Functional Magnetic Resonance Imaging Evidence for a Lack of Striatal Dysfunction During Implicit Sequence Learning in Individuals With Animal Phobia
Abstract
OBJECTIVE: This study investigated the neural substrates of implicit sequence learning in subjects with and without small animal phobia, in a follow-up to analogous studies of obsessive-compulsive disorder (OCD). METHOD: Ten subjects with specific phobia and 10 healthy comparison subjects were studied by using a serial reaction time task paradigm and functional magnetic resonance imaging. RESULTS: A main effect of condition (implicit sequence learning versus random sequence) was observed across diagnostic groups in the right striatum, as well as in other regions. In the striatum, the a priori region of interest, there were no significant effects of diagnosis or the interaction of diagnosis and condition. CONCLUSIONS: Brain activation in the striatum of subjects with specific phobia does not significantly differ from that of normal comparison subjects during implicit sequence learning. This suggests different pathophysiological mechanisms for specific phobia in contrast to OCD, in which deficient striatal recruitment has been reproducibly found with this paradigm. This approach offers promise for demonstrating diagnostic specificity across different neuropsychiatric disorders based on the presence or absence of deficient striatal activation.
The basal ganglia have been implicated in several cognitive and motor processes, one of which is implicit or procedural learning (1–3). The serial reaction time task has been used as an experimental paradigm for measuring implicit sequence learning (4). In combination with functional neuroimaging, the serial reaction time task has been used to demonstrate that the striatum (i.e., caudate and putamen) is engaged during implicit sequence learning in healthy subjects (2, 3, 5). Striatal dysfunction is strongly implicated in the pathophysiology of obsessive-compulsive disorder (OCD); this prompted earlier work comparing striatal function in patients with OCD and healthy comparison subjects by using the serial reaction time task and functional neuroimaging. We used positron emission tomography (PET) and the serial reaction time task (6) to demonstrate that adults with OCD display a deficit in striatal activation in relation to healthy comparison subjects during implicit sequence learning. We subsequently replicated this finding using the serial reaction time task in conjunction with functional magnetic resonance imaging (fMRI) (5, 7). However, the specificity of this striatal deficit in OCD in comparison to other psychiatric disorders has not been established. Consequently, in the current study, we sought to investigate whether subjects with another common anxiety disorder (specific phobia) would also exhibit striatal dysfunction in relation to healthy comparison subjects with the serial reaction time task and fMRI. Our a priori hypothesis was that the deficit in striatal activation during implicit learning found in OCD would not be present in subjects with specific phobia compared to healthy comparison subjects.
Method
Subjects
Ten adults with a SCID-derived DSM-IV diagnosis of specific phobia and 10 healthy comparison subjects who were matched for age (mean=29.8 years, SD=6.8, versus mean=26.7, SD=6.7, respectively) (F=1.07, df=1, 18, p=0.32), gender (six women and four men), right-handedness (8), and educational level (mean=17.4 years, SD=2.5, versus mean=17.0, SD=2.2) (F=0.15, df=1, 18, p=0.71) were studied. To increase homogeneity in the group with specific phobia, only individuals with small animal phobias were included (i.e., in this cohort, subjects had phobias to spiders, snakes, lizards, and insects). All subjects had no history of significant neurological or medical conditions, had not been taking psychotropic medications for at least 6 weeks, and did not have any other current axis I disorder. This investigation was conducted in accordance with the guidelines of the Subcommittee on Human Studies of Massachusetts General Hospital, and written informed consent was obtained from each subject. The current study was powered based on two previous neuroimaging studies that used the serial reaction time task to compare patients with OCD and matched comparison subjects (6, 7). Both of those experiments found between-group effects sizes of >1.2 (9). Therefore, in the current experiment, we planned to study 10 subjects in each group in order to provide >75% power to detect a difference of comparable effect size between the two groups at a statistical threshold of p=0.05.
