An In Vivo MRI Study of Prefrontal Cortical Complexity in First-Episode Psychosis
Abstract
OBJECTIVE: The purpose of this study was to investigate abnormalities in the surface complexity of the prefrontal cortex and in the hemispheric asymmetry of cortical complexity in first-episode patients with schizophrenia. METHOD: An estimate of the surface complexity of the prefrontal cortex was derived from the number of voxels along the boundary between gray matter and CSF. Magnetic resonance imaging scans were acquired from patients with a first episode of schizophrenia (N=17), patients with a first episode of affective psychosis (N=17), and normal comparison subjects (N=17), age-matched within a narrow age range (18–29 years). This study group was the focus of a previous study that showed lower prefrontal cortical volume in patients with schizophrenia. RESULTS: Prefrontal cortical complexity was not significantly different among the groups. However, the schizophrenia patients differed significantly from the normal comparison subjects in asymmetry, with the schizophrenia patients showing less left-greater-than-right asymmetry in cortical complexity than the comparison subjects. CONCLUSIONS: An abnormal pattern of asymmetry in the prefrontal cortex of first-episode patients with schizophrenia provides evidence for a neurodevelopmental mechanism in the etiology of schizophrenia.
Evidence suggests prefrontal cortical abnormalities in schizophrenia. More specifically, findings from postmortem examinations (1, 2) and structural (for reviews, see references 3–5) and functional (for review, see reference 6) neuroimaging studies suggest the presence of subtle structural abnormalities. Most magnetic resonance imaging (MRI) studies have focused on volume, with some studies showing lower prefrontal volume (7–9) and others showing no difference between schizophrenia patients and comparison subjects (10). Additional cortical abnormalities include greater sulcal width (11) and gyral pattern abnormalities (12).
The cortical surface area (i.e., the number of voxels along the boundary between the gray matter and CSF) is influenced by cortical folding. It is therefore an estimate of cortical surface complexity, rather than just volume, that may provide an alternative approach for quantifying cortical abnormalities in schizophrenia. Cortical complexity has previously also been estimated by using the gyrification index, which is the ratio of the length of the inner surface of the brain to the length of the outer surface. The gyrification index is considered a quantitative assessment of gyrification (13) and provides a measure of overall cortical folding. A postmortem study of the gyrification index in schizophrenia showed right-sided hypergyria in the prefrontal region in male patients (14), a finding that was subsequently replicated in vivo with MRI (15). In contrast, an MRI study of male schizophrenic patients showed lower than normal gyrification in the whole left hemisphere (16).
In an independent MRI study of cortical complexity, defined as the frequency of sulcal-gyral convolutions in all three planes in each subregion of the cortical surface model, Narr and colleagues found abnormal asymmetry of gyral complexity in schizophrenia (17).
The purpose of this study was to test the hypothesis that abnormalities of cortical complexity, estimated as the number of voxels along the boundary between gray matter and CSF in the prefrontal cortex, are present in the prefrontal cortex in first-episode schizophrenia. Our measure of complexity, which also provides a rough estimate of surface area, may provide information in support of disturbances in development in schizophrenia.
Method
Subjects
The study consisted of 17 first-episode patients with schizophrenia and 17 first-episode patients with affective psychosis, including 13 with bipolar (manic) disorder and four with major depressive disorder. The patients were diagnosed according to DSM-IV criteria by using the Structured Clinical Interview for DSM-IV Axis I Disorders (SCID) (18). Seventeen psychiatrically well comparison subjects were recruited through newspaper advertisements, and the nonpatient (19) and personality disorders (20) versions of the SCID were administered to rule out psychopathology. Each subject group included two women. The subjects were matched on age and parental socioeconomic status, and they met the following criteria: age 18–29 years, IQ above 75, right-handedness, negative history of seizures, negative history of head trauma with loss of consciousness or neurological disorder, and no lifetime history of alcohol or other drug dependence. The date of the first psychiatric hospitalization was used as a reliable marker of the time of psychosis onset. Further details of the subjects are documented elsewhere, as the subjects were part of a previous study evaluating prefrontal volume (7) and a study evaluating prefrontal cortical thickness (21).
