Comparative Effect of Atypical and Conventional Antipsychotic Drugs on Neurocognition in First-Episode Psychosis: A Randomized, Double-Blind Trial of Olanzapine Versus Low Doses of Haloperidol
Abstract
OBJECTIVE: The effect of antipsychotic medication on neurocognitive function remains controversial, especially since most previous work has compared the effects of novel antipsychotic medications with those of high doses of conventional medications. This study compares the neurocognitive effects of olanzapine and low doses of haloperidol in patients with first-episode psychosis. METHOD: Patients with a first episode of schizophrenia, schizoaffective disorder, or schizophreniform disorder (N=167) were randomly assigned to double-blind treatment with olanzapine (mean modal dose= 9.63 mg/day) or haloperidol (mean modal dose=4.60 mg/day) for the 12-week acute phase of a 2-year study. The patients were assessed with a battery of neurocognitive tests at baseline and 12 weeks after beginning treatment. RESULTS: An unweighted neurocognitive composite score, composed of measures of verbal fluency, motor functions, working memory, verbal memory, and vigilance, improved significantly with both haloperidol and olanzapine treatment (effect sizes of 0.20 and 0.36, respectively, no significant difference between groups). A weighted composite score developed from a principal-component analysis of the same measures improved to a significantly greater degree with olanzapine, compared with haloperidol. Anticholinergic use, extrapyramidal symptoms, and estimated IQ had little effect on the statistical differentiation of the medications, although duration of illness had a modest effect. The correlations of cognitive improvement with changes in clinical characteristics and with side effects of treatment were significant for patients who received haloperidol but not for patients who received olanzapine. CONCLUSIONS: Olanzapine has a beneficial effect on neurocognitive function in patients with a first episode of psychosis. However, in a comparison of the effects of olanzapine and low doses of haloperidol, the difference in benefit is small.
Neurocognition is severely impaired in schizophrenia. Measures of certain crucial functions, such as attention, memory, executive functions, and motor speed, in patients have been reported to reach two standard deviations below the mean for healthy comparison subjects (1). Meta-analytic techniques, which tend to underestimate the size of a statistical effect, have suggested that patients with schizophrenia perform between one and one and one-half standard deviations below the normal mean in numerous areas of neurocognition (2). Neurocognitive impairment is present at the first episode of psychosis, and patients with a first episode of psychosis may be as severely impaired in many cognitive domains as patients with chronic schizophrenia (3–5).
Neurocognitive impairment is associated with key features of schizophrenia, such as outcome and adaptive dysfunction (6, 7), including the inability to acquire skills, poor social problem solving, and poor community functioning (6, 8). Cognitive impairment may a stronger correlate of poor outcome than any other symptom domain (6). Thus, neurocognitive performance has come to be viewed as a crucial target for pharmacologic treatments for psychotic disorders.
Typical antipsychotics given at traditionally used doses provide little benefit in treating the cognitive disturbances of patients with schizophrenia (9). Atypical antipsychotic medications appear to improve cognitive performance in those patients (10, 11). Clozapine (12–14), risperidone (14–16), olanzapine (14, 16), and quetiapine (17) are associated with improved cognitive performance in patients with schizophrenia. A meta-analysis of 15 studies published as of July, 1998—all but two of which assessed the cognitive effects of clozapine or risperidone—suggested that significant improvement in cognition was found with the atypical antipsychotics even when the results of each study were corrected for multiple comparisons (18). Data published after this meta-analysis was completed suggested that olanzapine may have cognition-enhancing properties that are at least as substantial as those reported for risperidone and clozapine (14, 16). However, recent considerations, such as the importance of the dose of typical antipsychotic comparators (11, 18), have called into question whether atypical antipsychotics actually improve cognition or whether they simply afford a release from the deleterious effects, such as extrapyramidal symptoms, of inappropriately large doses of typical antipsychotics and concomitant adjunctive agents such as anticholinergics (11, 18, 19). Lower doses of typical medications may not have as deleterious an effect on cognition as the traditional dose ranges for those medications (20–22).
This study is a randomized, double-blind trial of relatively low doses of haloperidol versus olanzapine in a large group of patients with first-episode psychosis. We tested the following hypotheses: 1) olanzapine will improve neurocognitive functions after a 12-week trial, and 2) cognitive improvements with olanzapine will exceed those found with low doses of haloperidol.
Method
Study Setting and Sponsorship
The project was conducted at 14 academic medical centers in North America and Western Europe. Funding was provided by Lilly Research Laboratories. The study was directed by a steering committee composed of the study principal investigator (J.A.L.), the sponsor’s lead investigators (M.T., G.D.T.), the principal study biostatistician (T.M.S.), and the 14 site principal investigators.
Patient Selection Criteria
Patients were included in the study if they met the following criteria: 1) were age 16–40 years; 2) had onset of psychotic symptoms before age 35 years; 3) met the DSM-IV criteria for schizophrenia, schizophreniform disorder, or schizoaffective disorder, as assessed with the Structured Clinical Interview for DSM-IV, Research Version; 4) experienced psychotic symptoms (delusions, hallucinations, thought disorder, and grossly bizarre behavior) for at least 1 month but not more than 60 months; 5) had a score ≥4 on at least two Positive and Negative Syndrome Scale (23) psychosis items (P1, P2, P3, P5, or P6) or a score ≥5 on one psychosis item; 6) had a Clinical Global Impression (CGI) severity of illness score ≥4 (moderately ill); 7) required treatment with antipsychotic drugs on a clinical basis; 8) had a level of understanding sufficient to communicate with the research staff and to cooperate with all tests and examinations required by the protocol; 9) understood the nature of the study and signed an informed consent document (or had an authorized legal representative who understood the nature of the study and signed an informed consent document); and, for female patients of childbearing potential, 10) were using a medically accepted means of contraception.
