Effect of 7,8-Dihydroxyflavone, a Small-Molecule TrkB Agonist, on Emotional Learning
Abstract
Objective:
Despite increasing awareness of the many important roles played by brain-derived neurotrophic factor (BDNF) activation of TrkB, a fuller understanding of this system and the use of potential TrkB-acting therapeutic agents has been limited by the lack of any identified small-molecule TrkB agonists that fully mimic the actions of BDNF at brain TrkB receptors in vivo. However, 7,8-dihydroxyflavone (7,8-DHF) has recently been identified as a specific TrkB agonist that crosses the blood-brain barrier after oral or intraperitoneal administration. The authors combined pharmacological, biochemical, and behavioral approaches in a preclinical study examining the role of 7,8-DHF in modulating emotional memory in mice.
Method:
The authors first examined the ability of systemic 7,8-DHF to activate TrkB receptors in the amygdala. They then examined the effects of systemic 7,8-DHF on acquisition and extinction of conditioned fear, using specific and well-characterized BDNF-dependent learning paradigms in several models using naive mice and mice with prior traumatic stress exposure.
Results:
Amygdala TrkB receptors, which have previously been shown to be required for emotional learning, were activated by systemic 7,8-DHF (at 5 mg/kg i.p.). 7,8-DHF enhanced both the acquisition of fear and its extinction. It also appeared to rescue an extinction deficit in mice with a history of immobilization stress.
Conclusions:
These data suggest that 7,8-DHF may be an excellent agent for use in understanding the effects of TrkB activation in learning and memory paradigms and may be attractive for use in reversing learning and extinction deficits associated with psychopathology.
Brain-derived neurotrophic factor (BDNF) is widely distributed throughout the adult brain. BDNF binds to the nonselective p75 receptor, common to a variety of neurotrophins, as well as to its specific high-affinity TrkB receptor. There is evidence that BDNF plays a critical role in long-term potentiation (1) and is a molecular mediator of learning and memory. Intrahippocampal infusion of BDNF in vivo and BDNF infusion in hippocampal slices induce long-lasting enhancement of synaptic plasticity (2–4). In addition, BDNF has been shown to be involved in declarative and spatial memory processes (5, 6). For these reasons, BDNF is an attractive candidate for modulating learning and memory processes.
Deprivation of endogenous BDNF causes impairment of spatial learning and memory in rats (7), and forebrain-restricted deletion of BDNF severely impairs spatial learning (8). Similarly, BDNF and TrkB are both known to play an important role in fear memory acquisition and extinction (9, 10). We previously demonstrated that disruption of TrkB activation using lentiviral expression of a dominant-negative form of TrkB into the basolateral amygdala blocked the acquisition of fear (11) and the consolidation of extinction (12), which suggests that BDNF-dependent activation of TrkB in the amygdala regulates the learning of fear and extinction memories.
Recent studies indicate that BDNF polymorphisms may be implicated in emotionality and anxiety disorders (13, 14). In addition to the role of the BDNF/TrkB system in learning, memory, and synaptic plasticity, alterations within the system have been implicated in affective disorders (15). Despite this increasing awareness of the many important roles played by BDNF activation of TrkB, a fuller understanding of this system and the use of potential TrkB-acting therapeutic agents has been limited by the lack of any identified small-molecule TrkB agonists that fully mimic the actions of BDNF at brain TrkB receptors in vivo.
Jang et al. (16) recently screened a chemical library for compounds that activate TrkB in vitro and discovered that a series of flavone derivatives, most potently 7,8-dihydroxyflavone (7,8-DHF), significantly activated TrkB. They found that 7,8-DHF binds in vitro with high affinity to the TrkB receptor and provokes its dimerization and autophosphorylation, leading to downstream signaling cascade activation. Systemic administration of 7,8-DHF protects neurons in wild-type but not TrkB-deficient or mutated (F616A) mice from apoptosis, substantially activates TrkB in the brain 2 hours after injection, inhibits kainic acid-induced neuronal cell death, decreases infarct volumes in stroke in a TrkB-dependent manner, is neuroprotective in an animal model of Parkinson's disease (16), and rescues a BDNF deficit in a BDNF cortex-specific knockout murine model (17). To more fully examine the effects of this newly identified TrkB agonist, we examined the effects of 7,8-DHF on the acquisition and extinction of cue-dependent conditioned fear among the most simple yet robust rodent models of emotional learning and memory.
Method
Animals
All experiments were performed on adult (2-4 months old) wild-type strain C57BL/6J male mice from Jackson Laboratory (Bar Harbor, Me.). All procedures were approved by the Institutional Animal Care and Use Committee of Emory University and were in compliance with National Institutes of Health guidelines. Separate cohorts of animals were used in each experiment.
