Brain Activation Measured With fMRI During a Mental Arithmetic Task in Schizophrenia and Major Depression
Abstract
OBJECTIVE: The authors used functional magnetic resonance imaging (fMRI) to investigate brain activation in patients with schizophrenia and major depression while they performed two tasks—a vigilance task and a mental arithmetic task—that differed in cognitive complexity. METHOD: In the vigilance task, the participants had to press a response button whenever a specific number was seen on a screen inside the MR scanner. In the mental arithmetic task, the participants had to add two consecutive numbers and press the response button whenever the sum was 10. fMRI was performed with a 1.5-T MR scanner. Twelve patients with recurrent nonpsychotic unipolar major depression, 12 patients with schizophrenia, and 12 healthy comparison subjects were included in the study. RESULTS: Performance data showed that the patients were impaired relative to the comparison subjects and showed no difference in performance between the patient groups. The patients with schizophrenia, but not those with major depression, had less activation in prefrontal brain regions, relative to the comparison participants. However, subtracting brain activation during the vigilance task from activation during the mental arithmetic task showed that the schizophrenia patients had activation in parietal areas. CONCLUSIONS: A double dissociation of parietal and frontal lobe activation was found for the schizophrenia patients and the depression patients. The greater parietal lobe activation in the patients with schizophrenia may reflect a compensatory strategy for the failure to recruit cognitive processes that involve frontal lobe areas when solving a mental arithmetic task.
An intact cognitive apparatus that involves attention, working memory, and executive functions is necessary for performance of mental arithmetic, such as simple addition or subtraction. Despite the importance of the ability to perform simple number calculations in everyday life, this basic cognitive function has not been the target of neurocognitive studies of psychiatric disorders. In the few studies that have investigated arithmetic ability, the tasks performed by subjects either were complex operations similar to those in the WAIS test battery for assessment of general intellectual ability (1, 2) or involved several arithmetic operations in the same study procedure (3). In none of these studies was the correspondence between performance and brain activation studied.
A correspondence between performance and brain activation for mental arithmetic can be inferred from the clinical neuropsychological literature, which has consistently shown that patients with lesions in the inferior parietal lobe fail to perform simple number calculations (4–8). Some of these studies have also pointed to the angular and supramarginal gyri as critically involved brain areas (e.g., references 9–11). Recent studies involving healthy subjects and using hemodynamic neuroimaging methods (functional magnetic resonance imaging [fMRI] and positron emission tomography) have supported the literature on lesions by showing that inferior parietal and prefrontal cortical areas are activated during tasks that involve mental arithmetic (12–17).
We used fMRI to investigate activation in brain areas related to a continuous mental arithmetic task involving simple addition of single digits in patients with schizophrenia, patients with major depression, and healthy comparison subjects. We expected less activation in these brain areas in the patient groups, relative to the comparison subjects. We also expected that the amount of activation in these areas, and particularly in prefrontal areas, in the depressed patients would fall between that in the schizophrenia patients and that in the comparison subjects.
Method
Participants
Twelve patients with recurrent nonpsychotic unipolar major depression, 12 patients with schizophrenia, and 12 healthy comparison subjects were included in the study. The gender distributions were seven women and five men in the depressed group, six women and six men in the schizophrenia group, and seven women and five men in the comparison group. The patients were outpatients or inpatients recruited for research purposes from four psychiatric clinics in the Bergen and Oslo areas in Norway. Data for the comparison participants were previously reported by Landrø et al. (18). The patient groups were part of a larger Norwegian study of neurocognitive deficits in schizophrenia and depression. Neuropsychological data from the larger study have been published elsewhere (19).
Exclusion criteria were alcohol or drug abuse as a primary diagnosis, somatic disorders, recent ECT treatment, the presence of major hearing or vision difficulties, and a diagnosis of schizoaffective disorder. Present or past history of psychiatric disorder was also a reason for exclusion from the comparison group. Potential participants in all groups were excluded if they had a history of neurological disorder.
The depressed patients were taking various types of medication, including SSRIs, mianserin, nefazodone, venlafaxine, and moclobemide. None of the depressed patients was taking a tricyclic antidepressant medication. The schizophrenia patients were taking various types of antipsychotic medication, including both older agents (haloperidol, perphenazine, zuclopenthixol) and newer agents (olanzapine, risperidone).