Patients were excluded from the study for any of the following reasons: 1) prior antipsychotic drug treatment of more than 16 cumulative weeks in the patient’s lifetime, treatment with clozapine at any time in the patient’s lifetime, or treatment with an injectable depot antipsychotic within less than three dosing intervals before study entry; 2) currently being pregnant or nursing; 3) a serious, unstable illness or findings from a medical examination that suggested a contraindication to antipsychotic drug treatment; 4) a history of allergic or severe adverse reactions to the study medications; 5) DSM-IV substance dependence (except caffeine and nicotine) within 1 month before visit 1; 6) having been judged clinically to be at serious suicide risk; 7) having required treatment with anticonvulsants, benzodiazepines (except for use that was allowed for amelioration of agitation and control of extrapyramidal symptoms), antidepressants, psychostimulants, or other antipsychotic drugs used concurrently with study medications and not permitted as concomitant treatments; 8) contraindications to neuroimaging (e.g., presence of metal prostheses) included in the local regulatory agency’s policies; 9) a history of any DSM-IV psychotic disorder with recovery (recovery, though based on clinical impression of the patient’s history, was defined as the cessation of symptoms [positive and negative] and return of functioning for 6 months or longer; for example, a patient who had a brief psychotic disorder, recovered [no longer had positive and negative symptoms], and then had another episode 6.5 months later would be considered to have history of psychotic disorder with recovery); 10) a premorbid IQ of ≤70; or 11) having received ECT within 1 month (30 days) before study entry.
Characteristics of Patients
Of the 263 patients randomly assigned to treatment, 244 completed the baseline neurocognitive assessments and 167 were able to complete the neurocognitive assessments at baseline and at the 12-week endpoint. (Table 1 summarizes the demographic and clinical characteristics of the treatment groups.) Completers performed significantly better than noncompleters on the WAIS-R (26) subtests (F=5.35, df=1, 241, p<0.03) and the Continuous Performance Test (28) (F=4.85, df=1, 218, p<0.03). However, these differences were not significant after correction for multiple comparisons.
Study Design and Procedures
Patients who met the inclusion criteria were assessed for competence to provide informed consent. If they were competent, the study was explained and they were asked to participate and provide informed consent. Patients who had been receiving antipsychotic medications underwent a washout period of 2–14 days and were randomly assigned to treatment with olanzapine or haloperidol under double-blind conditions. During the 12-week acute treatment phase of this 2-year study, the initial dose titration ranges in the first 6 weeks were 5–10 mg/day for olanzapine and 2–6 mg/day for haloperidol and in the second 6 weeks were 5–20 mg/day for olanzapine and 2–20 mg/day for haloperidol. The dose titration schedule and philosophy were to go slowly and target the lowest effective dose while still enabling patients to receive effective treatment. Medication doses could be adjusted as clinically indicated within the prescribed range after week 6 of treatment and for the remainder of the study. The mean modal dose, defined as the dose that each individual received most frequently, was 4.60 mg (SD=2.18) for haloperidol and 9.63 mg (SD=2.18) for olanzapine. The mean modal dose during the week of neurocognitive testing was 5.54 mg (SD=4.32) for haloperidol and 10.62 mg (SD=3.67) for olanzapine.
Specific concomitant medications were permitted for given clinical indications. During the washout and acute treatment phases, 500–2000 mg/day of chloral hydrate, 1–8 mg/day of lorazepam, or 5–40 mg/day of diazepam could be given for the management of agitation, general behavior disturbances, and/or insomnia, but only for a cumulative duration of ≤21 days. These medications were not permitted within 24 hours of neurocognitive testing.
Antiparkinsonian medications were not administered prophylactically. However, if clinically significant extrapyramidal symptoms occurred, anticholinergic medication (up to 6 mg/day of benztropine or biperiden, 10–80 mg/day of propranolol, or procyclidine [oral or intramuscular administration of 5–10 mg, 2–3 times daily, for a maximum dose of 30 mg/day]) was allowed to be given. Antidepressants and mood stabilizers were not permitted in the acute treatment phase of the study. Patients requiring these medications were withdrawn from the study.
Testing Conditions and Timing of Neurocognitive Assessments
Patients were scheduled to be tested at baseline, before starting treatment with the study drug. However, a 3-day window was allowed to accommodate patients who could not be tested before receiving the study medication. Follow-up testing to determine short-term treatment effects on neurocognition was completed 12 weeks after the initiation of the study medication. Forty percent (31 of 78) of the haloperidol-treated patients and 7% (six of 89) of the patients in the olanzapine arm received anticholinergic treatment during the week that follow-up testing was completed.