Drugs
We administered 7,8-DHF (obtained both from coauthor K.Y. and from Tokyo Chemical Industry, catalog no. D1916) intraperitoneally at a dose of 5 mg/kg in a vehicle of 17% dimethylsulfoxide in phosphate-buffered saline; the same vehicle was also used in control groups. In those experiments in which 7,8-DHF was given, mice received a single dose 1 hour before the appropriate behavioral procedure.
Immunoblotting, Immunohistochemistry, and Autoradiography
For Western blots, mice were injected with 7,8-DHF at 5 mg/kg i.p.; they were sacrificed 1 hour or 2 hours later, and amygdala tissue was collected bilaterally. Immunoblot analysis was performed with anti-TrkB Y706 (phosphorylated TrkB; Santa Cruz Biotechnology, Santa Cruz, Calif.; 1:200 in 5% bovine serum albumin/phosphate-buffered saline), and anti-TrkB (Cell Signaling Technology, Danvers, Mass.; 1:500 in 5% bovine serum albumin/phosphate-buffered saline), anti-p-mitogen-activated protein kinase (MAPK; Cell Signaling Technology; 1:500 in 5% milk/phosphate-buffered saline), and anti-MAPK (Cell Signaling Technology; 1:5,000 in 5% milk/phosphate-buffered saline). For immunohistochemistry, fixed rat brain sections from prior studies (11) were incubated and blocked in phosphate-buffered saline, goat serum, and Triton X-100 and incubated with TrkB rabbit polyclonal antibody (1:500, SC-12, Santa Cruz Biotechnology) and an anti-rabbit biotinylated secondary antibody (1:500) for 2 hours. Avidin-biotin complexes were amplified using a standard Vectastain Elite ABC kit (Vector Laboratories, Burlingame, Calif.). For regional localization of 7,8-DHF binding to TrkB, 7,8-DHF binding was performed as previously described (18).
Cue Fear Conditioning and Extinction
Fear conditioning was conducted in nonrestrictive acrylic cylinders (SR-LAB startle response system, San Diego Instruments) located in a ventilated, sound-attenuated chamber. The footshock unconditioned stimulus was delivered through a stainless steel grid floor. Shock reactivity was defined as the peak activity (measured with a piezoelectric accelerometer) that occurred during the 200 msec after the onset of the unconditioned stimulus. The tone-conditioned stimulus was generated by a Tektronix function generator audio oscillator and delivered through a high-frequency speaker (9, 19).
Note that fear conditioning includes a longer intertrial interval (5 minutes) between each of five conditioned stimuli to maximize fear learning, as compared to a greater number of stimuli (ranging from 15 to 30) for fear expression and extinction protocols, which use shorter intertrial intervals of 30-90 seconds. On each of 2 days prior to training, mice were given a 10-minute startle chamber exposure session to habituate them to handling and context.
During cued fear conditioning, mice received five trials of a conditioned stimulus tone (30 seconds, 12 kHz, 70 dB) coterminating with an unconditioned stimulus footshock (500 msec, 0.5 mA in experiments 1 and 2; 500 ms, 1 mA in experiments 3 and 4) with an intertrial interval of 5 minutes. The expression of fear (in a different context from training) was assessed 24 hours after fear conditioning and consisted of 15 conditioned stimulus tone trials of 30 seconds each, with a 1.5-minute intertrial interval. For extinction testing in experiments 2 and 4, mice were given 30 conditioned stimulus tone trials with a 30-second intertrial interval. Fifteen conditioned stimuli were presented in experiment 3 in the extinction session. Stimulus presentation and data acquisition were controlled and digitized by, and stored in, an interfacing desktop computer using SR-LAB and analyzed with the FreezeView software program (Coulbourn Instruments, Whitehall, Pa.).
Immobilization Stress
Immobilization procedures were conducted in a room separate from fear training and testing apparatus. Each animal was immobilized by gently restraining its four limbs in a prone position to metal arms attached to a wooden board for 2 hours. All animals of the same cage received the same treatment—either immobilization or handling. After treatment, animals were returned to their home cage and remained undisturbed until fear training.
More methodological details are provided in the data supplement that accompanies the online edition of this article.
Results
Activation Effect of Systemic 7,8-DHF on TrkB Receptors in the Amygdala
We first confirmed that 7,8-DHF activated amygdala p-TrkB in vivo. Mice were given vehicle or 7,8-DHF systemically (5 mg/kg i.p.) either 1 or 2 hours prior to sacrifice. Immunoblots were then performed in duplicate on vehicle-treated and 7,8-DHF-treated amygdala tissue (Figure 1A,B). We found that the total level of TrkB protein did not change, but systemic 7,8-DHF led to robust increases in p-TrkB (Y706). To further demonstrate activation of the TrkB signaling pathway, we found that p-MAPK was activated within the amygdala 1 hour and 2 hours after administration of 7,8-DHF, again with no change in total MAPK.

