Defective Recognition of One’s Own Actions in Patients With Schizophrenia
Abstract
OBJECTIVE: The possibility that delusions of influence could be related to abnormal recognition of one’s own actions was investigated in persons with schizophrenia. METHOD: Schizophrenic patients with (N=6) and without (N=18) delusions of influence were compared with normal subjects (N=29) on an action recognition task. The image of a virtual right hand holding a joystick was presented to the subjects through a mirror so that the image was superimposed on their real hand holding a real joystick. Subjects executed discrete movements in different directions. Angular biases and temporal delays were randomly introduced in some trials, such that the movement of the virtual hand departed from the movement executed by the subjects. After each trial, subjects were asked whether the movement they saw was their own. RESULTS: Compared with normal subjects, both patient groups made significantly more recognition errors in trials with temporal delays. In trials with angular biases, the error rate of patients with delusions of influence significantly differed from that of comparison subjects and from that of patients without delusions of influence. CONCLUSIONS: The findings support the hypothesis that delusions of influence are associated with a quantifiable difficulty in correct self-attribution of actions. This difficulty may be related to a specific impairment of a neural action attribution system.
Among the wide range of manifestations characterizing schizophrenia, some of the positive symptoms have been considered critical for the diagnosis of this disease. According to Schneider (1), first-rank symptoms refer to a state where patients interpret their own thoughts or actions as due to the influence of alien forces or of other people. The fact that these symptoms, when narrowly defined (2), can be considered specific to schizophrenia, together with their relative homogeneity across patients, suggests that first-rank symptoms might reflect the disruption of a mechanism that normally generates consciousness of one’s own actions and thoughts and allows their correct attribution to their author. Thus a study of attribution behavior in schizophrenic patients would shed light on this critical function and increase understanding of the factors responsible for misattribution in the patients.
Previous studies that have examined the monitoring of actions in schizophrenia have found that patients with positive symptoms are impaired in error-correcting tasks when tested in the absence of visual feedback (3, 4) and in a drawing task that uses a joystick or a keyboard (5). However, these results do not clarify whether impaired performance in the absence of visual control is related to an altered representation of the action to be performed or to a defect in the sensorimotor loops normally used to control movement execution.
The hypothesis of altered representation is supported by clinical experience with patients who have delusions of influence. These patients tend to deny being the author of the decisions to perform their own actions, even if they have actually performed these actions, suggesting that they cannot match an executed action with the corresponding representation or intention. This hypothesis can be directly tested by studying patients’ ability to distinguish between actions that they have performed and actions performed by others. In a pioneering study (6), normal subjects were presented with movements of an uncertain origin through a procedure in which the image of an actor’s hand was visually superimposed on (and indistinguishable from) the subjects’ own hands. Movements performed by the actor’s hand could be either in concordance or in discordance with the subjects’ own movements. Even in the case of discordant movements, subjects experienced the actor’s hand as their own despite obvious discrepancies between self-generated movements and the movements they saw. In cases of discordant movements, they reported feelings of strangeness or impressions of having their hand pulled by some external force.
Daprati et al. (7) used a new version of this paradigm in a comparison of schizophrenic patients and normal subjects. In that study, subjects performed simple manual gestures with their right hand, which they monitored with a video camera. At times, the image of the subject’s hand was replaced by the image of an investigator’s hand performing the same or a different gesture. At the end of each trial, subjects gave a verbal judgment about whether the hand they had seen was their own hand or another hand. In the most “difficult” trials, in which the subjects saw another hand performing the same movement they had performed, normal subjects misjudged the alien hand as theirs in about 30% of the cases. The error rate was 80% in patients with delusions of influence and 57% in patients without delusions of influence.