Neurocognitive Tests
The test battery was divided into three components: primary, secondary, and additional batteries (Table 2). Tests were always completed in the order they are listed in Table 3. As stated in the protocol a priori, scores on only the tests from the primary battery were hypothesized to improve differentially with olanzapine treatment. This decision was based on several factors. First, we followed the guidelines of Keefe et al. (18) and Harvey and Keefe (11) regarding the optimal characteristics of tests to be used in clinical trials in which cognitive change is measured. We judged the tests in the primary battery to adhere to these guidelines more closely than those in the secondary battery because the primary battery included tests with statistical properties that allow findings of significant improvement in most schizophrenia patients and because previous data suggested that these tests measure phenomena that may be responsive to antipsychotic treatment. A demonstrated relationship of the cognitive construct with functional outcome was also a consideration. Second, since the entire neurocognitive battery is very lengthy, we were concerned that some patients would have difficulty completing all the tests. While training the testers, we emphasized that if a patient was having difficulty completing the test battery, his/her efforts should be focused on completing the primary battery, a strategy that would avoid discontinuation of testing at variable points in the battery. Thus, as expected, the number of patients was slightly smaller for the tests in the secondary battery.
The secondary battery of tests included measures that assessed specific hypotheses deemed to be less important than the primary hypothesis of general cognitive improvement. For instance, the Stroop Color and Word Test, the variable-interval delayed alternation task, and the object alternation task were chosen to test the hypothesis that olanzapine may improve inhibition of inappropriate responses. The Benton Line Orientation Test was included to determine whether any cognitive benefit of treatment would extend to tests of posterior brain function.
An additional battery of tests, to be used as possible covariates in analyzing the primary and secondary batteries, was completed only at week 12.
Statistical Methods
Primary analyses
Based on the hypotheses detailed in our original protocol (i.e., that the cognitive functions measured by the tests in the primary battery would respond to treatment), we constructed a composite score that weighted each of the cognitive domains from the primary battery equally. The neurocognitive measures were standardized by using data from the entire study group, without respect to treatment group membership of patients who were tested at baseline and endpoint. The mean of each measure was set to 0 and the standard deviation was set to 1 to allow each score to contribute to the composite without respect to scaling. A patient’s scores were included in the composite score analyses only if data from the patient were available for five or more of the six cognitive domains. These domains and their measures were as follows: attention (Continuous Performance Test, Identical Pairs Version d′), verbal fluency (category instances score plus Controlled Oral Association Test score), verbal memory (California Verbal Learning Test total recall score), motor speed (mean of the Finger Oscillation Test scores for the dominant hand and the nondominant hand), working memory (0.5 [Letter-Number Sequencing Test total score] – 0.5 [Dot Test of Visuospatial Working Memory deficit]), and visuomotor speed (WAIS-R digit-symbol test score). The signs of the Dot Test scores were reversed to make them consistent with the direction of scoring for other tests, for which higher scores reflect better performance. We then used analysis of variance to test whether the groups differed on this unweighted composite score.
Secondary analyses
We also formed a weighted composite score based on the first factor from a principal-component analysis of the primary battery variables at baseline. We chose a single factor because the first factor accounted for most of the variance in the variables and we wanted to minimize type I statistical error. Data were included for all patients for whom full baseline and endpoint scores were available. The principal-component analysis produced a set of weights for constructing the weighted composite score for each subject at baseline. This set of weights was applied to the endpoint neurocognitive scores to determine treatment-related change.
For both composite scores, the analyses were repeated with selected covariates added to the model in a stepwise procedure to determine whether the covariates changed the basic relation between treatment and neurocognitive change. We also performed exploratory analyses of the effects of each medication on the neurocognitive variables and of the relation between neurocognitive treatment effects and other clinical changes. Statistical significance was set at p<0.05 and was not controlled for multiple comparisons.
Results
Table 3 shows the raw baseline and endpoint neurocognitive test scores of the two treatment groups.
Effect of Treatment on Unweighted Composite Scores
Effect sizes were determined by dividing the difference between the baseline mean and the endpoint mean by the baseline standard deviation. Olanzapine improved the unweighted composite score significantly (t=6.46, df=88, p<0.0001), with an effect size of 0.36. Haloperidol also had a significant effect on the unweighted composite score (t=2.66, df=77, p<0.01), with an effect size of 0.20. The differential effect of the two treatments accounted for 2.2% of the variance in neurocognitive change, but the result only approached significance (F=3.09, df=1, 141, p=0.08).
Variables that might be expected to affect cognition were added as covariates to the model sequentially, in the hypothesized order of importance (Table 4): extrapyramidal symptoms as reflected by Simpson-Angus Rating Scale (41) total scores, a dichotomous variable of anticholinergic medication ingestion during the week of testing, National Adult Reading Test scores as a proxy of premorbid IQ, and duration of illness. National Adult Reading Test scores and duration of illness were included because they differed significantly between groups at baseline. In sum, extrapyramidal symptoms, use of anticholinergic medication, and National Adult Reading Test score had minimal effects on the differential neurocognitive effect of the medications. Duration of illness reduced the effect of treatment on the unweighted composite score.