The study was approved by the Regional Committee for Medical Research Ethics at the University of Bergen. All patients gave their written informed consent after the procedure had been carefully explained and after they had had the opportunity to ask questions about the research.
Clinical Assessments
The initial diagnostic interviews were done by experienced clinical psychiatrists and consisted of collection of relevant clinical and demographic information, including data about current and past medications. The Structured Clinical Interview for DSM-IV Axis I Disorders was used to confirm diagnoses. Severity of depression was measured with the 17-item Hamilton Depression Rating Scale (20) and the 10-item Montgomery-Åsberg Depression Rating Scale (21). To be included in the depression group, participants had to have a minimum score of 18 on the Hamilton depression scale and on the Montgomery-Åsberg Depression Rating Scale, which reflects moderate to severe depression. Ratings of psychiatric symptoms were made with the Brief Psychiatric Rating Scale (BPRS), the Positive and Negative Syndrome Scale (Norwegian translation) (22), and the Global Assessment of Functioning Scale (DSM-IV). Both patient groups were assessed with all scales. Additional clinical characteristics are presented in Table 1.
MRI Scanning
fMRI was performed with a 1.5-T Siemens Vision Plus scanner (Siemens, Erlangen, Germany). Initial scanning of anatomy was done with a T1-weighted three-dimensional fast low-angle shot pulse sequence. Thereafter, serial imaging with 100 blood-oxygen-level-dependent sensitive whole brain measurements was acquired with an echo-planar imaging pulse sequence during each of the two tasks. Thus, a total of 200 volume images were acquired. Each measurement of 4 seconds consisted of 40 axial slices, which constituted an image volume with a measurement interval of 6 seconds (flip angle=50°, acquisition time=4 seconds, TR=6 seconds, TE=84 msec, field of view=230 mm, matrix=64×64). The mean in-plane pixel size was 3.44×3.44 mm, and each slice had a thickness of 3.0 mm, thus creating nearly isotropic voxels. The first 10 volume images for each task were discarded before statistical analyses to avoid initial steady-state problems typically observed with echo-planar imaging sequences.
Study Procedure
There were two runs, corresponding to the two tasks, each with three “on” and three “off” blocks, presented in a boxcar design (Figure 1). The digit stimuli were the numbers “1” through “9.” Forty-eight trials with digit stimuli were presented during each “on” block. Each digit stimulus was presented for 300 msec, with a 2200-msec blank interstimulus interval. Thus, each “on” block lasted 120 seconds and was followed by a 60-second “off” block. The “on” and “off” blocks were alternated within each run. Six target stimulus presentations occurred in each “on” block. Thus, the total number of target stimulus presentations in a run was 18. The total length in time for the echo-planar imaging was about 25 minutes, and scanning of brain anatomy took another 10 minutes. The digit stimuli were presented with Micro Electronic Laboratory (MEL2) software (Psychology Software Tools, Inc., Pittsburgh). The “off” blocks consisted of a baseline period with no stimulus presentations. The participants viewed the digit stimuli by means of electronic goggles consisting of a liquid crystal display screen (Magnetic Resonance Technology, Inc., San Diego, Calif.) that were connected to a computer containing the MEL2 software that was outside the MR chamber. A response button was placed on the participant’s chest, and he/she was instructed to press the button according to the specific instructions for each run. The MEL2 software recorded both response accuracy (correct responses) and response latency (reaction time in milliseconds).
In the first run, the participants were instructed to press the response button whenever they saw the number 7 during each of the three “on” blocks. During the “off” blocks that were interspersed between the “on” blocks, the participants were instructed to simply relax and not to think about the digits seen during the “on” blocks. In the second run, the participants were instructed to add each consecutive number to the previous one, and press the button whenever the sum was 10. The stimulus setup is illustrated in Figure 1.
The first run emphasized visual perception and vigilance, while the second run in addition emphasized number manipulation and simple mental arithmetic. By subtracting activity during the first run from activity during the second run, activations related to mental arithmetic would be obtained when effects of perception and vigilance are subtracted out. The “off” blocks would serve as a within-task baseline condition. By contrasting activation during the second run between the groups, activations related to group differences during mental arithmetic would be obtained.