After a complete description of the study, written informed consent was obtained from each subject. The subjects were paid for participating.
MRI Methods
A 1.5-T General Electric scanner (General Electric Medical Systems, Milwaukee) was used to perform two MRI protocols. The first was a coronal series of contiguous spoiled gradient (SPGR) images, reformatted in the coronal plane and analyzed as 124 coronal 1.5-mm-thick slices. The second was an axial series of contiguous double-echo images (proton density and T2-weighted) to evaluate whole brain volume; details have been published previously (22).
An iterative expectation-maximization algorithm was used that estimated image intensity inhomogeneities, applied intensity corrections based on these estimates, and then classified tissue on the basis of the same set of signal intensity parameters for all subjects (23). To reduce noise before scan processing, an anisotropic diffusion filter (kappa=14 for SPGR and kappa=90 for proton/T2 images, iterations=3) was applied (24, 25). Finally, the results of the segmentation were superimposed on the coronal SPGR image and edited on computer workstations by using locally developed software algorithms to assign left and right prefrontal gray and white matter to separate tissue classes.
Prefrontal Segmentation
The prefrontal gray matter segmentations were manually edited in a previous study (7) by a single rater (Y.H.) blind to diagnostic groups, and they were edited further to define more fully cortical sulci before the measurement of cortical complexity. Briefly, prefrontal gray matter, measured anteriorly from the first slice containing brain tissue, extended posteriorly to the slice that was three slices anterior to the most anterior slice containing the temporal stem (the white matter tract connecting the temporal and frontal lobes).
Measurement of Prefrontal Cortical Complexity
An algorithm that measures the area of the boundary between prefrontal cortical gray matter and CSF in voxels (i.e., 0.9375× 0.9375×1.5 mm3) was applied to the edited segmentations (25–28). The gray matter/CSF voxel count refers to the number of voxels on the outer boundary of the prefrontal cortex, or the interface between cortical gray matter and CSF, and is an estimate of cortical surface complexity as well as cortical surface area (Figure 1).
Statistics
Previous evaluation of demographic and clinical measures (7) showed no difference among groups in age, parental socioeconomic status, three subscales of the Wechsler Adult Intelligence Scale—Revised, or clinical measures (for the two patient groups) including the Mini-Mental State Examination score, Global Assessment Scale score, total Brief Psychiatric Rating Scale score, age at first medication, medication dose (in chlorpromazine equivalents), and duration of illness. The first-episode patients with schizophrenia showed significantly lower socioeconomic status than the comparison subjects (F=6.97, df=2, 48, p=0.002).
A mixed model repeated-measures analysis of variance (ANOVA), with group (schizophrenia, affective psychosis, comparison) as the between-subjects factor and hemisphere (left, right) as the within-subjects factor, was used to test for differences in prefrontal cortical complexity between groups. Follow-up analyses included one-way ANOVA and post hoc Tukey’s honestly significant difference tests for complexity with the left and right hemispheres compared separately. In addition, asymmetry coefficients for the complexity measurements were obtained by using the formula (R–L)/0.5(R+L), where R and L were the complexity measurements in the right and left hemispheres, respectively. Correlations between prefrontal cortical complexity and demographic data were performed separately for each group. For both patient groups, exploratory correlations were also performed between prefrontal cortical complexity and clinical symptom measures. We also performed statistical analyses on our complexity measurements with correction for head size. Relative complexity was calculated by using the formula (ROI complexity/X)×100, where ROI was the region of interest and X was the volume of the intracranial contents or prefrontal gray matter.
Results
There was no main effect for group for prefrontal cortical complexity (F=0.81, df=2, 48, p=0.45), although complexity was greater in the left hemisphere for all groups (F=11.21, df=1, 48, p=0.002). Of particular note, the group-by-hemisphere interaction was also significant (F=3.30, df=2, 48, p=0.05), driven by less asymmetry in the patients with schizophrenia. More specifically, mean surface complexity, in voxels, was 12,206 (SD=1,260) on the right and 12,233 (SD=1,173) on the left in the schizophrenic patients, 12,565 (SD=1,495) on the right and 12,857 (SD=1,414) on the left in the affective psychosis patients, and 11,913 (SD=1,210) on the right and 12,517 (SD=1,476) on the left in the comparison subjects (Figure 2). The results of one-way ANOVAs for prefrontal left and right hemisphere cortical complexity were also not significant (left: F=0.90, df=2, 48, p=0.42; right: F=1.03, df=2, 48, p=0.37).