a MAPK=mitogen-activated protein kinase. Panel A shows immunoblots of mouse amygdala punches examining total TrkB protein (top), activated p-TrkB (second row), total MAPK (third row), and activated p-MAPK (bottom row). Each condition is represented in duplicate, with amygdala punches from mice injected intraperitoneally with vehicle (control) or 7,8-DHF (5 mg/kg) 1 hour or 2 hours prior to sacrifice. Full-length TrkB is detected at both ∼95 kDa (nonglycosylated) and ∼140-145 kDa (glycosylated) forms. Although total levels of TrkB and MAPK do not change, systemic 7,8-DHF led to robust activation/phosphorylation of amygdala TrkB (p-TrkB Y706) and MAPK (p-MAPK). Panel B shows the quantification (mean values with standard deviations) of the p-TrkB706:TrkB ratio represented in the top two immunoblots of panel A, demonstrating increased p-TrkB706 with 7,8-DHF treatment. In panel C, receptor autoradiography demonstrates that 3H-7,8-DHF binds to brain regions known to express TrkB protein, including the amygdala (arrow). Panel D shows in situ hybridization of TrkB mRNA expression from the Allen Brain Atlas (www.allenbrainatlas.com). In panel E, 3H-7,8-DHF shows no significant binding when tissue is pretreated with excess cold 7,8-DHF, suggesting relative specificity. Panel F shows dense expression of TrkB protein on pyramidal neurons in basolateral rat amygdala identified with immunocytochemistry. Scale bar represents ∼50 mm.
We next confirmed the autoradiography receptor binding pattern of 3H-7,8-DHF (Kd=320 nM [16]) as shown in Figure 1C. The binding pattern resembles the known mRNA expression pattern of the TrkB receptor (Figure 1D), and this binding was prevented with excess cold -7,8-DHF (Figure 1E). These data are consistent with previous data indicating that systemic 7,8-DHF activates p-TrkB at endogenous TrkB receptors in the mouse brain (16). Additionally, pyramidal neurons in the rodent amygdala appear to express high levels of TrkB receptor, as determined by immunohistochemistry (Figure 1F). Together, these data confirm that amygdala TrkB receptors are prevalent, bind to 7,8-DHF in vivo, and are activated by systemic administration of 7,8-DHF.
Effect of Systemic 7,8-DHF on Learning of Conditioned Fear
Based on previous in vitro and in vivo studies (16, 17), we anticipated that 5 mg/kg of 7,8-DHF would be the optimal dose for mouse learning experiments. However, we performed a dose-response study to directly compare the effects of 0, 1, 5, and 25 mg/kg i.p. of 7,8-DHF during the averaged first three fear-conditioning stimuli in mice (Figure 2A). We found an overall significant drug effect with analysis of variance (ANOVA) (linear trend, F=2.7, df=1, 39, one-tailed p≤0.05). Post hoc analyses revealed that mice treated with 5 mg/kg were significantly more fearful than those that received only vehicle (one-tailed t test, p≤0.05). Thus, we used 5 mg/kg for the remaining studies.

a Panel A shows results of a dose-response study performed with 7,8-DHF (N=10 in each dose group). The graph shows the average proportion of animals freezing during the first three conditioned stimulus trials (#p≤0.05 relative to vehicle [control]). Panel B shows the mean total distance and the distance traveled in the center of the open field for 10 minutes with 7,8-DHF or vehicle injected 1 hour before testing; there were no differences between groups. Panel C shows the mean shock reactivity in the startle apparatus during the cue-dependent fear conditioning; there were no differences between groups. Panel D shows the mean percentage of time spent freezing, in three conditioned stimulus trials per group (across the entire 15 conditioned stimuli), in testing for cue-dependent fear memory 24 hours after fear conditioning. Mice that had received 7,8-DHF prior to fear conditioning showed increased freezing. Panel E shows the mean percentage of time spent freezing within-session for each test trial for the first 10 trials of session. Within-session fear differences were particularly pronounced in the first conditioned stimulus trial of the testing session. Panel F shows that the mean total time spent freezing during testing (conditioned stimulus and no-stimulus intertrial periods) was significantly greater in the group of mice that had received 7,8-DHF 1 hour prior to fear conditioning. Error bars in panels B-F indicate standard deviations. In panels D-F, *p<0.05 between 7,8-DHF and vehicle groups.