Principal-Component Analysis
The first eigenvalue was 2.98, and the sum of the eigenvalues was 6; thus, the first factor accounted for about 50% of the total matrix variance. The weights for the measures included in the first factor were all in the same direction and ranged from 0.35 to 0.46 (Table 5). Although the weights were close to equal, the weight for the verbal fluency sum (0.46) was approximately 30% higher than that for the Finger Oscillation Test (0.35), indicating that the verbal fluency measure contributed about 30% more information to the first factor, compared with the Finger Oscillation Test.
The weighted scores were subjected to the sequence of covariate analyses used for the unweighted composite scores. The treatments differed significantly in their effect on the weighted composite score (F=5.75, df=1, 112, p<0.02), with 4.9% of the variance in the weighted composite score explained by treatment. The effect size of this improvement was 0.36 standard deviations for olanzapine and 0.15 standard deviations for haloperidol. The effects of the covariates are summarized in Table 6.
Effects of Treatment on Specific Neurocognitive Measures
Figure 1 shows the effect size of treatment on the measures from the primary battery, calculated as a standardized z score for each measure.
Relation of Neurocognitive Change With Other Clinical Changes
In the haloperidol group only, change in the unweighted neurocognitive composite score was significantly correlated with changes in the CGI severity of illness score, the Simpson-Angus Rating Scale score, and the Positive and Negative Syndrome Scale total, negative, and general symptom scores (Table 7). (No significant correlations with scores on a measure of akathisia [42] were found.) In the olanzapine group, none of these correlations were significant. The pattern of correlations with the weighted composite score was very similar. Significant differences between the groups were found for the correlations of the change in the unweighted composite score with the change in the negative symptom score (F=5.54, df=1, 163, p=0.02) and in the extrapyramidal symptom score (F=4.72, df=1, 163, p=0.03) and for the correlations of the change in the weighted composite score with the change in the CGI severity of illness score (F=4.12, df=1, 130, p=0.04) and in the extrapyramidal symptom score (F=7.74, df=1, 130, p=0.006).
Discussion
The majority of published studies investigating the neurocognitive effects of antipsychotic treatment have had substantial methodological weaknesses (11, 18, 19). Many of these problems were addressed in this study, which included double-blind conditions, random assignment of subjects to the medication groups, low doses of the typical antipsychotic comparator, and a large number of subjects. The neurocognitive effect size for olanzapine treatment (0.36 for the unweighted and weighted composite scores) was in the range of the improvements reported in most previous studies of atypical antipsychotics (11), although not as large as the benefit reported for olanzapine by Purdon et al. (16). However, the size of this effect was small relative to the severity of deficits found in patients with first-episode psychosis and schizophrenia, which ranged between one and two standard deviations from the scores for healthy comparison subjects (2, 11). Thus, while olanzapine improves neurocognitive function in psychotic patients, the need for further improvement is substantial.
Whether neurocognitive improvement with atypical antipsychotics is secondary to other clinical changes, such as symptom improvement or release from the side effects of typical antipsychotics, is a matter of controversy (11, 18, 19). The absence of a significant correlation between neurocognitive change and symptom change in patients treated with olanzapine suggests that the cognitive benefit of olanzapine is not secondary to other clinical changes.
The statistical approach and design features of this study helped address the effects of dosing, side effects, and anticholinergic medication on neurocognition in patients who received haloperidol. One of the key features of this study design was the dosing strategy chosen for patients randomly assigned to receive haloperidol. Although previous studies of the effect of atypical antipsychotics suggested that risperidone, olanzapine, clozapine, and quetiapine improve neurocognitive performance in patients with schizophrenia, many of these studies have been criticized for the large doses of typical antipsychotic medication (usually haloperidol) used in the comparison condition (11, 18). Patients randomly assigned to the haloperidol arm of this study received a mean modal dose of 5.21 mg/day (260.5 mg/day of chlorpromazine equivalents). The mean dose of haloperidol that patients received the week of testing was slightly higher (5.54 mg/day, equivalent to 277.0 mg/day of chlorpromazine equivalents). Both of these doses are substantially lower than the mean modal dose of typical antipsychotic medication (736.4 mg/day of chlorpromazine equivalents) used as a comparator in previous studies investigating the effects of atypical antipsychotics on neurocognitive function (11). The significant neurocognitive improvement in patients who received haloperidol suggests that the relative neurocognitive efficacy of olanzapine and other atypical antipsychotics is partially attributable to the high doses of typical medication usually prescribed in these studies. Although methodological differences between this study and the study of low-dose haloperidol by Green et al. (20) limit direct comparison, the effect sizes of neurocognitive change with haloperidol (0.20 for the unweighted composite score and 0.15 for the weighted composite score) were very similar between the studies.
The significant improvement in the composite scores with haloperidol was supported by analyses of the individual measures from the primary battery and exploratory post hoc analyses of the measures from the secondary battery. The specific improvement in visuospatial working memory and Wisconsin Card Sorting Test performance with haloperidol was unexpected. Although the working memory benefit is potentially explained by baseline differences between groups on this measure, this finding parallels work completed in nonhuman primates, which, under stressful noise conditions, improve on measures of visuospatial working memory with low haloperidol doses (0.005 mg/kg), but not with higher doses (0.01 mg/kg) (43), and worsen with even higher doses (0.07–0.20 mg/kg) (44).