These studies demonstrated that clinical difficulty in identifying the origin of an action can be experimentally provoked. However, they did not identify the cues that allowed the comparison subjects to give correct attribution responses and that were missed by the patients with delusions of influence. The study described here was designed to answer this question. In a study design similar to that used by Daprati et al. (7), a realistic virtual hand, instead of the hand of an investigator, was superimposed on the subject’s hand. This device allowed more standardized study conditions, including systematic distortion of the movements of the virtual hand with respect to those of the subjects’ hand. The results fully support the existence of impaired attributions of action in schizophrenia, especially in patients with delusions of influence.
Method
Subjects
Twenty-nine patients with schizophrenia (23 men and six women; mean age=34.6 years, SD=11.0) and 29 normal subjects (20 men and nine women; mean age=36.7 years, SD=11.5) participated in the study. Patients and comparison subjects were naive about the purpose of the study procedure. This study was approved by the local ethics committee (Comité Consultatif de Protection des Personnes se Prétant à la Recherche Biomédicale, Centre Léon Bérard, Lyon). After a complete description of the study procedures, all participants provided written consent.
Patients who were selected for the study met the DSM-IV criteria for schizophrenia. None of the patients met criteria for additional diagnoses. Comparison subjects were recruited from the maintenance staff of two hospitals. Exclusion criteria in both groups were visual and auditory disorders, history of neurological illness or trauma, alcohol and drug dependence according to the DSM-IV criteria, and age older than 65 years or younger than 18 years. The patient and comparison groups did not differ significantly in age, sex, laterality, and educational level.
Seventeen of the 29 patients were hospitalized at the time of the study. Twelve patients met the criteria for the paranoid type of schizophrenia, three for the disorganized type, 11 for the undifferentiated type, and three for the residual type. All but three patients were right-handed, according to assessments made with the Edinburgh Inventory (8). The mean disease duration was 11.3 years (SD=9.0, range=1–33). All patients were treated with antipsychotic medication (principally risperidone, olanzapine, clozapine, and levomepromazine) and were clinically stable at the time of testing. We could find no information in the literature on possible effects of medications on tasks similar to those used in the present work.
Neuropsychological tests, including the Birmingham Object Recognition Battery (9) and Raven’s Coloured Progressive Matrices (10), were used to assess patients’ spatial perception abilities and intellectual performance. The Birmingham Object Recognition Battery assesses the ability to process basic features of simple or geometric pictures. The patients’ mean scores on the six tests from the battery that were used in the study were within the normal range (line match test A score=26.7, compared with the cutoff score of 22 for the lower limit of the normal range; circle match test A score=26.7, compared with the cutoff of 19; line orientation match test A score=24.5, compared with the cutoff of 18; position of gap match test A score=34.7, compared with the cutoff of 24; minimal feature view task score=24.4, compared with the cutoff of 18.5; and item match task score=31.5, compared with the cutoff of 24). The Coloured Progressive Matrices examines the effectiveness of visuospatial reasoning and may detect low intellectual performance. The patients’ mean score on this test (mean=28.4, SD=2.3, range=24–31) was within the normal range for their age group (11).
All patients underwent clinical assessment with the Scale for the Assessment of Positive Symptoms (SAPS) (12) and the Scale for the Assessment of Negative Symptoms (SANS) (13). Mean scores were 24.7 for the SAPS (SD=12.3, range=8–60) and 41.3 for the SANS (SD=19.9, range=6–85). In addition, a subscale for passivity phenomena, consisting of items 15–19 of the SAPS, was defined. This subscale allowed classification of the patients as having or not having delusions of influence. At the time of testing, six patients with a passivity phenomena subscale score greater than 2 (mean=6.3, SD=2.8, range=3–9) were classified as “influenced.” The remaining 23 patients, with scores of 2 or less on this subscale, were classified as “noninfluenced.” There were no differences between the two groups of patients in educational level, intellectual level, clinical features (SAPS and SANS total scores), and scores on five tests from the Birmingham Object Recognition Battery. The mean age of the influenced patients (25.2 years, SD=3.5) was significantly lower than the mean age of the noninfluenced patients (37.1 years, SD=11.0) (t=–2.6, df=27, p<0.02). Performance on the item match task of the Birmingham Object Recognition Battery was significantly lower in influenced patients (mean score=29.7, SD=3.9) than in noninfluenced patients (mean score=31.9, SD=0.3) (t=–2.7, df=27, p<0.02) but was within the normal range.