We initially examined the behavioral effects of 7,8-DHF in an open-field maze after 7,8-DHF injection (5 mg/kg i.p., 1 hour prior to test). We found no differences between 7,8-DHF and vehicle on total distance traveled as a measure of baseline locomotion, nor did we find any differences in time traveled in center or surround (Figure 2B; less time spent in the center indicates anxiety-like behavior). These data suggest that acute dosing of systemic 7,8-DHF does not have any significant effects on locomotion or innate anxiety-like behavior.
We next performed Pavlovian fear conditioning (see behavioral experiment 1 in the online data supplement), pairing auditory cues to footshocks to examine whether this TrkB agonist would directly enhance fear learning. There were no differences in footshock reactivity in vehicle and drug groups when 7,8-DHF was administered 1 hour prior to training (Figure 2C), suggesting that 7,8-DHF does not affect pain sensitivity or the unconditioned stimulus representation. Twenty-four hours after fear conditioning, we examined the expression of conditioned fear in the absence of drug. When we examined freezing only on conditioned stimulus trials (grouping trials in bins of three; Figure 2D), we found overall fear increases in the 7,8-DHF group (F=5.1, df=1, 41, p≤0.05). This effect was particularly pronounced in the very first conditioned stimulus trial of the session (Figure 2E; repeated-measures ANOVA, group effect, F=4.8, df=1, 41, p<0.05; group-by-drug effect, F=3.2, df=9, 41, p<0.01). Examination of freezing during the first conditioned stimulus trial (trial 7) revealed differences between the vehicle and 7,8-DHF groups (p<0.05). We also found that the overall fear (freezing during both conditioned stimulus and intertrial no-stimulus periods) was significantly increased in the animals that had been treated with 7,8-DHF (Figure 2F; F=4.3, df=1, 41, p≤0.05). These results suggest that 7,8-DHF enhances new fear learning, a BDNF-dependent learning process, with no effect on overall activity, locomotion, anxiety-like behavior, or pain sensitivity.
Effect of 7,8-DHF on Extinction of Fear, a BDNF-Dependent Process
Extinction of fear is the gradual reduction of conditioned fear behavior to a conditioned stimulus when it is repeatedly presented in the absence of the unconditioned stimulus. Extinction has previously been shown to be BDNF dependent and to require new learning processes. We examined whether extinction in previously conditioned mice would also be enhanced with 7,8-DHF. Mice were first fear-conditioned and matched for similar levels of freezing behavior to the conditioned stimulus tone (see experiment 2 and Figure S1B in the online data supplement) when tested 24 hours later (pre-extinction). On the next day, groups were given either vehicle or 7,8-DHF (5 mg/kg i.p.) 1 hour prior to a suboptimal (15-trial) extinction training, which normally would not support robust extinction of fear. Compared to vehicle, 7,8-DHF supported extinction, as shown by a repeated-measures ANOVA comparing pre-extinction and within-extinction freezing (F=4.4, df=1, 24, p<0.05) (Figure 3A). Least-significant-difference post hoc analysis showed that the 7,8-DHF group froze less in the within-extinction session than did the vehicle group (p<0.05). More detailed analysis of the within-extinction session can be seen in Figure 3B, revealing a decreased level of conditioned freezing in drug-treated mice (F=5.2, df=1, 24, p<0.05). An additional post-extinction session performed 24 hours later led to a floor effect, with no subsequent freezing differences between groups (day 6, post-extinction session) (Figure 3C).
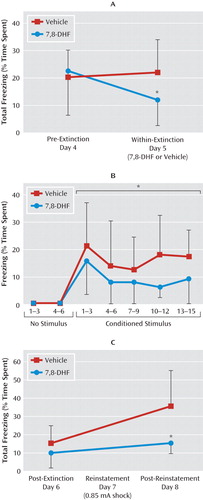
a Panel A shows the mean percentage of total time spent freezing during the testing. Pre-extinction was performed 24 hours after cue-dependent fear conditioning, and within-extinction (in the presence of drug) was performed 24 hours after that. Mice that received 7,8-DHF showed enhanced extinction of fear relative to those that received vehicle. Panel B shows the mean percentage of time spent freezing in response to conditioned stimulus presentation during the within-extinction session, represented in blocks of three trials. Mice that received 7,8-DHF 1 hour beforehand had similar initial freezing but showed rapid extinction of fear compared to those that received vehicle. In panel C, after all animals had been extinguished to a minimal level of fear (post-extinction test in the absence of drug, 24 hours after within-session), they were then subject to reinstatement (24 hours after post-extinction). Total freezing in post-extinction and post-reinstatement was compared between animals that had received 7,8-DHF or vehicle 1 hour before the extinction training. Error bars indicate standard deviations. *p<0.05 between the 7,8-DHF and vehicle groups.