The potential effect of extrapyramidal symptoms and anticholinergic medication on neurocognitive performance in this study was limited to the patients who received haloperidol. Accounting for these factors had little effect on the differentiation between the treatments. Yet, even with the lower doses of haloperidol used in this study, the correlations between these factors and change in neurocognitive performance after treatment were significant in the patients who received haloperidol, suggesting that the effect of typical antipsychotics may not be neutral (19) but that some patients may have cognitive worsening and apparent increases in negative symptoms because of the side effects of these medications. Coupled with the findings of haloperidol-related improvements on several of the secondary measures, this body of work suggests the conservative conclusion that low doses of haloperidol are less deleterious than high doses in their neurocognitive effects (11, 18–20).
The neurocognitive measure that improved the most in the patients who received olanzapine was the response sensitivity measure (d′) obtained from the Continuous Performance Test, Identical Pairs Version. This measure reflects patients’ capacity to maintain vigilance in an effortful test of visual information processing that includes working memory components. This improvement was paralleled by substantial gains with olanzapine on another measure of information processing and speed, the digit-symbol test of the WAIS-R. The importance of deficits in performance on various versions of the Continuous Performance Test has been demonstrated in cognitive, functional, and neurobiological studies of schizophrenia. Compared to tasks that measure other cognitive domains, Continuous Performance Test tasks have been found to correlate more strongly with various forms of functional outcome in patients with schizophrenia (6, 8). Deficits in performance on very effortful Continuous Performance Test versions, such as the four-digit form of the Continuous Performance Test, Identical Pairs Version, have long been considered among the most fundamental impairments in schizophrenia (45). Such tests reveal deficits in children at high risk for schizophrenia (28), especially those who develop schizophrenia as adults (46), and impairments in patients with schizophrenia who perform normally in all other neurocognitive domains (47). Finally, performance on similar tasks of active maintenance of visual information is mediated in part by activation of the dorsolateral prefrontal cortex (48–50), as well as other regions (49–51). The dorsolateral prefrontal cortex tends to be less efficient in patients with schizophrenia during performance of these tasks (52, 53). The relationship between the improvement in Continuous Performance Test scores with olanzapine and the potential neurobiological changes that result from olanzapine is an intriguing subject that will be addressed in a separate manuscript that describes the neuroanatomic data generated from this study.
The data from this study suggest that a 12-week course of treatment is sufficient to detect improvement in neurocognitive functioning. Although the short-term neurocognitive effects of olanzapine do not appear to be related to acute clinical changes, it is possible that continued improvement in cognitive function may lead to improved long-term outcomes, such as improved quality of life (13). This issue will be addressed in future manuscripts generated from the 2-year component of this study.
Aspects of this study limit the generalizability of the findings. First, only 167 of 244 patients were tested at baseline and then again 12 weeks later. However, after correction for multiple comparisons, no significant differences were found between the patients who completed the 12-week trial and those who did not, suggesting that the study completers were relatively representative of the larger subject group. Second, estimates of premorbid and current intelligence were higher in the haloperidol group than in the olanzapine group. These measures were collected at the end of the 12-week trial to ensure stabilization for the larger 2-year study. It is doubtful that National Adult Reading Test scores were improved by haloperidol treatment, but this possibility cannot be ruled out. However, when National Adult Reading Test scores were added as a covariate to the between-group analyses of neurocognitive improvement, these scores did not have an effect. Third, this study allowed inclusion of patients who had received up to 16 weeks of previous antipsychotic treatment. Although very few patients had received this much previous treatment, there was considerable heterogeneity on this measure, and 28% of the patients had never taken antipsychotic medication. The inclusion of a wide variety of first-episode patients may have had an effect on the assessment of the immediate effects of these drugs on cognition.
In conclusion, patients with first-episode psychosis randomly assigned to double-blind treatment with olanzapine or low-dose haloperidol demonstrated significant improvement in neurocognitive function. The neurocognitive advantage of olanzapine over haloperidol was small. This difference approached but did not reach statistical significance in the comparison of an unweighted composite score but was statistically significant in the comparison of a weighted composite score developed from a principal-component analysis. Neurocognitive improvement in patients who received olanzapine was not related to other acute clinical improvements or side effect changes.