The Serial Reaction Time Task
Use of the serial reaction time task, modified for fMRI, has been previously described in detail (5). In brief, each trial consisted of an asterisk appearing in one of four horizontally arranged boxes for 1.0 seconds, followed by a gap of 0.2 seconds in which no asterisk was presented. The subjects were instructed to press one of four spatially corresponding keys in immediate response to the appearance of the asterisk by using a separate finger for each key (left and right index and middle fingers). A 96-trial practice run was followed by two runs (each lasting ∼7.5 minutes and consisting of 312 trials). Each run consisted of contiguous alternating blocks of random and implicit learning conditions bracketed by a 27.5-second fixation block (i.e., low-level baseline): random/implicit learning/random/implicit learning/random/implicit learning/random. In the implicit learning condition, the stimuli followed a 12-item sequence (position: 1-2-1-4-2-3-4-1-3-2-4-3) that repeated six times for a total of 72 trials. The random condition entailed 24 stimuli in which stimulus locations were pseudorandomly determined. The random and implicit learning blocks were not marked or distinguished before or during task administration. A Sharp XG-2000-V color liquid crystal display projector (Osaka, Japan) was used for stimulus presentation; reaction time and response accuracy were recorded.
Debriefing
Immediately following the two experimental runs, the subjects completed a computerized debriefing procedure, as previously described (2, 5). After answering multiple-choice questions to assess their awareness of the stimuli, the subjects were informed that a sequence had been present and were asked to attempt to recall the sequence by making 15 key presses. The recall task was scored based on the longest consecutive string of correct responses, which was then compared with a chance distribution as an index of significant explicit knowledge. All subjects also completed the following self-report measures of anxiety and depression: the Beck Anxiety Inventory (10) and the Beck Depression Inventory (11).
fMRI
A 1.5-T imaging device equipped for echo-planar imaging was used with a three-axis gradient head coil. After an automated scout image was made and appropriate shimming was conducted, two high-resolution three-dimensional magnetization prepared rapid gradient echo (MP-RAGE) sequences (TR=7.25 msec, TE=3 msec, flip angle=7°, voxel size=1.3×1×1 mm) were collected for spatial normalization and positioning. fMRI images were acquired by using a gradient echo, T2*-weighted sequence (TR=2.75 sec, TE=40 msec, flip angle=90°). T2 images were collected in an oblique coronal plane (24 slices perpendicular to the commissural line, with a voxel size of 8×3.125×3.125 mm). For each fMRI run, measurements from 160 time points were acquired.
Analysis
Each functional run was motion-corrected to the first run by using AFNI software (12, 13) (http://afni.nimh.nih.gov/afni/index.shtml), spatially smoothed (full width at half maximum=5 mm) with a three-dimensional Gaussian filter (http://www.fmrib.ox.ac.uk/fsl), and normalized to the low-level baseline (i.e., the fixation block). Normalized, motion-corrected functional images were aligned to a three-dimensional structural image created by motion-correcting and averaging two high-resolution three-dimensional sagittal images. After Talairach transformation (14, 15), functional data were averaged across all subjects according to condition. A t statistic for the group statistical maps was then computed on a voxel-wise basis for the main contrast of interest (i.e., implicit learning versus random) by using a fixed-effects model. The threshold for significance of the striatum, our a priori region of interest, was p≤2×10–4; for the remaining subcortical regions, the adjusted threshold was p≤6.6×10–5; for the cerebral cortex, the threshold was p≤5.8×10–6. This reflects Bonferroni corrections for multiple comparisons based on voxel size (8×3.125×3.125 mm) and the average total volume of the relevant structures in healthy young adults (16).
To assess for differences between groups in the striatum, a functionally derived region-of-interest analysis was performed. A region of interest was defined as 3 or more contiguous voxels exceeding the statistical threshold (i.e., p≤2×10–4). Significant activations were found within the right caudate and right putamen. Labels were made based on the Talairach coordinates of the functional voxels in these striatal regions of interest; blood-oxygen-level-dependent (BOLD) signal intensity data were extracted from the functional images of each subject. Percent BOLD signal change for each condition versus baseline values was entered into an analysis of variance for repeated measures to assess main effects of condition (i.e., implicit learning or random) across the entire cohort and diagnosis (specific phobia or healthy). A post hoc voxel-based random-effects analysis was also performed to compare brain responses between groups for the implicit learning versus random task contrast and to confirm the results of the region-of-interest analysis.