We then examined reinstatement in previously treated 7,8-DHF and vehicle extinction groups by administering a footshock without the conditioned cue prior to cue fear retesting (Figure 3C; repeated-measures ANOVA, drug effect, F=6.0, df=1, 24, p<0.05). Least-significant-difference post hoc analysis showed that the vehicle group had spent more time freezing than the 7,8-DHF group in the post-reinstatement test on day 8 (p<0.05). Thus, mice that had received 7,8-DHF 1 hour before the within-extinction session (day 5) showed less conditioned freezing following reinstatement than the group that had received vehicle only. Note that no differences were observed in locomotor activity prior to and between tone exposure in this context. Together these data suggest that 7,8-DHF may enhance the extinction of conditioned fear and subsequently reduce the level of post-reinstatement fear following a footshock stressor.
Effect of 7,8-DHF on Fear Extinction in a Mouse Stress Model With Diminished Extinction
Fear-related psychopathology, such as posttraumatic stress disorder (PTSD), may be due in part to deficits in extinction of fear. We sought to examine whether 7,8-DHF might enhance extinction in a stress model in which a prior traumatic event produced a deficit in fear extinction. We used a mouse version of immobilization stress in which naive mice are restrained to immobilization boards for 2 hours. In rats this procedure has been shown to be quite stressful, detectable in the hypothalamic-pituitary-adrenal (HPA) axis and behaviorally for several weeks following a single session (20, 21). In the present study, naive mice with no pharmacological manipulation were fear-conditioned as described above, with tone-shock pairings 6 days after the immobilization procedure (see experiment 3 and Figure S1C in the online data supplement). We found that in mice, as in rats, immobilization stress leads to a rapid but transient increase in plasma corticosterone levels (Figure 4A; t=13.06, df=30, p<0.001), demonstrating the robust HPA-axis effects of this one-time stressor.
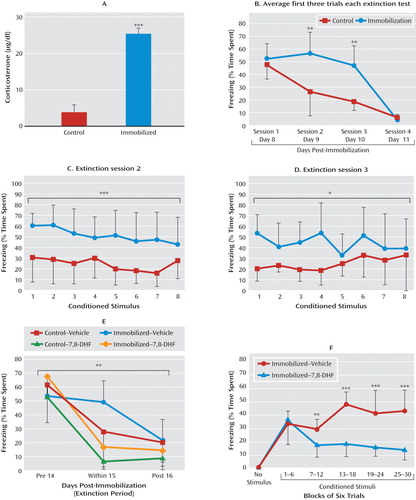
a Mice underwent 2-hour immobilization 6 days before the first session of fear conditioning, followed by repeated sessions of extinction. Panel A shows that shortly after immobilization, there is a robust activation of the hypothalamic-pituitary-adrenal axis, as demonstrated by a greater mean acute level of plasma corticosterone relative to control mice. In panel B, the immobilized group showed delayed extinction in analyses of conditioned freezing in extinction sessions 2 and 3 (panels C and D), whereas both groups had equivalent low levels of freezing by session 4, suggesting a delay in extinction following immobilization stress. (In panels A-D, ***p<0.001, **p<0.01, *p<0.05 between control and immobilization groups.) In panel E, animals also showed impaired extinction when fear-conditioned 12 days after immobilization stress. Because of the delayed nature of extinction in immobilization-treated animals, we examined the last session to compare differential freezing during pre-extinction, within-session extinction, and post-extinction sessions across groups (with and without immobilization, and with and without 7,8-DHF). Mice that were given 7,8-DHF prior to extinction showed a significant drug-by-session effect of enhancement of extinction across sessions (**p<0.01 session-by-group interaction between pre-extinction, within-extinction, and post-reinstatement). Panel F shows that 7,8-DHF was associated with decreased freezing in the within-session extinction trials in animals with a prior history of immobilization. Freezing (average of six trials within each bin) is shown during within-extinction session, 1 hour after administration of 7,8-DHF or vehicle (***p<0.001, **p<0.01 for the immobilization-vehicle compared with immobilization-7,8-DHF groups). Error bars indicate standard deviations.
Mice that have previously undergone immobilization have a significant deficit in fear extinction that is apparent when analyzing the first three trials of each session across the four extinction sessions (Figure 4B; repeated-measures ANOVA, session-by-group effect, F=59, df=3, 42, p<0.01; least-significant-difference post hoc analyses of sessions 2 and 3 revealed greater levels of freezing in the immobilization group than the control group, p<0.01). After examination of freezing sessions 2 and 3 with repeated-measures ANOVA, we found that the immobilization group showed more freezing than the control group in session 2 (F=616.52, df=1, 14, p<0.001) (Figure 4C) and session 3 (F=383.84, df=1, 14, p<0.05) (Figure 4D), but with no differences between groups in session 4.