In order to understand further our group-by-hemisphere interaction, we additionally analyzed asymmetry coefficients for all groups (Figure 2). One-way ANOVA revealed a significant difference among the three groups in the asymmetry coefficient for prefrontal cortical complexity (F=3.24, df=2, 48, p=0.05). Post hoc analysis revealed that the first-episode patients with schizophrenia had a significantly smaller asymmetry coefficient for prefrontal cortical complexity than the normal comparison subjects (p=0.04, Tukey’s honestly significant difference test), indicating that the schizophrenia subjects had less left-greater-than-right hemispheric asymmetry in prefrontal cortical complexity than the comparison subjects. This difference indicates that the difference in complexity between the left and right cortical surfaces is smaller in patients with schizophrenia than in normal comparison subjects. We did not find a significant difference in asymmetry coefficients between the patients with affective psychosis and either the comparison subjects or the schizophrenic patients (Figure 2).
No significant correlations were found between cortical complexity and demographic and clinical measures for any group.
Discussion
In this study, we found a group-by-hemisphere interaction for prefrontal cortical complexity. In order to understand this interaction further, we analyzed the asymmetry coefficients for all groups. We found that the asymmetry coefficients for surface complexity significantly differed among the groups; the comparison subjects showed the normal pattern of left-greater-than-right asymmetry, and the first-episode patients with schizophrenia lacked such normal asymmetry. Our finding of less hemispheric asymmetry in prefrontal cortical complexity in first-episode schizophrenia is consistent with a growing body of literature that suggests that a lack of normal brain asymmetry, in terms of structure as well as function, may be part of the neuropathology of schizophrenia. Our results, however, do not resolve the issue of whether our asymmetry findings are specific to first-episode schizophrenia, as we did not show significant differences in cortical complexity between first-episode patients with affective psychosis and either first-episode patients with schizophrenia or normal comparison subjects. More research, with larger study groups, will be useful in clarifying this issue further.
Previous studies have indicated diminished or absent cerebral asymmetry in schizophrenia. The abnormalities in hemispheric asymmetry in schizophrenia include 1) a lack of normal asymmetry in prefrontal and occipitoparietal volumes (29); 2) less than normal asymmetry in the temporal lobes (30), the planum temporale cortex (31), the lateral (sylvian) fissure (32, 33), and the frontal and occipital lobes (34, 35); and 3) a reversal of normal asymmetry in the volumes of the occipital (36, 37) and frontal (36) lobes, posterior superior temporal gyrus (38), and inferior parietal lobule (39) and in planum temporal surface area (40, 41).
Moreover, in addition to findings of abnormal gyrification complexity in schizophrenia (14–16), there have also been reports of abnormal temporal lobe sulcal-gyral patterns in schizophrenia (12). Using cortical complexity measures, Narr and colleagues (17) found a disturbance in the patterns of gyral asymmetries in the frontal regions in schizophrenia. It is important to note, however, that other studies have failed to find evidence of abnormal asymmetry in schizophrenia (42, 43).
Cortical asymmetries become apparent during fetal development (44). Therefore, the absence or reversal of normal hemispheric asymmetries in schizophrenia suggests a disruption of normal neuronal development that results in abnormal cerebral lateralization. Our finding of less hemispheric asymmetry of prefrontal cortical complexity is consistent with this hypothesis of abnormal neurodevelopment in schizophrenia.