Results
Subject and Data Selection
Twelve subjects with specific phobia and 17 healthy comparison subjects were initially tested. Two subjects with specific phobia and three healthy comparison subjects were eliminated because of image quality and explicit contamination (i.e., more than six consecutive correct responses on the debriefing task), respectively. Ten healthy comparison subjects were selected blind to individual fMRI or other behavioral data from the remaining 14 to best match the age, gender, educational levels, and the number of subjects in the specific phobia group. Debriefing data for one specific phobia subject was missing (reaction time data was complete, however). Analysis of reaction time data revealed a mean reaction time advantage or improvement of 16.1 msec for this subject (less than the mean for the specific phobia group of 34.0 msec), which was not indicative of explicit contamination. Therefore, this subject’s data was included; thus, 10 subjects with specific phobia and 10 healthy comparison subjects were included in the final analysis. There was no difference in the index of explicit contamination (maximum consecutive correct responses on the debriefing task [2, 5]) in either the initial cohort (11 subjects with specific phobia versus 17 healthy comparison subjects) (t=–1.80, df=26, p=0.08) or the final study cohort (nine subjects with specific phobia versus 10 healthy comparison subjects) (t=–0.65, df=17, p=0.52).
Behavioral Performance
At the time of assessment in our laboratory, there were no significant differences between the subjects with specific phobia and the healthy comparison subjects regarding measures of anxiety or depression (Beck Depression Inventory score—subjects with specific phobia: mean=1.5, SD=1.3; comparison subjects—mean=3.1, SD=2.7; F=2.90, df=1, 18, p=0.11; Beck Anxiety Inventory score—mean=4.8, SD=4.9; mean=3.4, SD=3.6, respectively (F=0.53, df=1, 18, p=0.48).
The debriefing task revealed an explicit contamination index of 4 (SD=1) across the cohort (all ≤5; chance performance, mean=3.71, SD=1.26), such that individual scores greater than 6 were suggestive of significant explicit knowledge. Error rates were less than 6% for all subjects (subjects with specific phobia: mean=2.19%, SD=1.80%; comparison subjects: mean=2.07%, SD=1.85%) (F=0.02, df=1, 18, p=0.88). There was a significant reaction time advantage for the implicit learning versus random condition across subject groups (implicit learning: mean-median reaction time=410.5 msec, SD=51.7; random: mean-median=443.7 msec, SD=55.1) (F=159.58, df=1, 18, p<0.0001), reflecting implicit learning but no between-group difference (F=0.05, df=1, 18, p=0.83).
fMRI Results
For the main contrast (implicit learning versus random) across groups with a fixed-effects model, the foci of significant activation were found within the right caudate (four voxels; Talairach coordinates of peak voxel: x=13.9, y=3.1, and z=13.7; p=1.45×10–7), the right putamen (eight voxels; peak: x=26.0, y=–1.8, and z=1.9; p=1.7×10–6) (Figure 1), and in the left thalamus (seven voxels; peak: x=–13.4, y=–10.6, and z=1.0; p=1.14×10–7). In the cortical search volume, there were significant activations in the left insula (three voxels; peak: x=–37.4, y=–25.3, and z=17.6; p=1.02×10–6), the left superior temporal gyrus (five voxels; peak: x=–61.4, y=–48.3, and z=15.8; p=1.7×10–8), and the bilateral mid-cingulate cortex (11 voxels; peak: x=–2.0, y=–13.7, and z=36.2; p=2.45×10–11).
Of note, when we used a more stringent random-effects model, a similar pattern of activations was found for the implicit learning versus random contrast; however, this did not meet the threshold for statistical significance.