During the study procedure, five noninfluenced patients were unable to perform the task correctly, and their data were not included in the comparative analysis. The behavior of these five patients during the task will be described in the first section of Results. They did not differ from the other noninfluenced patients in age, illness duration, educational level, intellectual performance, perceptual abilities (Birmingham Object Recognition Battery scores), or total SAPS score. By contrast, their total SANS score was significantly higher (mean=58.2, SD=16.5) than that of the other noninfluenced patients (mean=36.0, SD=18.8) (t=2.38, df=21, p<0.03).
Materials
During the study procedure, the image of an electronically constructed hand was presented to the subjects on a computer screen with a high refresh rate. A specially designed program synthesized pictures of a hand holding a joystick according to the position of a joystick that was actually held by the subject and was connected to the computer. This design allowed the dynamic representation of the movements of the joystick held by the subject with an intrinsic delay <30 msec. Temporal or angular biases could be introduced in this representation (see the section on procedure), modifying the apparent direction or the degree of synchrony of the movement actually performed by the subject with respect to the movement displayed on the computer screen.
The computer screen was placed face down on a metallic support. A horizontal mirror, located 18 centimeters below the screen, reflected the image. The joystick was placed below the mirror on the table supporting the apparatus. The distance between the table and the mirror was 31 cm, so that the subject’s hand holding the joystick was approximately 18 cm below the mirror. Thus, when subjects looked at the mirror, they saw the image of a virtual hand moving a joystick just above their own hand actually moving a joystick.
Procedure
Subjects sat comfortably in front of the apparatus with their forehead leaning on a foam cushion. They held the joystick with the right hand, with the elbow resting on the table. The position of the forearm was adjusted so as to coincide with the direction of the virtual forearm seen in the mirror. Subjects were instructed to maintain their fingers in a fixed position on the joystick and to restrict their movements to the wrist joint.
The task consisted of executing a series of simple movements with the joystick. Each trial started with a dark screen. A green spot (1 cm in diameter) was displayed for 1 second on the left, on the right, or on the top of the screen. The image of the virtual hand then appeared for 2 seconds, during which the subject had to execute a movement of the joystick in the direction indicated by the position of the green spot. Immediately after the trial, subjects had to answer the following question with a yes-or-no response: “Did the movement you saw on the screen exactly correspond to that you have made with your hand?”
Three categories of trials were used: 1) neutral trials, in which the movements of the virtual hand exactly replicated those made by the joystick, 2) trials with angular biases, in which the movements of the virtual hand deviated by a given angular value (5°, 10°, 15°, 20°, 25°, 30°, and 40° either to the right or to the left) with respect to those made by the joystick, and 3) trials with temporal biases, in which the movements of the virtual hand were delayed by a given time (50, 100, 150, 200, 300, 400, and 500 msec) with respect to those made by the joystick. Each trial with a temporal bias was run four times for each of the three directions of movement (left, right, and top of the screen) (N=84); trials with an angular bias were run two times with a bias to the right and two times with a bias to the left for each of the three directions of movement (N=84). Finally, neutral trials were run 12 times. Each subject therefore executed a total of 180 trials. The order of presentation of the 180 trials was randomized before the participation of each subject. Identical trials could not be presented one after the other.
A 5-minute break was provided after 90 trials. Missed trials were repeated if necessary. Before the undergoing the study procedure, each subject had a training session during which the subject was instructed to freely move the joystick. During the first 30 seconds of the training session, no bias was used; then, a 500-msec bias was introduced; and finally a 30° bias was introduced.
Data Analysis
Verbal responses of the subjects were recorded. Depending on whether trials were with or without bias, subjects could potentially make two types of errors: 1) “yes” responses in trials with a bias, and 2) “no” responses in neutral trials. The maximum number of errors was 12 for the neutral trials and 84 for the trials with an angular or a temporal bias. Presentation of the results will focus on the “yes” responses, which reflect the subjects’ ability to recognize a movement as their own.