![]() |
![]() |
![]() |
![]() |
![]() |
![]() |
![]() |
Presented at the International Congress for Schizophrenia Research, Whistler, B.C., Canada, April 28–May 2, 2001. Received July 24, 2002; revisions received March 11 and Oct. 7, 2003; accepted Oct. 9, 2003. From the Department of Psychiatry and Behavioral Sciences, Duke University School of Medicine, Durham, N.C.; the Department of Psychiatry, Harvard Medical School, Boston; the Department of Psychiatry, University of Toronto, Toronto; the Departments of Psychiatry and Biostatistics, University of North Carolina School of Medicine, Chapel Hill, N.C.; the Clinical Neuroscience Research Centre, Dartford, Kent, U.K.; Division of Neuroscience, University Medical Center Utrecht, Utrecht, the Netherlands; the Department of Psychological and Brain Sciences, University of Louisville, Louisville; the Department of Psychiatry, University of Pennsylvania School of Medicine, Philadelphia; and Lilly Research Laboratories, Indianapolis. Address reprint requests to Dr. Keefe, Box 3270, Duke University Medical Center, Durham, NC 27710; [email protected] (e-mail). This paper was based on results from the Comparative Efficacy and Safety of Atypical and Conventional Antipsychotic Drugs in First-Episode Psychosis study conducted by the HGDH Study Group sponsored by Eli Lilly and Company. The HGDH Study Group consists of Drs. Jeffrey A. Lieberman and Diana Perkins, Department of Psychiatry, University of North Carolina School of Medicine, Chapel Hill, N.C.; Dr. Charles B. Nemeroff, Department of Psychiatry, Emory University School of Medicine, Atlanta; Dr. Bruce M. Cohen, McLean Hospital, Harvard Medical School, Belmont, Mass.; Dr. Mauricio Tohen, Lilly Research Laboratories, Indianapolis, and McLean Hospital, Belmont, Mass.; Dr. Joseph P. McEvoy, John Umstead Hospital, Butner, N.C.; Dr. John Kuldau, Department of Psychiatry, University of Florida, Gainesville, Fla.; Drs. Alan I. Green and Anthony Kalinowski, Massachusetts Mental Health Center, Harvard Medical School, Boston; Drs. Anthony J. Rothschild and Jayendra K. Patel, Department of Psychiatry, University of Massachusetts Medical Center, Worcester, Mass.; Dr. Raquel E. Gur, Department of Psychiatry, University of Pennsylvania Medical Center, Philadelphia; Drs. Robert B. Zipursky and Zafiris J. Daskalakis, Department of Psychiatry, University of Toronto School of Medicine, Toronto; Dr. Stephen M. Strakowski, Department of Psychiatry, University of Cincinnati, Cincinnati; Dr. Ira Glick, Department of Psychiatry, Stanford University School of Medicine, Stanford, Calif.; Dr. John De Quardo, Department of Psychiatry, University of Michigan Medical Center, Ann Arbor, Mich.; Prof. Dr. R.S. Kahn, University Hospital Utrecht, Utrecht, the Netherlands; Dr. Tonmoy Sharma, Clinical Neuroscience Research Centre, Dartford, Kent, U.K.; and Prof. Robin Murray, Institute of Psychiatry, London.
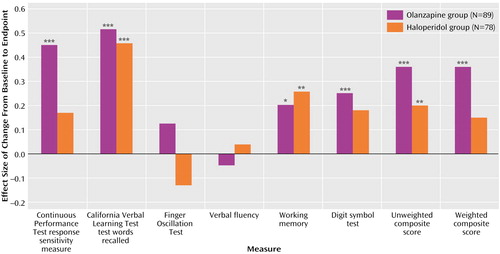
Figure 1. Effect Size of Change in Neurocognitive Measures After 12 Weeks of Treatment With Olanzapine and Low Doses of Haloperidol in Patients With First-Episode Psychosisa,b
aSignificant within-group differences between baseline and endpoint scores are indicated with asterisks.
bOlanzapine dose range = 5–20 mg/day. Haloperidol dose range = 2–20 mg/day.
*p<0.05. **p<0.01. ***p<0.001.
1. Harvey PD, Keefe RSE: Cognitive impairment in schizophrenia and implications of atypical neuroleptic treatment. CNS Spectrums 1997; 2:1–11Google Scholar
2. Heinrichs RW, Zakzanis KK: Neurocognitive deficit in schizophrenia: a quantitative review of the evidence. Neuropsychology 1998; 12:426–445Crossref, Medline, Google Scholar
3. Hoff AL, Riordan H, O’Donnell DW, Morris L, DeLisi LE: Neuropsychological functioning of first-episode schizophreniform patients. Am J Psychiatry 1992; 149:898–903Link, Google Scholar
4. Saykin AJ, Shtasel DL, Gur RE, Kester DB, Mozley LH, Stafiniak P, Gur RC: Neuropsychological deficits in neuroleptic naive patients with first-episode schizophrenia. Arch Gen Psychiatry 1994; 51:124–131Crossref, Medline, Google Scholar
5. Bilder RM, Goldman RS, Robinson D, Reiter G, Bell L, Bates JA, Pappadopulos E, Willson DF, Alvir JMJ, Woerner MG, Geisler S, Kane JM, Lieberman JA: Neuropsychology of first-episode schizophrenia: initial characterization and clinical correlates. Am J Psychiatry 2000; 157:549–559Link, Google Scholar
6. Green MF: What are the functional consequences of neurocognitive deficits in schizophrenia? Am J Psychiatry 1996; 153:321–330Link, Google Scholar
7. Harvey PD, Howanitz E, Parrella M, White L, Davidson M, Mohs RC, Hoblyn J, Davis KL: Symptoms, cognitive functioning, and adaptive skills in geriatric patients with lifelong schizophrenia: a comparison across treatment sites. Am J Psychiatry 1998; 155:1080–1086Link, Google Scholar