Regions of interest were defined based upon the findings of the fixed-effects analysis. In the striatum, our a priori region of interest, region-of-interest analysis confirmed a main effect of condition (implicit learning versus random) in both of the regions of interest in the right caudate (F=17.9, df=1, 18, p=0.0005) and the putamen (F=7.8, df=1, 18, p<0.02). However, there was no main effect of diagnosis (caudate: F=0.2, df=1, 18, p=0.66; putamen: F=0.2, df=1, 18, p=0.66) or the diagnosis-by-condition interaction (caudate: F=0.0, df=1, 18, p=0.99; putamen: F=0.01, df=1, 18, p=0.94) in either striatal region. Post hoc voxel-wise random effects analysis also revealed no between-group difference in the striatum or in any other brain areas for the implicit learning versus random contrast.
Discussion
Previous PET and fMRI studies have demonstrated impaired striatal recruitment in patients with OCD in relation to healthy comparison subjects with comparable behavioral performance (6, 7). The present study investigated the specificity of these findings by examining a group of subjects with specific phobia, a disorder included in the same nosological category as OCD (anxiety disorders, DSM-IV). According to our a priori hypothesis, the patients with specific phobia would not differ from the healthy comparison subjects in behavioral and brain responses while performing the serial reaction time task because cortical-striatal dysfunction is not proposed in contemporary neurocircuitry models of specific phobia (17, 18). Indeed, both the specific phobia and healthy comparison groups demonstrated implicit learning, which was associated with activations in the right striatum, as well as the thalamus and several regions of the cerebral cortex. Furthermore, there were no main effects of diagnosis or diagnosis-by-condition interactions in the striatum, our a priori region of interest. These findings replicate previous PET and fMRI studies of the serial reaction time task in healthy subjects, providing further evidence for the reliability of the serial reaction time task as a probe of striatal function (7). However, it should be noted that while striatal activation is believed to contribute to implicit learning, activation of this brain area in the context of the serial reaction time task could also be related to processing speed, as responses were faster to the implicit learning versus the random condition.
According to DSM-IV, specific phobia and OCD are categorized as anxiety disorders. This is primarily based on some shared phenomenological attributes of these disorders (e.g., symptoms of anxiety). However, developing a neurobiological framework for understanding psychiatric disorders is essential for advancing specific preventive and treatment strategies. The current findings of similar striatal function in subjects with specific phobia and healthy comparison subjects, in the context of our previous OCD studies demonstrating a striatal deficit relative to health comparison subjects, suggest that OCD and specific phobia may have distinct pathophysiological mechanisms, despite sharing some phenomenological similarities. In fact, several previous neuroimaging studies converge to implicate sensory and paralimbic regions in specific phobia. For example, Wik, Fredrikson, and colleagues (19–21) demonstrated significant changes in regional cerebral blood flow (rCBF) within the secondary visual cortex as well as in the prefrontal posterior cingulate and the anterior temporal-polar cortices when subjects with snake and spider phobia were exposed to phobic stimuli. In a separate PET study that also used symptom provocation in subjects with small animal phobia, we (22) demonstrated significant rCBF changes in anterior paralimbic territories (including the insular cortex), the left somatosensory cortex, and the left thalamus. The sensory cortical findings in these studies were interpreted in the context of the modality in which the phobic stimuli were presented (i.e., visual or somatosensory).
Limitations of this study include the modest number of subjects, especially in light of negative between-group findings. Nonetheless, these data indicate a minimal between-group effect size, which is noteworthy given the large between-group effects found in analogous neuroimaging serial reaction time task studies of subjects with OCD versus healthy comparison subjects. Both prior neuroimaging studies that used the serial reaction time task to compare striatal activation on subjects with OCD versus healthy comparison subjects found significant group differences with comparable group sizes (eight to 14 subjects) (6, 7). In addition to future studies with larger numbers of subjects, direct comparisons between matched groups of subjects with OCD and specific phobia, as well as cohorts with other psychiatric diagnoses, would be important to clarify diagnostic specificity. Conversely, to the extent that cortical-striatal dysfunction has been hypothesized as a core feature of so-called obsessive-compulsive spectrum disorders (e.g., Tourette’s syndrome; see reference 7), these same methods could be used to assess the generalizability of striatal recruitment deficiencies across subjects with those diagnoses. In this regard, with neuroimaging and the serial reaction time task, initial experiments also have observed abnormal activation patterns in subjects with schizophrenia (23) as well as Tourette’s syndrome (7), although the reported abnormalities in those disorders differ from those seen in OCD.