We next examined whether 7,8-DHF would enhance extinction of conditioned fear in mice with a prior history of immobilization. We used a longer undisturbed period (12 days) after stress than in the previous experiment (6 days) to evaluate the longevity of the stress-dependent delayed extinction effect (see experiment 4 and Figure S1D in the online data supplement). Fifteen days after the immobilization session, mice were given vehicle or 7,8-DHF (5 mg/kg i.p.) 1 hour before their first period of extinction training. There were no differences among groups in non-cue-related locomotor activity, shock reactivity during training, or conditioned freezing prior to extinction training. However, when we examined previously immobilized animals with and without 7,8-DHF treatment, we found that extinction was significantly enhanced with 7,8-DHF (Figure 4E; session-by-drug interaction, F=17.6, df=2, 39, p<0.001). When we included the two control groups (same fear and extinction protocols, but no prior immobilization), we still found an overall between-session extinction effect (Figure 4E; session-by-group interaction, F=3.95, df=6, 84, p<0.01), with significant post hoc differences between immobilization with and without 7,8-DHF (p<0.001), control with and without 7,8-DHF (p<0.05), and immobilization and control groups (p<0.01). These data suggest that 7,8-DHF is effective both in naive animals and in those with prior traumatic stress. We then analyzed freezing in the within-extinction session specifically in immobilization-stressed animals (Figure 4F). We observed a drug effect, suggesting that the immobilization-vehicle group presented more freezing (or a deficit in extinction) than the immobilization-7,8-DHF group (F=21.6, df=1, 13, p<0.001; block-by-group interaction, F=9.7, df=5, 80, p<0.001).
Together with the other experiments, these data suggest that 7,8-DHF enhances extinction of fear in naive animals as well as in animals with extinction deficits due to prior traumatic exposure.
Discussion
We have demonstrated that 7,8-DHF, a systemic TrkB agonist, enhances emotional learning. We have also built on prior in vitro data demonstrating that 7,8-DHF is a novel specific agonist at the TrkB receptor and that it crosses the blood-brain barrier to activate TrkB receptors in the brain, even in the absence of endogenous BDNF (16, 17). Our data show that 7,8-DHF enhances several well-defined emotional learning and memory paradigms that have previously been shown to be BDNF dependent.
We first demonstrated that a single systemic dose of 7,8-DHF enhances the learning of cue-dependent fear conditioning in wild-type animals. This potentiated the fear response to the cue, suggesting that 7,8-DHF is likely targeting TrkB receptors in the brain that are normally critical for fear learning.
Extinction, or the specific new learning of fear inhibition, has also been shown to be BDNF dependent. We showed that 7,8-DHF could enhance extinction in wild-type mice. Clinical fear-related disorders have been shown to be related to a deficit in extinction, which may be related to the decreased BDNF found in a number of models of chronic stress. In our final experiment, we demonstrated that 7,8-DHF “rescued” a deficit in extinction of conditioned fear found in animals with a prior history of a single traumatic stress exposure.
Patients with PTSD and other anxiety disorders are thought to have deficits in extinction of aversive memories (22, 23). Similarly, rodents with anxiety-like behavior or trauma exposure demonstrate a deficit in extinction of conditioned fear (24–26). Notably, the finding that a single stressor is sufficient to impair later extinction is still quite novel and may have only one precedent in the literature (27). This disruption of extinction is thought to be due to both abnormalities in HPA-axis function and possible alterations in BDNF signaling. In mice, 2-hour immobilization led to a decrease in hippocampus BDNF protein levels at 5 and 10 hours after stress, but levels had returned to control levels when evaluated at 24 hours (28). In rats, a single 2-hour period of stress immobilization has been found to be more stressful than high-intensity footshocks (20). Similar stress has been shown to decrease BDNF mRNA in the hippocampus (29), and immobilization has been shown to decrease BDNF mRNA levels in the amygdala and cortex (30). In both cases, no difference was found in the TrkB receptor levels in any area. Together, these data suggest that even individual episodes of significant traumatic stress can alter BDNF function, which may affect later extinction processing.
Our data suggest that 7,8-DHF can fully “rescue” the deficit in extinction produced by prior immobilization stress. In fact, we found that 7,8-DHF exerts similar effects in both control and immobilization-stressed groups (Figure 4E). This finding suggests that the TrkB receptor pathways may be intact after immobilization stress. Thus, the extinction deficit observed in previously immobilization-stressed mice may be due to defective release of BDNF or to a defect in factors other than the BDNF-TrkB pathway, but which can be rescued with additional activation of TrkB.
