Absence of Histological Lesions in Primate Models of ECT and Magnetic Seizure Therapy
Abstract
OBJECTIVE: The authors present preliminary findings from the first nonhuman primate neuropathological study of ECT to use perfusion fixation and adequate controls and the first to compare ECT with magnetic seizure therapy, to their knowledge. METHOD: Twelve Macaca mullata received 6 weeks of daily ECT, magnetic seizure therapy, or anesthesia alone. After perfusion fixation, their brains were examined while masked to intervention. RESULTS: No identified lesions were attributable to the interventions. Cortical and hippocampal immunoreactivity for glial fibrillary acidic protein (an astrocytic marker) was most intense in the group that received ECT. CONCLUSIONS: This small but rigorous primate study supports the view that ECT does not produce histological lesions in the brain and provides the first comparable safety data on magnetic seizure therapy.
While critics have stated that ECT results in cell death and structural brain damage, the literature fails to confirm this (1). Investigations in epilepsy have shown that the conditions needed to result in cell loss do not occur with ECT (2). Most experimental neuropathological studies of ECT have used rodents. Nonhuman primate investigations, mostly conducted some 50 years ago, were flawed. Limitations included a lack of random assignment and masked histological evaluations or a lack of control animals altogether. Outdated ECT procedures could have resulted in hypoxia or prolonged seizures. Most critically, handling the brains before fixation introduced neuropathological artifacts. We present preliminary results from the first study to our knowledge to apply modern methods of ECT administration in nonhuman primates and to use perfusion fixation and masked, controlled evaluation of potential neuropathological effects. This report also was prompted by the development of magnetic seizure therapy, a form of convulsive treatment that may have benefits relative to ECT (3).
Method
Twelve adolescent Macaca mulatta, the initial subjects in an ongoing study of the cognitive and anatomic effects of ECT and magnetic seizure therapy, were divided into cohorts of three and matched for age, weight, and sex. Each cohort was housed in a group. Within each cohort, the monkeys were randomly assigned to ECT, magnetic seizure therapy, or sham. All staff not involved in the delivery of the interventions were masked to group assignment. This study was approved by the Institutional Animal Care and Use Committee of New York State Psychiatric Institute.
Interventions were performed 4 days per week for 6 weeks. A 5-week recovery period was interposed before the last intervention week to permit maturation of possible neuropathological effects. Animals were euthanized 3 days after the last intervention.
Monkeys were sedated with intramuscular ketamine (5 mg/kg) and intramuscular xylazine (0.35 mg/kg). Like human ECT, interventions were administered under general anesthesia with intravenous methohexital (0.5 mg/kg), muscle relaxation with intravenous succinylcholine (3.5 mg/kg), and continuous ventilatory support (100% O2 positive pressure). Bilateral ECT and magnetic seizure therapy were administered at 2.5 times an individual monkey’s seizure threshold, approximating high doses of bilateral ECT in patients. ECT was delivered with the Spectrum 5000Q (MECTA Corp., Lake Oswego, Ore.). Magnetic seizure therapy used a custom repetitive stimulator with enhanced output (Magstim Company Limited, Whitland, South West Wales, U.K.). Sham interventions were identical but were without brain stimulation. Physiological monitoring followed guidelines for human ECT.
Before removal from the skull, brains were fixed by transcardiac perfusion with 50 mM Na2S followed by 10% buffered formalin. Frozen 40-μ sections of the left hemisphere, from the amygdala through the hippocampus, were stained at 1.6-mm intervals with hematoxylin and eosin, cresyl violet, and immunocytochemical stains for microtubule-associated protein 2 (a cytoskeletal protein of dendrites) and glial fibrillary acidic protein (a cytoskeletal protein of astrocytes). Immunocytochemical staining used the avidin-biotinylated peroxidase method (4) after boiling in tris buffer (5). The chromogen was diaminobenzidine, with nickel intensification (6). The brainstem was similarly sectioned and stained with hematoxylin and eosin. An experienced neuropathologist (A.J.D.) examined all sections, which were masked to intervention.
Glial fibrillary acidic protein staining was examined with a 10× loupe in the superior frontal gyrus, the middle temporal gyrus, the putamen, the amygdala, the entorhinal cortex, the dentate gyrus (granular cell layer and hilus), and the pyramidal cell layer of hippocampal field CA1. In addition, digital photomicrographs were prepared by the observer under identical conditions of illumination and exposure and compared in equivalent regions of the molecular layer of the hippocampal dentate gyrus and the lacunosum-moleculare of CA1 (Figure 1). Within each cohort, the three animals were ranked from darkest to lightest stained in each region.