Received Oct. 7, 2003; revision received March 11, 2004; accepted March 22, 2004. From the Clinical Neuroscience Division, Laboratory of Neuroscience, VA Boston Healthcare System—Brockton Division, and Department of Psychiatry, Harvard Medical School; the Surgical Planning Laboratory, MRI Division, Department of Radiology, Brigham and Women’s Hospital and Harvard Medical School, Boston; the Psychotic Disorders Unit and the Psychiatric Neuroimaging Research Group, Department of Psychiatry, Massachusetts General Hospital, Boston; the Department of Psychiatry, Kyorin University School of Medicine, Tokyo; the Cognitive Neuroscience Laboratory, McLean Hospital, Belmont, Mass.; and the Brigham Behavioral Neurology Group, Brigham and Women’s Hospital, Boston. Address correspondence and reprint requests to Dr. Shenton or Dr. McCarley, Department of Psychiatry-116A, VA Boston Healthcare System—Brockton Division, Harvard Medical School, 940 Belmont St., Brockton, MA 02301; [email protected] or robert_mccarley@ hms.harvard.edu (e-mail). Supported by the Stanley Medical Research Institute (Ms. Wiegand); the Whitaker Foundation (Dr. Warfield); an award from the Center for Integration of Medicine and Innovative Technology (Dr. Warfield); grants K02 MH-01110 (Dr. Shenton), R01 MH-50740 (Dr. Shenton), and R01 MH-40799 (Dr. McCarley) from NIMH; grants P41 RR-13218 (Drs. Kikinis and Warfield) and R01 RR-11747 (Drs. Kikinis and Warfield) from the NIH National Center for Research Resources; grants P01 CA-67165 (Dr. Warfield) and R01 CA-86879 (Dr. Warfield) from the National Cancer Institute; and VA Merit Awards (Drs. Shenton and McCarley).
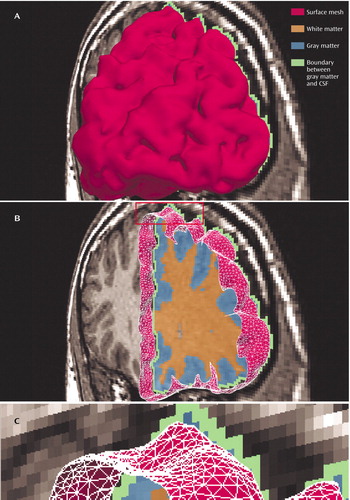
Figure 1. Graphical Visualization of the Prefrontal Cortexa
aThe top panel, A, is a model of the prefrontal cortex in the anterior-posterior viewing direction. The middle panel, B, is a model of the prefrontal cortex cut to show the different tissue types associated with a coronal slice of the magnetic resonance (MR) volume. The area identified in the rectangle at the top of panel B is shown as a close-up in the bottom panel, C. Here, the surface mesh (red) and the other tissue types (identified as orange, blue, and green) can be better appreciated. A measure of cortical surface complexity, which also serves as an estimate of cortical surface area, was obtained by counting the total number of green voxels in the MR volume.
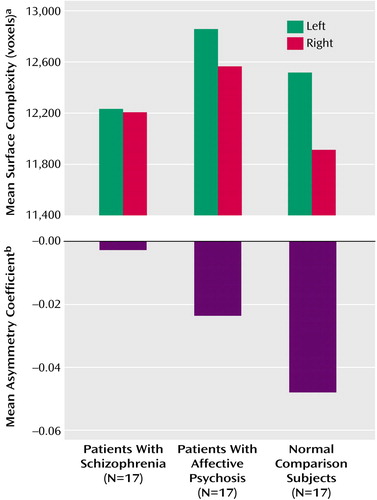
Figure 2. Surface Complexity of the Prefrontal Cortex in the Left and Right Hemispheres and Asymmetry of Cortical Surface Complexity in First-Episode Patients With Schizophrenia, First-Episode Patients With Affective Psychosis, and Normal Comparison Subjects
aSurface complexity was calculated by summing the number of voxels along the boundary between the gray matter and CSF in the prefrontal cortex (i.e., the total number of green voxels shown in Figure 1).
bA larger asymmetry coefficient corresponds to greater asymmetry, and a negative asymmetry coefficient indicates greater complexity in the left hemisphere than in the right hemisphere.