We found no effects on baseline behaviors or pain sensitivity following acute 7,8-DHF. These data suggest that 7,8-DHF did not have indirect effects that could alter the experience of fear training. Notably we found augmented learning for both fear and extinction, further supporting the role of the TrkB agonist in potentiating learning, and it was not due to enhancing or reducing sensory/motor function in a certain direction. Also, all of the learning paradigms performed here followed a single acute administration of systemic 7,8-DHF, which suggests that the emotional learning and memory enhancements were a function of direct and rapid activation of brain TrkB, as previously shown in vitro and in vivo (16) and demonstrated for the first time here with autoradiography. Our data do not yet indicate where 7,8-DHF is acting in our fear acquisition and extinction studies. However, this study's immunoblots, autoradiography studies, and prior data from our group all suggest that TrkB in the amygdala, and possibly in the hippocampus and prefrontal cortex, could be involved in the systemic 7,8-DHF effects. Therefore, 7,8-DHF is likely augmenting emotional learning and plasticity, in the presence of endogenous BDNF, for both fear learning and extinction.
Overall, our findings suggest that this TrkB agonist may be an excellent research tool for understanding the effects of TrkB activation in a variety of learning and memory paradigms. Notably, the BDNF val/met polymorphism has been associated with increased anxiety-like behavior in humans (31, 32) and mice (33), as well as with altered extinction in both species (34). Recently this mouse model was shown to have an extinction deficit that was reversed with d-cycloserine (35), an NMDA [N-methyl-d-aspartic acid]-dependent cognitive enhancer (22). It will be fascinating to examine whether 7,8-DHF or similar TrkB-specific small-molecule agonists are able to reverse these effects in animal models and humans with the BDNF val/met polymorphism. Recent data also suggest that BDNF infusion into infralimbic cortex enhances extinction of fear (36), and there is a burgeoning literature suggesting that decreased BDNF signaling may be a critical component in the pathophysiology of mood disorders (15). Future research on the examination of systemically available BDNF agonists may lead to important, clinically relevant findings for the treatment of depression and anxiety disorders. Moreover, 7,8-DHF may be an attractive agent for improving extinction and other emotional learning deficits associated with psychopathology in humans.
1. : Hippocampal long-term potentiation is impaired in mice lacking brain-derived neurotrophic factor. Proc Natl Acad Sci USA 1995; 92:8856–8860Crossref, Medline, Google Scholar
2. : Brain-derived neurotrophic factor induces long-term potentiation in intact adult hippocampus: requirement for ERK activation coupled to CREB and upregulation of Arc synthesis. J Neurosci 2002; 22:1532–1540Crossref, Medline, Google Scholar
3. : Long-lasting neurotrophin-induced enhancement of synaptic transmission in the adult hippocampus. Science 1995; 267:1658–1662Crossref, Medline, Google Scholar
4. : Brain-derived neurotrophic factor triggers transcription-dependent, late phase long-term potentiation in vivo. J Neurosci 2002; 22:7453–7461Crossref, Medline, Google Scholar
5. : Rapid and selective induction of BDNF expression in the hippocampus during contextual learning. Nat Neurosci 2000; 3:533–535Crossref, Medline, Google Scholar
6. : BDNF upregulation during declarative memory formation in monkey inferior tem-poral cortex. Nat Neurosci 2000; 3:1134–1142Crossref, Medline, Google Scholar
7. : Deprivation of endogenous brain-derived neurotrophic factor results in impairment of spatial learning and memory in adult rats. Brain Res 1999; 835:259–265Crossref, Medline, Google Scholar
8. : Learning deficits in forebrain-restricted brain-derived neurotrophic factor mutant mice. Neuro-science 2003; 121:341–354Google Scholar
9. : Hippocampus-specific deletion of BDNF in adult mice impairs spatial memory and extinction of aversive memories. Mol Psychiatry 2007; 12:656–670Crossref, Medline, Google Scholar
10. : Regulation of amygdala-dependent learning by brain-derived neurotrophic factor is mediated by extracellular signal-regulated kinase and phosphatidylinositol-3-kinase. Neuropsychopharmacology 2006; 31:287–296Crossref, Medline, Google Scholar