Finally, given that prior neuroimaging studies of subjects with specific phobia have most consistently implicated the involvement of paralimbic and sensory cortical regions (19–22), neuroimaging studies employing specially designed probes to assess function within those systems are indicated to further elucidate the pathophysiology of specific phobia. In conclusion, the current findings do not support striatal dysfunction in subjects with specific phobia, in contrast to previous findings regarding subjects with OCD.
Received Nov. 1, 2002; revision received Feb. 21, 2003; accepted March 3, 2003. From Massachusetts General Hospital, Harvard Medical School; the Department of Psychiatry, University of Illinois at Chicago, Chicago; and Tufts University, Medford, Mass. Address reprint requests to Dr. Rauch, Department of Psychiatry, Massachusetts General Hospital—East, Bldg. 149, Rm. 9130, 13th St., Charlestown, MA 02129; [email protected] (e-mail). Supported by NIMH grant MH-60219 (to Dr. Rauch). Dr. Martis is funded by the Department of Psychiatry, University of Illinois at Chicago; Dr. Wright by NIMH grant MH-64806 and the Robert Wood Johnson Foundation; and Dr. Shin by the National Alliance for Research on Schizophrenia and Depression. The authors thank Larry White and Mary Foley for technical support and Doug Greve for advice regarding data analysis.
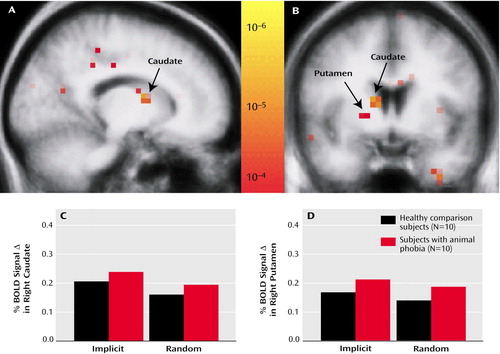
Figure 1. Striatal Activation in Subjects With Animal Phobia and Normal Comparison Subjects During Implicit Sequence Learninga
aThe uppermost images depict a statistical map from all subjects superimposed upon sagittal (A) and coronal (B) group-averaged T1 structured images. Significant signal changes during functional magnetic resonance imaging in the right caudate and putamen are indicated. The bar graphs show the percent of blood-oxygen-level-dependent (BOLD) signal change versus the baseline fixation in the caudate (C) and putamen (D). Although a main effect of condition was present, there was no significant difference between the groups.
1. Grafton ST, Hazeltine E, Ivry R: Functional mapping of sequence learning in normal humans. J Cogn Neurosci 1995; 7:497–510Crossref, Medline, Google Scholar
2. Rauch SL, Savage CR, Brown HD, Curran T, Alpert NM, Kendrick A, Fischman AJ, Kosslyn SM: A PET investigation of implicit and explicit sequence learning. Hum Brain Mapp 1995, 3:271–286Google Scholar
3. Doyon J, Owen AM, Petrides M, Sziklas V, Evans AC: Functional anatomy of visuomotor skill learning in human subjects examined with positron emission tomography. Eur J Neurosci 1996; 8:637–648Crossref, Medline, Google Scholar
4. Nissen MJ, Bullemer P: Attentional requirements of learning: evidence from performance measures. Cogn Psychol 1987; 19:1–32Crossref, Google Scholar
5. Rauch SL, Whalen PJ, Savage CR, Curran T, Kendrick A, Brown HD, Bush G, Breiter HC, Rosen BR: Striatal recruitment during an implicit sequence learning task as measured by functional magnetic resonance imaging. Hum Brain Mapp 1997; 5:124–132Crossref, Medline, Google Scholar
6. Rauch SL, Savage CR, Alpert NM, Dougherty D, Kendrick A, Curran T, Brown HD, Manzo P, Fischman AJ, Jenike MA: Probing striatal function in obsessive-compulsive disorder: a PET study of implicit sequence learning. J Neuropsychiatry Clin Neurosci 1997; 9:568–573Crossref, Medline, Google Scholar