Nonparametric statistics were used to analyze the data because the scores were not normally distributed. Results for the normal comparison group and two subgroups of patients (influenced and noninfluenced) were compared with the median test and pairwise Mann-Whitney U tests.
Results
Descriptive Analysis
Comparison subjects and patients gave “yes” (correct) responses in nearly all neutral trials. The median value of erroneous “no” responses was equally small (N=1) for all three groups. The distribution of “yes” responses for the biased trials, although clearly different between the groups, as will be discussed in the next section, had a relatively similar pattern across the groups. Among both comparison subjects and patients, the number of “yes” responses was higher for the smaller temporal and angular biases and decreased as the biases increased. In other words, only the slope of the curve differed between groups. This was not the case, however, for five of the 29 patients, who showed no tendency for a decay of “yes” responses in the trials with angular biases, even for the larger values of the biases. Instead, they continued to give “yes” responses at nearly the maximum rate for all biases. These patients, who gave “yes” responses for more than 90% of the trials in the last three classes of angular biases (25°, 30° and 40°), were considered nonresponsive to this experimental variable. For this reason, their data were not included in the comparison analysis. None of these patients had delusions of influence or hallucinations.
Between-Group Analysis
Global differences
Schizophrenic patients with delusions of influence gave globally more “yes” responses than noninfluenced schizophrenic patients and comparison subjects in both the trials with angular biases (median=56.5 for influenced patients; median=39.0 for noninfluenced patients; median=33.0 for comparison subjects) and temporal biases (median=53.5 for influenced patients; median=49.5 for noninfluenced patients; median=29.0 for comparison subjects). The median test for “yes” responses revealed that the differences between groups were significant both for the trials with angular biases (χ2=7.67, df=2, p<0.03) and those with temporal biases (χ2=20.49, df=2, p<0.001). The Mann-Whitney U tests for global response scores revealed that influenced patients produced significantly more errors than comparison subjects both in trials with angular biases (U=19.5, z=–2.95, N=35, p=0.003) and in trials with temporal biases (U=16.5, z=–3.09, N=35, p=0.002), but that noninfluenced patients significantly differed from comparison subjects only in trials with temporal biases (U=73.0, z=–4.11, N=47, p<0.001). The difference approached significance in trials with angular biases (U=178.5, z=–1.80, N=47, p=0.07). However, there was a significant difference between the two groups of patients for the trials with an angular bias (U=23.5, z=2.03, N=24, p<0.05). No correlation was found between patients’ global scores and age, sex, illness duration, and performance on the Birmingham Object Recognition Battery, and no correlation was found between normal subjects’ global scores and age and sex.
Pairwise comparisons
Figure 1 shows that the differences between the comparison group and the two patients groups varied as a function of the amplitude of the biases. In the trials with an angular bias, patients without delusions of influence showed a sharp decrease in erroneous “yes” responses (to 50% of the maximum number of errors) for a bias between 15° and 20°, a pattern not very different from that of the comparison subjects. However, patients with delusions of influence did not reach the same number of “yes” responses until the bias increased to 30°40°. In the trials with a temporal bias, comparison subjects showed a clear decrease in “yes” responses for a relatively small bias (100–150 msec), while both influenced and noninfluenced patients did not show a decrease in the rate of “yes” responses until the bias reached 300 msec.
Pairwise comparisons for each class of trials showed that both groups of patients produced significantly more errors than the comparison group in the trials with a temporal bias longer than 100 msec (Table 1). In the trials with an angular bias, pairwise differences between the influenced patients and the comparison group were significant for biases larger than 10° and pairwise differences between the noninfluenced patients and the comparison group were significant only for biases of 30° and 40° (Table 2).