8. Green MF, Kern RS, Braff DL, Mintz J: Neurocognitive deficits and functional outcome in schizophrenia: are we measuring the “right stuff”? Schizophr Bull 2000; 26:119–136Crossref, Medline, Google Scholar
9. Blyler CR, Gold JM: Cognitive effects of typical antipsychotic treatment: another look, in Cognitive Deficits in Schizophrenia. Edited by Sharma T, Harvey PD. Oxford, Oxford University Press, 2000, pp 241–265Google Scholar
10. Meltzer HY, McGurk SR: The effects of clozapine, risperidone, and olanzapine on cognitive function in schizophrenia. Schizophr Bull 1999; 25:233–255Crossref, Medline, Google Scholar
11. Harvey PD, Keefe RSE: Studies of cognitive change in patients with schizophrenia following novel antipsychotic treatment. Am J Psychiatry 2001; 158:176–184Link, Google Scholar
12. Lee MA, Thompson PA, Meltzer HY: Effects of clozapine on cognitive function in schizophrenia. J Clin Psychiatry 1994; 55(Sept suppl B):82–87Google Scholar
13. Buchanan RW, Holstein C, Breier A: The comparative efficacy and long-term effect of clozapine treatment on neuropsychological test performance. Biol Psychiatry 1994; 36:717–725Crossref, Medline, Google Scholar
14. Bilder RM, Goldman RS, Volavka J, Czobor P, Hoptman M, Sheitman B, Lindenmayer J-P, Citrome L, McEvoy J, Kunz M, Chakos M, Cooper TB, Horowitz TL, Lieberman JA: Neurocognitive effects of clozapine, olanzapine, risperidone, and haloperidol in patients with chronic schizophrenia or schizoaffective disorder. Am J Psychiatry 2002; 159:1018–1028Link, Google Scholar
15. Green MF, Marshall BD Jr, Wirshing WC, Ames D, Marder SR, McGurk S, Kern RS, Mintz J: Does risperidone improve verbal working memory in treatment-resistant schizophrenia? Am J Psychiatry 1997; 154:799–804Link, Google Scholar
16. Purdon SE, Jones BD, Stip E, Labelle A, Addington D, David SR, Breier A, Tollefson GD (Canadian Collaborative Group for Research in Schizophrenia): Neuropsychological change in early phase schizophrenia during 12 months of treatment with olanzapine, risperidone, or haloperidol. Arch Gen Psychiatry 2000; 57:249–258Crossref, Medline, Google Scholar
17. Velligan DI, Newcomer J, Pultz J, Csernansky J, Hoff AL, Mahurin RK, Miller AL: Does cognitive function improve with quetiapine in comparison to haloperidol? Schizophr Res 2002; 53:239–248Crossref, Medline, Google Scholar
18. Keefe RSE, Silva SG, Perkins DO, Lieberman JA: The effects of atypical antipsychotic drugs on neurocognitive impairment in schizophrenia: a review and meta-analysis. Schizophr Bull 1999; 25:201–222Crossref, Medline, Google Scholar
19. Carpenter WT, Gold JM: Another view of therapy for cognition in schizophrenia. Biol Psychiatry 2002; 51:969–971Crossref, Medline, Google Scholar
20. Green MF, Marder SR, Glynn SM, McGurk SR, Wirshing WC, Wirshing DA, Liberman RP, Mintz J: The neurocognitive effects of low-dose haloperidol: a two-year comparison with risperidone. Biol Psychiatry 2002; 51:972–978Crossref, Medline, Google Scholar
21. Seidman LJ, Pepple JR, Faraone SV, Kremen WS, Green AI, Brown WA, Tsuang MT: Neuropsychological performance in chronic schizophrenia in response to neuroleptic dose reduction. Biol Psychiatry 1993; 33:575–584Crossref, Medline, Google Scholar
22. Sweeney JA, Keilp JG, Haas GL, Hill J, Weiden PJ: Relationships between medication treatments and neuropsychological test performance in schizophrenia. Psychiatry Res 1991; 37:297–308Crossref, Medline, Google Scholar
23. Kay SR, Fiszbein A, Opler LA: The Positive and Negative Syndrome Scale (PANSS) for schizophrenia. Schizophr Bull 1987; 13:261–276Crossref, Medline, Google Scholar
24. Montgomery SA, Åsberg M: A new depression scale designed to be sensitive to change. Br J Psychiatry 1979; 134:382–389Crossref, Medline, Google Scholar
25. Nelson HE: National Adult Reading Test Manual (NART), 2nd ed. Windsor, UK, National Foundation for Educational Research-Nelson, 1991Google Scholar
26. Wechsler D: Wechsler Adult Intelligence Scale—Revised. San Antonio, Tex, Psychological Corp, 1974Google Scholar
27. Oldfield RC: The assessment and analysis of handedness: the Edinburgh Inventory. Neuropsychologia 1971; 9:97–113Crossref, Medline, Google Scholar
28. Cornblatt BA, Keilp JG: Impaired attention, genetics, and the pathophysiology of schizophrenia. Schizophr Bull 1994; 20:31–46Crossref, Medline, Google Scholar
29. Benton AL, Hamsher K: Multilingual Aphasia Examination Manual, Revised. Iowa City, University of Iowa, 1978Google Scholar
30. Delis DC, Kramer JH, Kaplan E, Ober BA: The California Verbal Learning Test Manual. New York, Psychological Corp, 1987Google Scholar