7. Rauch SL, Whalen PJ, Curran T, Shin LM, Coffey BJ, Savage CR, McInerney S, Baer L, Jenike MA: Probing striato-thalamic function in obsessive-compulsive disorder and Tourette syndrome using neuroimaging methods, in Tourette Syndrome. Edited by Cohen DJ, Goetz CG, Jankovic J. Philadelphia, Lippincott Williams & Wilkins, 2001, pp 207–224Google Scholar
8. Oldfield RC: The assessment and analysis of handedness: the Edinburgh Inventory. Neuropsychologia 1971; 9:97–113Crossref, Medline, Google Scholar
9. Cohen J: Statistical Power Analysis for the Behavioral Sciences. Hillsdale, NJ, Lawrence Erlbaum, 1988Google Scholar
10. Beck AT, Ward CH, Mendelson M, Mock J, Erbaugh J: An inventory for measuring depression. Arch Gen Psychiatry 1961; 4:561–571Crossref, Medline, Google Scholar
11. Beck AT, Steer RA: Beck Anxiety Inventory Manual. San Antonio, Tex, Psychological Corporation, 1990Google Scholar
12. Cox RW: AFNI: software for analysis and visualization of functional magnetic resonance neuroimages. Comput Biomed Res 1996; 29:162–173Crossref, Medline, Google Scholar
13. Cox RW, Jesmanowicz A: Real-time 3D image registration for functional MRI. Magn Reson Med 1999; 42:1014–1018Crossref, Medline, Google Scholar
14. Talairach J, Tournoux P: Co-Planar Stereotaxic Atlas of the Human Brain: Three-Dimensional Proportional System. New York, Thieme Medical, 1988Google Scholar
15. Collins DL, Neelin P, Peters TM, Evans AC: Automatic 3D intersubject registration of MR volumetric data in standardized Talairach space. J Comput Assist Tomogr 1994; 18:192–205Crossref, Medline, Google Scholar
16. Filipek PA, Richelme C, Kennedy DN, Caviness VS Jr: The young adult human brain: an MRI-based morphometric analysis. Cereb Cortex 1994; 4:344–360Crossref, Medline, Google Scholar
17. Fyer AJ: Current approaches to etiology and pathophysiology of specific phobia. Biol Psychiatry 1998; 44:1295–1304Crossref, Medline, Google Scholar
18. Martis B, Malizia A, Rauch SL: Functional neuroanatomy of anxiety disorders, in Biological Psychiatry, vol 2. Edited by D’Haenen H, den Boer JA, Willner P. Chichester, UK, John Wiley & Sons, 2002, pp 989–1002Google Scholar
19. Wik G, Fredrikson M, Ericson K, Eriksson L, Stone-Elander S, Greitz T: A functional cerebral response to frightening visual stimulation. Psychiatry Res 1993; 50:15–24Crossref, Medline, Google Scholar
20. Fredrikson M, Wik G, Greitz T, Eriksson L, Stone-Elander S, Ericson K, Sedvall G: Regional cerebral blood flow during experimental fear. Psychophysiology 1993; 30:126–130Crossref, Medline, Google Scholar
21. Fredrikson M, Wik G, Annas P, Ericson K, Stone-Elander S: Functional neuroanatomy of visually elicited simple phobic fear: additional data and theoretical analysis. Psychophysiology 1995; 32:43–48Crossref, Medline, Google Scholar
22. Rauch SL, Savage CR, Alpert NM, Miguel EC, Baer L, Breiter HC, Fischman AJ, Manzo PA, Moretti C, Jenike MA: A positron emission tomographic study of simple phobic symptom provocation. Arch Gen Psychiatry 1995; 52:20–28Crossref, Medline, Google Scholar
23. Kumari V, Gray JA, Honey GD, Soni W, Bullmore ET, Williams SC, Ng VW, Vythelingum GN, Simmons A, Suckling J, Corr PJ, Sharma T: Procedural learning in schizophrenia: a functional magnetic resonance imaging investigation. Schizophr Res 2002; 57:97–107Crossref, Medline, Google Scholar