The two groups of patients differed significantly from each other only for the trials with a 10° bias (Table 2). For most of the other angles, the differences were close to significance. For the trials with a temporal bias, no significant differences between the two groups of patients were observed (Table 1).
Discussion
The present results demonstrate that experimental manipulations of the appearance of one’s own movements impair the correct self-attribution of these movements and that this effect is dramatically larger in patients with schizophrenia.
Comparison subjects still recognize as their own a movement delayed by up to 150 msec with respect to the movement they actually executed. Similarly, if normal subjects see their movement rotated from its actual trajectory by about 15°, they still accept it as their own. These results show that accuracy in detecting the features of one’s own movement is limited and that this limitation is far above perceptual thresholds of the visual system for detecting temporal gaps or angular deviations. The limits reported here for detecting differences between self-produced and externally produced movements rather appear to be those of a specific neural system devoted to perception of biological movements. The existence of such a system is indeed suggested by several psychophysical experiments (14–16).
In the study described here, the patients were clearly worse than comparison subjects at recognizing that movements that were delayed or that deviated from their own movements were distinct from their own movements. Five patients, who were not included in the comparison study, were unable to execute half of the study task; they gave the same response in all trials with angular biases, a pattern of responses that suggested they were unable to “see” this type of bias. This behavior might be explained by the fact that trials with temporal and angular biases were randomized, and these patients might have been unable to consciously monitor the existence of two types of biases. One can only conjecture that they would have behaved differently in an experiment with blocked, instead of randomized, trials. Indeed, these patients had performed correctly in the training session, where the two types of biases were presented separately.
The remaining 24 schizophrenic patients all responded not differently from chance when a time delay up to 300 msec was introduced. For angular deviations, only the patients with delusions of influence responded at chance for deviations up to 30° whereas noninfluenced patients presented an error rate similar to that of comparison subjects, i.e., they became aware of the angle around 15°. Before this lower self-attribution threshold in patients can be related to a specific impairment, however, we have to exclude the possibility that it could be explained by unrelated factors, such as defective perceptual or attentional mechanisms. In the case of angular biases, this possibility seems to be ruled out by the fact that all patients (including the influenced ones) performed well on the Birmingham Object Recognition Battery (9), indicating that they had a normal ability to discriminate small angular differences. In the case of temporal biases, one could argue that schizophrenic patients are known to be slow in many tasks and that their reaction times are globally greater, a feature that can be categorized among the negative symptoms (17).
However, the temporal delay condition in this study was quite different from a reaction time task, and the patients’ impairment was different from slowness to respond. In this study, patients’ impairment was manifested in a difficulty perceiving slight temporal differences. Recent findings about schizophrenic patients’ difficulty in discriminating moving visual stimuli might represent a rationale for the difficulty met by the patients in the present study (18, 19). Although this impairment in resolving temporal delays probably contributed to the high rate of misattributions observed here, it may not represent the core of the problem because it did not differentiate the two subgroups of patients.
In contrast, the deficit in the detection of movement direction might represent a critical factor in misattributing actions. An impairment in trajectory judgments has been reported in schizophrenic patients (20), but the deficit was not related to the clinical symptoms of the patients. The novelty of our finding is that only patients with delusions of influence were impaired in attributing movements with angular biases. An observer’s perception of the direction of a movement is critical information for the observer’s understanding of the agent of this movement; the arm points to the goal of the action, and its direction reveals the intention of the agent. Thus, it is not surprising that a patient deprived of this information will misinterpret the intention displayed by others in their movements and that this misinterpretation will have consequences for the patient’s understanding of interactions between people. The fact that, in the present study, patients with delusions of influence attributed to themselves movements that were different from those they had performed suggests that they could attribute to themselves movements performed by others, hence, the feeling of being influenced by other agents.
