory consolidation. Science 1997; 277:821–825Crossref, Medline, Google Scholar
38. Goodglass H: Understanding Aphasia. New York, Academic Press, 1993Google Scholar
39. Vandenberghe R, Price C, Wise R, Josephs O, Frackowiak RS: Functional anatomy of a common semantic system for words and pictures. Nature 1996; 383:254–256Crossref, Medline, Google Scholar
40. Schlosser R, Hutchinson M, Joseffer S, Rusinek H, Saarimaki A, Stevenson J, Dewey SL, Brodie JD: Functional magnetic resonance imaging of human brain activity in a verbal fluency task. J Neurol Neurosurg Psychiatry 1998; 64:492–498Crossref, Medline, Google Scholar
41. Binder JR: Neuroanatomy of language processing studied with functional MRI. Clin Neurosci 1997; 4:87–94Crossref, Medline, Google Scholar
42. Karayiorgou M, Morris MA, Morrow B, Shprintzen RJ, Goldberg R, Borrow J, Gos A, Nestadt G, Wolyniec PS, Lasseter VK: Schizophrenia susceptibility associated with interstitial deletions of chromosome 22q11. Proc Natl Acad Sci USA 1995; 92:7612–7616Google Scholar
43. Bassett AS, Hodgkinson K, Chow EW, Correia S, Scutt LE, Weksberg R:22q11 deletion syndrome in adults with schizophrenia. Am J Med Genet 1998; 81:328–337Google Scholar
44. Russell AJ, Munro JC, Jones PB, Hemsley DR, Murray RM: Schizophrenia and the myth of intellectual decline. Am J Psychiatry 1997; 154:635–639Link, Google Scholar
45. Hafner H, an der Heiden W: Epidemiology of schizophrenia. Can J Psychiatry 1997; 42:139–151Crossref, Medline, Google Scholar
46. Jacobsen LK, Giedd JN, Vaituzis AC, Hamburger SD, Rajapakse JC, Frazier JA, Kaysen D, Lenane MC, McKenna K, Gordon CT, Rapoport JL: Temporal lobe morphology in childhood-onset schizophrenia. Am J Psychiatry 1996; 153:355–361; correction, 153:851Link, Google Scholar
47. Gur RE, Mozley PD, Shtasel DL, Cannon TD, Gallacher F, Turetsky B, Grossman R, Gur RC: Clinical subtypes of schizophrenia: differences in brain and CSF volume. Am J Psychiatry 1994; 151:343–350Link, Google Scholar
48. Andreasen NC, Flashman L, Flaum M, Arndt S, Swayze V II, O’Leary DS, Ehrhardt JC, Yuh WTC: Regional brain abnormalities in schizophrenia measured with magnetic resonance imaging. JAMA 1994; 272:1763–1769Google Scholar
49. Schlaepfer TE, Harris GJ, Tien AY, Peng LW, Lee S, Federman EB, Chase GA, Barta PE, Pearlson GD: Decreased regional cortical gray matter volume in schizophrenia. Am J Psychiatry 1994; 151:842–848Link, Google Scholar
50. Pearlson GD, Petty RG, Ross CA, Tien AY: Schizophrenia: a disease of heteromodal association cortex? Neuropsychopharmacology 1996; 14:1–17Google Scholar