Results
A microscopic focus of congenital cortical dysplasia was present in a sham-treated monkey. In another sham-treated monkey, there were a few eosinophilic pyramidal neurons in the CA1 sector of the hippocampus. Several similar neurons were present in one microscopic focus in the deep layers of the parietal cortex in one animal treated with magnetic seizure therapy. Neither hematoxylin and eosin nor glial fibrillary acidic protein staining revealed any reaction to these lesions. No other abnormalities were seen.
Immunohistochemistry for microtubule-associated protein 2 showed strong staining of dendrites and occasional neuronal cell bodies in all regions of gray matter. Qualitative evaluation revealed no differences between groups or any abnormalities in individual animals.
Glial fibrillary acidic protein staining revealed no focal astrocytosis. There was a statistically significant effect of treatment on diffuse glial fibrillary acidic protein immunoreactivity in the superior frontal gyrus (Friedman’s χ2=7, df=2, p=0.03), where immunoreactivity was the greatest in the ECT group and the least in the sham group for all four cohorts. In three of the four cohorts, this order was maintained in the pyramidal layer of CA1 and the molecular layer of the dentate gyrus, and the ECT group exceeded the sham group in the amygdala.
Discussion
We report an absence of neuropathological lesions when ECT and magnetic seizure therapy were given intensively in a nonhuman primate model. The study mimicked clinical conditions more closely than had ever been done previously. We avoided the confounds of earlier studies by using general anesthesia, muscle relaxation, oxygenation, seizure and physiological monitoring, and formalin perfusion before removal of the brain. The number of interventions and their frequency were at the upper limit of what would be used clinically. The 5-week recovery period before the last week of intervention allowed detection of acute, evolving, or mature changes.
None of the ECT-treated monkeys showed pathological findings. The finding compatible with pathological change was eosinophilia of a few neurons in the CA1 region of one sham-treated animal and in a single microscopic focus of the parietal cortex in one animal treated with magnetic seizure therapy. The appearance of these neurons was consistent with acute hypoxia. However, these changes were too pathologically acute to be linked to magnetic seizure therapy or accompanying anesthesia. We saw no neuronophagia or focal astrocytosis, which would have developed between intervention and euthanization. In contrast, incidental hypoxic change could have appeared in rare neurons during the procedures used for euthanization.
Diffuse glial fibrillary acidic protein immunoreactivity in the superior frontal gyrus, the amygdala, and the hippocampus was greatest in ECT-treated monkeys, probably indicating widespread astrocytic activation, although the number of animals is too small for meaningful statistical evaluation. This possibility is consistent with ECT studies in rodents (7). While the time course of glial fibrillary acidic protein immunoreactivity after ECT has not been described, mRNA levels peak in the first few days and then decline, suggesting a transient reaction to neuronal excitation (8). Similarly, spreading depression does not cause neuronal injury but temporarily stimulates glial fibrillary acidic protein expression in the rat (9, 10).
The group was too small for us to detect subtle, quantitative changes such as smaller numbers of neurons or larger numbers of microglia. Many more animals would be needed to rule out lesions that occur infrequently, but small groups are inherent to terminal primate studies. Although animal studies cannot fully represent the cumulative effects of a lifetime of treatment and illness, primates offer the closest possible models of the direct impact of interventions on the human brain. Another concern is that however well masked the examiner, negative findings could result from a rating bias against identifying any pathological changes. The documentation of neuronal eosinophilia argues against such a bias.
It has been common to interpret the cognitive effects of ECT as evidence of structural brain damage. However, cognitive changes, such as the amnestic effects of short-acting sedatives, can occur without cell loss or injury. Despite the limitations of this study, the absence of pathological findings provides empirical evidence that routine use of convulsive therapy does not produce structural brain damage.