1. Rajkowska G, Selemon LD, Goldman-Rakic PS: Neuronal and glial somal size in the prefrontal cortex: a postmortem morphometric study of schizophrenia and Huntington disease. Arch Gen Psychiatry 1998; 55:215–224Crossref, Medline, Google Scholar
2. Selemon LD, Rajkowska G, Goldman-Rakic PS: Elevated neuronal density in prefrontal area 46 in brains from schizophrenic patients: application of a three-dimensional, stereologic counting method. J Comp Neurol 1998; 392:402–412Crossref, Medline, Google Scholar
3. McCarley RW, Wible CG, Frumin M, Hirayasu Y, Levitt JJ, Fischer IA, Shenton ME: MRI anatomy of schizophrenia. Biol Psychiatry 1999; 45:1099–1119Crossref, Medline, Google Scholar
4. Shenton ME, Frumin M, McCarley RW, Maier SE, Westin C-F, Fischer IA, Dickey C, Kikinis R: Morphometric magnetic resonance imaging studies: findings in schizophrenia, in Psychiatric Neuroimaging Research Strategies. Edited by Dougherty DD, Rauch SL. Washington, DC, American Psychiatric Press, 2001, pp 1–60Google Scholar
5. Shenton ME, Wible CG, McCarley RW: A review of magnetic resonance imaging studies of brain abnormalities in schizophrenia, in Brain Imaging in Clinical Psychiatry. Edited by Krishnan KRR, Doraiswamy PM. New York, Marcel Dekker, 1997, pp 297–380Google Scholar
6. Manoach DS: Prefrontal cortex dysfunction during working memory performance in schizophrenia: reconciling discrepant findings. Schizophr Res 2003; 60:285–298Crossref, Medline, Google Scholar
7. Hirayasu Y, Tanaka S, Shenton ME, Salisbury DF, DeSantis MA, Levitt JJ, Wible C, Yurgelun-Todd D, Kikinis R, Jolesz FA, McCarley RW: Prefrontal gray matter volume reduction in first episode schizophrenia. Cereb Cortex 2001; 11:374–381Crossref, Medline, Google Scholar
8. Gur RE, Cowell P, Turetsky BI, Gallacher F, Cannon T, Bilker WB, Gur RC: A follow-up magnetic resonance imaging study of schizophrenia: relationship of neuroanatomical changes to clinical and neurobehavioral measures. Arch Gen Psychiatry 1998; 55:145–152Crossref, Medline, Google Scholar
9. Nopoulos P, Torres I, Flaum M, Andreasen NC, Ehrhardt JC, Yuh WTC: Brain morphology in first-episode schizophrenia. Am J Psychiatry 1995; 152:1721–1723Link, Google Scholar
10. Baare WF, Hulshoff Pol HE, Hijman R, Mali WP, Viergever MA, Kahn RS: Volumetric analysis of frontal lobe regions in schizophrenia: relation to cognitive function and symptomatology. Biol Psychiatry 1999; 45:1597–1605Crossref, Medline, Google Scholar
11. Weinberger DR, Torrey EF, Neophytides AN, Wyatt RJ: Structural abnormalities in the cerebral cortex of chronic schizophrenic patients. Arch Gen Psychiatry 1979; 36:935–939Crossref, Medline, Google Scholar
12. Kikinis R, Shenton ME, Gerig G, Hokama H, Haimson J, O’Donnell BF, Wible CG, McCarley RW, Jolesz FA: Temporal lobe sulco-gyral pattern anomalies in schizophrenia: an in vivo MR three-dimensional surface rendering study. Neurosci Lett 1994; 182:7–12Crossref, Medline, Google Scholar
13. Zilles K, Armstrong E, Schleicher A, Kretschmann HJ: The human pattern of gyrification in the cerebral cortex. Anat Embryol (Berl) 1988; 179:173–179Crossref, Medline, Google Scholar
14. Vogeley K, Schneider-Axmann T, Pfeiffer U, Tepest R, Bayer TA, Bogerts B, Honer WG, Falkai P: Disturbed gyrification of the prefrontal region in male schizophrenic patients: a morphometric postmortem study. Am J Psychiatry 2000; 157:34–39Link, Google Scholar