An interesting hypothesis (21, 22) proposes that the understanding of an action performed by an external agent could be based on an internal simulation of that action. By placing himself “in the shoes of the agent,” the observer experiences the same feelings and builds a representation of the observed action. This theory is supported by two types of arguments. First, different modalities of action representation have in common a subliminal activation of the motor system, which can be measured in terms of increased excitability of the motor cortex (23–25). Second, functional imaging studies have shown that cerebral activity during imagination, preparation, and observation of a given action involves largely overlapping patterns of activation (26–28). The network common to all of these conditions involves the inferior parietal lobule (Brodmann’s area 40), part of the supplementary motor area and the ventral premotor area. The location and amplitude of cerebral activation produced by the representation of an action thus enables a subject to determine the origin of that action. A functional imagery study in schizophrenic patients with delusions of influence (29) supported this hypothesis.
Because of the small size of the group of patients with delusions of influence in this study and the fact that many individual statistical tests were combined in the final distributions, the results should be interpreted cautiously and confirmed in further experiments. Additional information is also needed about patterns of performance on similar tasks among nonschizophrenic psychiatric patients.
![]() |
![]() |
Received March 9, 2000; revision received Sept. 19, 2000; accepted Sept. 29, 2000. From Institut des Sciences Cognitives, Centre National de la Recherche Scientifique. Address reprint requests to Dr. Franck, Institut des Sciences Cognitives, 67 Boulevard Pinel, 69675 Bron Cedex, France; [email protected] (e-mail). Dr. Franck was supported by a grant from Conseil Scientifique de Recherche, Le Vinatier. The authors thank Hassen Slimani and Carole Etienne for technical support.
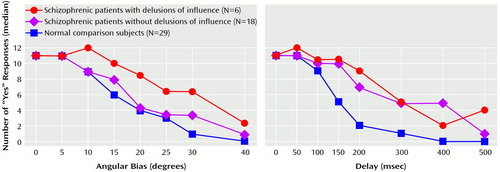
Figure 1. Number of “Yes” Responses by Schizophrenic Patients With and Without Delusions of Influence and Normal Comparison Subjects Asked Whether Hand Movements on a Computer Screen Matched Their Own Hand Movements, by Degree of Angular Bias or Temporal Delay in the Movements on the Screen
1. Schneider K: Klinische Psychopathologie. Stuttgart, Germany, Thieme Verlag, 1955Google Scholar
2. O’Grady JC: The prevalence and diagnostic significance of Schneiderian first-rank symptoms in a random sample of acute psychiatric in-patients. Br J Psychiatry 1990; 156:496–500Crossref, Medline, Google Scholar
3. Malenka RC, Angel RW, Hampton B, Berger PA: Impaired central error-correcting behavior in schizophrenia. Arch Gen Psychiatry 1982; 39:101–107Crossref, Medline, Google Scholar
4. Frith CD, Done DJ: Experiences of alien control in schizophrenia reflect a disorder in the central monitoring of action. Psychol Med 1989; 19:359–363Crossref, Medline, Google Scholar
5. Mlakar J, Jensterle J, Frith CD: Central monitoring deficiency and schizophrenic symptoms. Psychol Med 1994; 24:557–564Crossref, Medline, Google Scholar
6. Nielsen T: Volition: a new experimental approach. Scand J Psychol 1963; 4:225–230Crossref, Google Scholar
7. Daprati E, Franck N, Georgieff N, Proust J, Pacherie E, Dalery J, Jeannerod M: Looking for the agent: an investigation into consciousness of action and self-consciousness in schizophrenic patients. Cognition 1997; 65:71–86Crossref, Medline, Google Scholar
8. Oldfield RC: The assessment and analysis of handedness: the Edinburgh Inventory. Neuropsychologia 1971; 9:97–113Crossref, Medline, Google Scholar