Received Feb. 19, 2003; revision received July 9, 2003; accepted July 24, 2003. From the Magnetic Brain Stimulation Laboratory, the Departments of Biological Psychiatry and Neuroscience, New York State Psychiatric Institute; and the Departments of Psychiatry, Pathology, Radiology, and Anatomy and Cell Biology, College of Physicians and Surgeons of Columbia University, New York. Address reprint requests to Dr. Dwork, Department of Neuroscience, New York State Psychiatric Institute, 1051 Riverside Dr., Unit 62, New York, NY 10032; [email protected] (e-mail). Supported in part by NIMH grants MH-60884 and MH-01577 (to Dr. Lisanby), grant MH-35636 (to Dr. Sackeim), a Distinguished Investigator Award (to Dr. Sackeim), and Young Investigator Awards (to Drs. Ilievski and Rosoklija) from the National Alliance for Research on Schizophrenia and Depression. The authors thank Lili Bernstein, B.A., Alma Campos, B.A., Edward Kwon, B.A., Bruce Luber, Ph.D., and Vilas Patel for their assistance with this project.
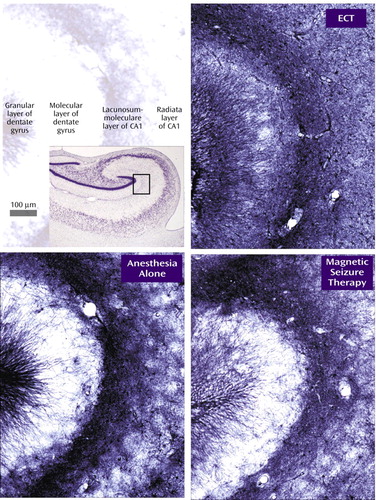
Figure 1. Immunohistochemistry for Glial Fibrillary Acidic Protein in a Representative Cohort of Rhesus Monkeysa
aThe upper left panel indicates the locations of the granular and molecular layers of the dentate gyrus and the lacunosum-moleculare and radiata layers of CA1. The Nissl-stained image in the inset box contains a rectangle that indicates the position of the glial fibrillary acidic protein photomicrographs.
1. Devanand DP, Dwork AJ, Hutchinson ER, Bolwig TG, Sackeim HA: Does ECT alter brain structure? Am J Psychiatry 1994; 151:957–970Link, Google Scholar
2. Meldrum BS: Neuropathological consequences of chemically and electrically induced seizures. Ann NY Acad Sci 1986; 462:186–193Crossref, Medline, Google Scholar
3. Lisanby SH, Luber B, Schlaepfer T, Sackeim HA: Safety and feasibility of magnetic seizure therapy (MST) in major depression: randomized within-subject comparison with electroconvulsive therapy. Neuropsychopharmacology 2003; 28:1852–1865Crossref, Medline, Google Scholar
4. Hsu SM, Raine L, Fanger H: Use of avidin-biotin-peroxidase complex (ABC) in immunoperoxidase techniques: a comparison between ABC and unlabeled antibody (PAP) procedures. J Histochem Cytochem 1981; 29:577–580Crossref, Medline, Google Scholar
5. Cattoretti G, Pileri S, Parravicini C, Becker MH, Poggi S, Bifulco C, Key G, D’Amato L, Sabattini E, Feudale E, et al: Antigen unmasking on formalin-fixed, paraffin-embedded tissue sections. J Pathol 1993; 171:83–98Crossref, Medline, Google Scholar
6. Adams JC: Heavy metal intensification of DAB-based HRP reaction product (letter). J Histochem Cytochem 1981; 29:775Crossref, Medline, Google Scholar
7. Kragh J, Bolwig TG, Woldbye DP, Jorgensen OS: Electroconvulsive shock and lidocaine-induced seizures in the rat activate astrocytes as measured by glial fibrillary acidic protein. Biol Psychiatry 1993; 33:794–800Crossref, Medline, Google Scholar
8. Steward O: Electroconvulsive seizures upregulate astroglial gene expression selectively in the dentate gyrus. Brain Res Mol Brain Res 1994; 25:217–224Crossref, Medline, Google Scholar
9. Kraig RP, Dong LM, Thisted R, Jaeger CB: Spreading depression increases immunohistochemical staining of glial fibrillary acidic protein. J Neurosci 1991; 11:2187–2198Crossref, Medline, Google Scholar
10. Bonthius DJ, Stringer JL, Lothman EW, Steward O: Spreading depression and reverberatory seizures induce the upregulation of mRNA for glial fibrillary acidic protein. Brain Res 1994; 645:215–224Crossref, Medline, Google Scholar