15. Vogeley K, Tepest R, Pfeiffer U, Schneider-Axmann T, Maier W, Honer WG, Falkai P: Right frontal hypergyria differentiation in affected and unaffected siblings from families multiply affected with schizophrenia: a morphometric MRI study. Am J Psychiatry 2001; 158:494–496Link, Google Scholar
16. Kulynych JJ, Luevano LF, Jones DW, Weinberger DR: Cortical abnormality in schizophrenia: an in vivo application of the gyrification index. Biol Psychiatry 1997; 41:995–999Crossref, Medline, Google Scholar
17. Narr KL, Thompson PM, Sharma T, Moussai J, Zoumalan C, Rayman J, Toga AW: Three-dimensional mapping of gyral shape and cortical surface asymmetries in schizophrenia: gender effects. Am J Psychiatry 2001; 158:244–255Link, Google Scholar
18. First MB, Spitzer RL, Gibbon M, Williams JBW: Structured Clinical Interview for DSM-IV Axis I Disorders (SCID). Washington, DC, American Psychiatric Press, 1995Google Scholar
19. First M, Spitzer R, Williams J, Gibbon M: Structured Clinical Interview for DSM-IV—Non-Patient Edition (SCID-NP, Version 1.0). Washington, DC, American Psychiatric Press, 1995Google Scholar
20. First MB, Gibbon M, Spitzer RL, Williams JBW, Benjamin L: Structured Clinical Interview for DSM-IV Axis II Personality Disorders (SCID-II): User’s Guide. Washington, DC, American Psychiatric Press, 1997Google Scholar
21. Wiegand LC, Warfield SK, Levitt JJ, Hirayasu Y, Salisbury DF, Heckers S, Dickey CC, Kikinis R, Jolesz FA, McCarley RW, Shenton ME: Prefrontal cortical thickness in first-episode psychosis: a magnetic resonance imaging study. Biol Psychiatry 2004; 55:131–140Crossref, Medline, Google Scholar
22. Dickey CC, Shenton ME, Hirayasu Y, Fischer I, Voglmaier MM, Niznikiewicz MA, Seidman LJ, Fraone S, McCarley RW: Large CSF volume not attributable to ventricular volume in schizotypal personality disorder. Am J Psychiatry 2000; 157:48–54Link, Google Scholar
23. Wells W, Kikinis R, Grimson W, Jolesz FA: Adaptive segmentation of MRI data. IEEE Trans Med Imaging 1996; 15:429–442Crossref, Medline, Google Scholar
24. Gerig G, Kubler O, Kikinis R, Jolesz FA: Nonlinear anisotropic filtering of MRI data. IEEE Trans Med Imaging 1992; 2:221–232Crossref, Google Scholar
25. Kikinis R, Jolesz FA, Gerig G, Sandor T, Cline HE, Lorensen WE, Halle M, Benton SA:3D morphometric and morphologic information derived from clinical brain MR images, in 3D Imaging in Medicine. Edited by Hohne KH, Fuchs H, Pizer SM. Berlin, Springer-Verlag, 1990, pp 441–454Google Scholar
26. Jaume S, Ferrant M, Macq B, Hoyte LX, Fielding JR, Schreyer A, Kikinis R, Warfield SK: Tumor detection in the bladder wall with a measurement of abnormal thickness in CT scans. IEEE Trans Biomed Eng 2003; 50:383–390Crossref, Medline, Google Scholar
27. Schreyer AG, Fielding JR, Warfield SK, Lee JH, Loughlin KR, Dumanli H, Jolesz FA, Kikinis R: Virtual CT cystoscopy: color mapping of bladder wall thickness. Invest Radiol 2000; 35:331–334Crossref, Medline, Google Scholar
28. Warfield SK, Kaus M, Jolesz FA, Kikinis R: Adaptive, template moderated, spatially varying statistical classification. Med Image Anal 2000; 4:43–55Crossref, Medline, Google Scholar
29. Sharma T, Lancaster E, Sigmundsson T, Lewis S, Takei N, Gurling H, Barta P, Pearlson G, Murray R: Lack of normal pattern of cerebral asymmetry in familial schizophrenic patients and their relatives—the Maudsley Family Study. Schizophr Res 1999; 40:111–120Crossref, Medline, Google Scholar