11. : Brain-derived neurotrophic factor and tyrosine kinase receptor B involvement in amygdala-dependent fear conditioning. J Neurosci 2004; 24:4796–4806Crossref, Medline, Google Scholar
12. : Amygdala BDNF signaling is required for consolidation but not encoding of extinction. Nat Neurosci 2006; 9:870–872Crossref, Medline, Google Scholar
13. : The BDNF Val66Met polymorphism affects amygdala activity in response to emotional stimuli: evidence from a genetic imaging study. Neuroimage 2008; 42:1554–1559Crossref, Medline, Google Scholar
14. : New insights into BDNF function in depression and anxiety. Nat Neurosci 2007; 10:1089–1093Crossref, Medline, Google Scholar
15. : A neurotrophic model for stress-related mood disorders. Biol Psychiatry 2006; 59:1116–1127Crossref, Medline, Google Scholar
16. : A selective TrkB agonist with potent neurotrophic activities by 7,8-dihydroxyflavone. Proc Natl Acad Sci USA 2010; 107:2687–2692Crossref, Medline, Google Scholar
17. : Prelimbic cortical BDNF is required for memory of learned fear but not ex-tinction or innate fear. Proc Natl Acad Sci USA 2010; 107:2675–2680Crossref, Medline, Google Scholar
18. : High affinity GABA receptors: autoradiographic localization. Brain Res 1981; 222:285–307Crossref, Medline, Google Scholar
19. : Beta-catenin is required for memory consolidation. Nat Neurosci 2008; 11:1319–1326Crossref, Medline, Google Scholar
20. : Post-stress recovery of pituitary-adrenal hormones and glucose, but not the response during exposure to the stressor, is a marker of stress intensity in highly stressful situations. Brain Res 2002; 926:181–185Crossref, Medline, Google Scholar
21. : A single exposure to immobilization causes long-lasting pituitary-adrenal and behavioral sensitization to mild stressors. Horm Behav 2008; 54:654–661Crossref, Medline, Google Scholar
22. : Cognitive enhancers as adjuncts to psycho-therapy: use of d-cycloserine in phobic individuals to facilitate extinction of fear. Arch Gen Psychiatry 2004; 61:1136–1144Crossref, Medline, Google Scholar
23. : Failure of extinction of fear responses in posttraumatic stress disorder: evidence from second-order conditioning. Am J Psy-chiatry 2007; 164:1684–1692Link, Google Scholar
24. : Impaired extinction of learned fear in rats se-lectively bred for high anxiety: evidence of altered neuronal processing in prefrontal-amygdala pathways. Eur J Neurosci 2008; 28:2299–2309Crossref, Medline, Google Scholar
25. : Effects of single prolonged stress and d-cycloserine on contextual fear extinction and hippocampal NMDA receptor expression in a rat model of PTSD. Neuropsychopharmacology 2008; 33:2108–2116Crossref, Medline, Google Scholar
26. : Stress-induced prefrontal reorganization and executive dysfunction in rodents. Neurosci Biobehav Rev 2009; 33:773–783Crossref, Medline, Google Scholar
27. : Brief uncontrollable stress causes dendritic retraction in infralimbic cortex and resistance to fear extinc-tion in mice. J Neurosci 2006; 26:5733–5738Crossref, Medline, Google Scholar
28. : Voluntary exercise protects against stress-induced decreases in brain-derived neurotrophic factor protein expression. Neuroscience 2004; 124:985–992Crossref, Medline, Google Scholar
29. : Stress and glucocorticoids affect the expression of brain-derived neurotrophic factor and neuro-trophin-3 mRNAs in the hippocampus. J Neurosci 1995; 15:1768–1777Crossref, Medline, Google Scholar
30. : Stress increases brain-derived neurotropic factor messenger ribonucleic acid in the hypothala-mus and pituitary. Endocrinology 1995; 136:3743–3750Crossref, Medline, Google Scholar
31. : Association of a functional BDNF polymorphism and anxi-ety-related personality traits. Psychopharmacology (Berl) 2005; 180:95–99Crossref, Medline, Google Scholar
32. : BDNF Val66Met polymorphism is associated with HPA axis reactivity to psychological stress characterized by genotype and gender interactions. Psychoneuroendocrinology 2009; 34:382–388Crossref, Medline, Google Scholar
33. : Genetic variant BDNF (Val66Met) polymorphism alters anxiety-related behavior. Science 2006; 314:140–143Crossref, Medline, Google Scholar
34. : A genetic variant BDNF polymorphism alters extinction learning in both mouse and human. Science 2010; 327:863–866Crossref, Medline, Google Scholar
35. : Variant BDNF Val66Met polymorphism affects extinction of conditioned aversive memory. J Neurosci 2009; 29:4056–4064Crossref, Medline, Google Scholar
36. : Induction of fear extinction with hippocampal-infralimbic BDNF. Science 2010; 328:1288–1290Crossref, Medline, Google Scholar