9. Riddoch MJ, Humphreys GW: BORB: Birmingham Object Recognition Battery. Hove, UK, Lawrence Erlbaum Associates, 1993Google Scholar
10. Raven JC: Guide to Using the Coloured Progressive Matrices (1947): Sets A, Ab, B. London, Lewis, 1958Google Scholar
11. Orme JE: Hypothetically true norms for the Progressive Matrices Tests. Hum Dev 1966; 9:222–230Crossref, Medline, Google Scholar
12. Andreasen NC: Scale for the Assessment of Positive Symptoms (SAPS). Iowa City, University of Iowa, 1984Google Scholar
13. Andreasen NC: Scale for the Assessment of Negative Symptoms (SANS). Iowa City, University of Iowa, 1983Google Scholar
14. Shiffrar M, Freyd JJ: Apparent motion in the human body. Psychol Sci 1990; 1:257–264Crossref, Google Scholar
15. Ramachandran VS, Armel C, Foster C, Stoddard R: Object recognition can drive motion perception. Nature 1998; 395:852–853Crossref, Medline, Google Scholar
16. Stevens JA, Fonlupt P, Shiffrar M, Decety J: New aspects of motion perception: selective neural encoding of apparent human movements. Neuroreport 2000; 11:109–115Crossref, Medline, Google Scholar
17. Cadenhead KS, Geyer MA, Butler RW, Perry W, Sprock J, Braff DL: Information processing deficits of schizophrenia patients: relationship to clinical ratings, gender and medication status. Schizophr Res 1997; 28:51–62Crossref, Medline, Google Scholar
18. Chen Y, Palafox GP, Nakayama K, Levy DL, Matthysse S, Holzman PS: Motion perception in schizophrenia. Arch Gen Psychiatry 1999; 56:149–154Crossref, Medline, Google Scholar
19. Schwartz BD, Maron BA, Evans WJ, Winstead DK: High velocity transient visual processing deficits diminish ability of patients with schizophrenia to recognize objects. Neuropsychiatry Neuropsychol Behav Neurol 1999; 12:170–177Medline, Google Scholar
20. O’Donnell BF, Swearer JM, Smith LT, Nestor PG, Shenton ME, McCarley RW: Selective deficits in visual perception and recognition in schizophrenia. Am J Psychiatry 1996; 153:687–692Link, Google Scholar
21. Gallese V, Goldman A: Mirror neurons and the simulation theory of mind reading. Trends Cogn Sci 1998; 2:493–501Crossref, Medline, Google Scholar
22. Jeannerod M: The 25th Bartlett Lecture: to act or not to act: perspectives on the representation of actions. Q J Exp Psychol A 1999; 52:1–29Crossref, Medline, Google Scholar
23. Jeannerod M, Frak V: Mental imaging of motor activity in humans. Curr Opin Neurobiol 1999; 9:735–739Crossref, Medline, Google Scholar
24. Fadiga L, Fogassi L, Pavesi G, Rizzolatti G: Motor facilitation during action observation: a magnetic stimulation study. J Neurophysiol 1995; 73:2608–2611Google Scholar
25. Fadiga L, Buccino G, Craighero L, Fogassi L, Gallese V, Pavesi G: Corticospinal excitability is specifically modulated by motor imagery: a magnetic stimulation study. Neuropsychologia 1999; 37:147–158Crossref, Medline, Google Scholar
26. Rizzolatti G, Fadiga L, Matelli M, Bettinardi V, Paulesu E, Perani D, Fazio F: Localization of grasp representations in humans by PET, 1: observation versus execution. Exp Brain Res 1996; 111:246–252Crossref, Medline, Google Scholar
27. Grafton ST, Arbib MA, Fadiga L, Rizzolatti G: Localization of grasp representations in humans by positron emission tomography, 2: observation compared with imagination. Exp Brain Res 1996; 112:103–111Crossref, Medline, Google Scholar
28. Decety J, Grezes J, Costes N, Perani D, Jeannerod M, Procyk E, Grassi F, Fazio F: Brain activity during observation of actions: influence of action content and subject’s strategy. Brain 1997; 120:1763–1777Google Scholar
29. Spence SA, Brooks DJ, Hirsch SR, Liddle PF, Meehan J, Grasby PM: A PET study of voluntary movement in schizophrenic patients experiencing passivity phenomena (delusions of alien control). Brain 1997; 120:1997–2011Google Scholar