31. Keefe RSE, Roitman SE, Harvey PD, Blum CS, DuPre RL, Prieto DM, Davidson M, Davis KL: A pen-and-paper human analogue of a monkey prefrontal cortex activation task: spatial working memory in patients with schizophrenia. Schizophr Res 1995; 17:25–33Crossref, Medline, Google Scholar
32. Gold JM, Carpenter C, Randolph C, Goldberg TE, Weinberger DR: Auditory working memory and Wisconsin Card Sorting Test performance in schizophrenia. Arch Gen Psychiatry 1997; 54:159–165Crossref, Medline, Google Scholar
33. Spreen O, Strauss E: A Compendium of Neuropsychological Tests: Administration, Norms, and Commentary, 2nd ed. New York, Oxford University Press, 1998Google Scholar
34. Heaton RK, Chelune GJ, Talley JL, Kay GG, Curtiss G: Wisconsin Card Sorting Test Manual. Odessa, Fla, Psychological Assessment Resources, 1993Google Scholar
35. Bilder RM, Turkel E, Bates JA, Goldman RS, Lieberman JA, Schooler N, Robinson D, Kane JM: Concurrent validation of computerized neurocognitive assessment tools: is the mouse mightier than the pen? (abstract). Biol Psychiatry 2001, 49(suppl 1):56SGoogle Scholar
36. Seidman LJ, Oscar-Berman M, Kalinowski AG, Ajilore O: Experimental and clinical neuropsychological measures of prefrontal dysfunction in schizophrenia. Neuropsychology 1995; 9:481–490Crossref, Google Scholar
37. Golden CJ: Stroop Color and Word Test: A Manual for Clinical and Experimental Uses. Wood Dale, Ill, Stoelting Co, 1978Google Scholar
38. Ruff RM: Ruff Figural Fluency Test Manual. Odessa, Fla, Psychological Assessment Resources, 1996Google Scholar
39. Wechsler D: Wechsler Memory Scale—Revised. San Antonio, Tex, Psychological Corp, 1987Google Scholar
40. Benton AL, Sivan AB, Hamsher KD, Varney NR, Spreen O: Contributions to Neuropsychological Assessment: A Clinical Manual, 2nd ed. New York, Oxford University Press, 1994Google Scholar
41. Simpson GM, Angus JWS: A rating scale for extrapyramidal side effects. Acta Psychiatr Scand Suppl 1970; 212:11–19Crossref, Medline, Google Scholar
42. Barnes TRE: A rating scale for drug-induced akathisia. Br J Psychiatry 1989; 154:672–676Crossref, Medline, Google Scholar
43. Arnsten AF, Goldman-Rakic PS: Noise stress impairs prefrontal cortical cognitive function in monkeys: evidence for a hyperdopaminergic mechanism. Arch Gen Psychiatry 1998; 55:362–368Crossref, Medline, Google Scholar
44. Castner SA, Williams GV, Goldman-Rakic PS: Reversal of antipsychotic-induced working memory deficits by short-term dopamine D1 receptor stimulation. Science 2000; 287:2020–2022Crossref, Medline, Google Scholar
45. Seidman LJ: Schizophrenia and brain dysfunction: an integration of recent neurodiagnostic findings. Psychol Bull 1983; 94:195–238Crossref, Medline, Google Scholar
46. Cornblatt B, Obuchowski M, Roberts S, Pollack S, Erlenmeyer-Kimling L: Cognitive and behavioral precursors of schizophrenia. Dev Psychopathol 1999; 11:487–508Crossref, Medline, Google Scholar
47. Weickert TW, Goldberg TE, Gold JM, Bigelow LB, Egan MF, Weinberger DR: Cognitive impairments in patients with schizophrenia displaying preserved and compromised intellect. Arch Gen Psychiatry 2000; 57:907–913Crossref, Medline, Google Scholar
48. Callicott JH, Mattay VS, Bertolino A, Finn K, Coppola R, Frank JA, Goldberg TE, Weinberger DR: Physiological characteristics of capacity constraints in working memory as revealed by functional MRI. Cereb Cortex 1999; 9:20–26Crossref, Medline, Google Scholar
49. Cohen JD, Perlstein WM, Braver TS, Nystrom LE, Noll DC, Jonides J, Smith EE: Temporal dynamics of brain activation during a working memory task. Nature 1997; 386:604–608Crossref, Medline, Google Scholar
50. Keilp JG, Herrera J, Stritzke P, Cornblatt BA: The Continuous Performance Test, Identical Pairs Version (CPT-IP), III: brain functioning during performance of numbers and shapes subtasks. Psychiatry Res 1997; 74:35–45Crossref, Medline, Google Scholar
51. Hager F, Volz HP, Gaser C, Mentzel HJ, Kaiser WA, Sauer H: Challenging the anterior attentional system with a continuous performance task: a functional magnetic resonance imaging approach. Eur Arch Psychiatry Clin Neurosci 1998; 248:161–170Crossref, Medline, Google Scholar
52. Calicott JH, Ramsey NF, Tallent K, Bertolino A, Knable MB, Coppola R, Goldberg T, van Gelderen P, Mattay VS, Frank JA, Moonen CT, Weinberger DR: Functional magnetic resonance imaging brain mapping in psychiatry: methodological issues illustrated in a study of working memory in schizophrenia. Neuropsychopharmacology 1998; 18:186–196Crossref, Medline, Google Scholar
53. Barch DM, Carter CS, Braver TS, Sabb FW, MacDonald A III, Noll DC, Cohen JD: Selective deficits in prefrontal cortex function in medication-naive patients with schizophrenia. Arch Gen Psychiatry 2001; 58:280–288Crossref, Medline, Google Scholar