30. DeLisi LE, Hoff AL, Neale C, Kushner M: Asymmetries in the superior temporal lobe in male and female first-episode schizophrenic patients: measures of the planum temporale and superior temporal gyrus by MRI. Schizophr Res 1994; 12:19–28Crossref, Medline, Google Scholar
31. Falkai P, Bogerts B, Schneider T, Greve B, Pfeiffer U, Pilz K, Gonsiorzcyk C, Majtenyi C, Ovary I: Disturbed planum temporale asymmetry in schizophrenia: a quantitative post-mortem study. Schizophr Res 1995; 14:161–176Crossref, Medline, Google Scholar
32. Hoff AL, Riordan H, O’Donnell D, Stritzke P, Neale C, Boccio A, Anand AK, DeLisi LE: Anomalous lateral sulcus asymmetry and cognitive function in first-episode schizophrenia. Schizophr Bull 1992; 18:257–272Crossref, Medline, Google Scholar
33. Falkai P, Bogerts B, Greve B, Pfeiffer U, Machus B, Folsch-Reetz B, Majtenyi C, Ovary I: Loss of sylvian fissure asymmetry in schizophrenia: a quantitative post mortem study. Schizophr Res 1992; 7:23–32Crossref, Medline, Google Scholar
34. Falkai P, Schneider T, Greve B, Klieser E, Bogerts B: Reduced frontal and occipital lobe asymmetry on the CT-scans of schizophrenic patients: its specificity and clinical significance. J Neural Transm Gen Sect 1995; 99:63–77Crossref, Medline, Google Scholar
35. Bilder RM, Wu H, Bogerts B, Degreef G, Ashtari M, Alvir JM, Snyder PJ, Lieberman JA: Absence of regional hemispheric volume asymmetries in first-episode schizophrenia. Am J Psychiatry 1994; 151:1437–1447Link, Google Scholar
36. Luchins DJ, Weinberger DR, Wyatt RJ: Schizophrenia: evidence of a subgroup with reversed cerebral asymmetry. Arch Gen Psychiatry 1979; 36:1309–1311Crossref, Medline, Google Scholar
37. Tsai LY, Nasrallah HA, Jacoby CG: Hemispheric asymmetries on computed tomographic scans in schizophrenia and mania: a controlled study and a critical review. Arch Gen Psychiatry 1983; 40:1286–1289Crossref, Medline, Google Scholar
38. Pearlson GD, Barta PE, Powers RE, Menon RR, Richards SS, Aylward EH, Federman EB, Chase GA, Petty RG, Tien AY: Ziskind-Somerfeld Research Award 1996: medial and superior temporal gyral volumes and cerebral asymmetry in schizophrenia versus bipolar disorder. Biol Psychiatry 1997; 41:1–14Crossref, Medline, Google Scholar
39. Niznikiewicz M, Donnino R, McCarley RW, Nestor PG, Iosifescu DV, O’Donnell B, Levitt J, Shenton ME: Abnormal angular gyrus asymmetry in schizophrenia. Am J Psychiatry 2000; 157:428–437Link, Google Scholar
40. Barta PE, Pearlson GD, Brill LB II, Royall R, McGilchrist IK, Pulver AE, Powers RE, Casanova MF, Tien AY, Frangou S, Petty RG: Planum temporale asymmetry reversal in schizophrenia: replication and relationship to gray matter abnormalities. Am J Psychiatry 1997; 154:661–667Link, Google Scholar
41. Petty RG, Barta PE, Pearlson GD, McGilchrist IK, Lewis RW, Tien AY, Pulver A, Vaughn DD, Casanova MF, Powers RE: Reversal of asymmetry of the planum temporale in schizophrenia. Am J Psychiatry 1995; 152:715–721Link, Google Scholar
42. Kleinschmidt A, Falkai P, Huang Y, Schneider T, Furst G, Steinmetz H: In vivo morphometry of planum temporale asymmetry in first-episode schizophrenia. Schizophr Res 1994; 12:9–18Crossref, Medline, Google Scholar
43. Bartley AJ, Jones DW, Torrey EF, Zigun JR, Weinberger DR: Sylvian fissure asymmetries in monozygotic twins: a test of laterality in schizophrenia. Biol Psychiatry 1993; 34:853–863Crossref, Medline, Google Scholar
44. Chi JG, Dooling EC, Gilles FH: Gyral development of the human brain. Ann Neurol 1977; 1:86–93Crossref, Medline, Google Scholar