Image Processing and Statistical Analysis
The data for the different conditions were modeled according to a boxcar stimulus function that was convolved with the hemodynamic response function. Statistical analyses were performed by using contrasts according to the general linear model as implemented in the statistical parametric mapping analysis software package (SPM 99) (London, Wellcome Department of Cognitive Neurology, http://www.fil.ion.ucl.ac.uk) run under MATLAB (Mathworks, Natick, Mass.). The echo-planar images were realigned intraindividually to the first image (11th datapoint) in the first time series, or run, on a voxel-by-voxel basis for each individual, to correct for head movements. The realigned images were then coregistered with the three-dimensional fast low-angle shot anatomy images, transformed into the standardized stereotactic reference system developed by Talairach and Tournoux (23) (Montreal Neurological Institute version), and smoothed with an 8-mm Gaussian kernel. Whole-volume global effects were removed, and the time series data were high-pass filtered to remove artifacts due to cardiorespiratory and other cyclical influences. Areas with statistically significant changes were determined by using the t statistic on a voxelwise basis.
We have chosen to present the higher-order group main effects and the group-by-condition interactions in order to reduce the large amount of data generated in this design. Thus, two different kinds of statistical analyses are reported:
1. For within-group comparisons, the images obtained during the second run (mental arithmetic task) were contrasted with the corresponding images obtained during the first run (vigilance task) according to the following formula: (run 2on–off) – (run 1on–off). The reversed contrast for each group was determined according to the following formula: (run 1on–off) – (run 2on–off). Voxels were identified as significantly activated if they passed an intensity threshold of p<0.05, corrected for multiple comparisons, with a minimum of 10 adjacent voxels to define an activated cluster.
2. For group-by-condition interactions, the images from the within-group reversed contrasts ([run 1on–off] – [run 2on–off]) were compared between the different groups to look for group-by-condition interactions according to the following formula: The interaction analyses were performed by using the two-sample t test included in the SPM 99 package. The two-sample t test was based on a random effects model. Due to the dramatic reduction in the degrees of freedom for this type of analysis, the significance threshold was raised to p<0.01.
Results
Response Accuracy and Response Latency
Response accuracy in the comparison group during both the vigilance task (run 1) and the mental arithmetic task (run 2) was significantly better than the corresponding performances of the schizophrenia group and the depression group (p<0.05, t tests). No significant difference in response accuracy was found between the patient groups. The comparison participants had significantly shorter reaction times during the mental arithmetic task (run 2) than did the patients with schizophrenia but not the patients with depression. No significant difference in reaction time was found between the patient groups. Mean response accuracy scores and response latency times are reported in Table 2. There were no significant correlations between the performance and activation data.
Within-Group Comparisons: Contrasting Runs
Comparison group
Figure 2 shows brain regions with significant activation in the comparison group when activations during the vigilance task were subtracted from activations during the mental arithmetic task ([run 2on–off] – [run 1on–off]). Four clusters passed the p threshold and cluster size cutoff. They were localized in the left and right middle frontal gyri (Brodmann’s area 44/45 and Brodmann’s area 9) and the left and right inferior parietal lobule (Brodmann’s area 40). The Talairach coordinates and t values for maximal activation in these clusters were x=–39, y=18, z=18 (t=6.30) for the medial part of the left middle frontal gyrus; x=45, y=15, z=39 (t=5.35) for the right middle frontal gyrus; x=–39, y=–36, z=39 (t=6.36) for the left inferior parietal lobule; and x=27, y=–51, z=42 (t=5.94) for the right superior parietal lobule.
When activations during the mental arithmetic task were subtracted from activations during the vigilance task ([run 1on–off] – [run 2on–off]), one significant cluster was found in the right transverse temporal gyrus. The coordinates and t value for maximal activation in this cluster were x=60, y=–12, z=12 (t=4.62).
Schizophrenia group
Figure 3 shows brain regions with significant activation in the schizophrenia group when activations during the vigilance task were subtracted from activations during the mental arithmetic task ([run 2on–off] – [run 1on–off]). Two main clusters passed the p threshold and cluster size cutoff: a band of activation from the left to right superior parietal lobule (Brodmann’s area 40/7), including the precuneus, and a relatively smaller cluster in the left middle frontal lobe (Brodmann’s area 9). The coordinates and t values for maximal activation in these clusters were x=–21, y=–72, z=45 (t=6.66) for the left parietal lobule/precuneus; x=24, y=–66, z=48 (t=5.89) for the right superior parietal lobule; and x=–45, y=12, z=27 (t=5.40) for the left inferior frontal gyrus.
When activations during the mental arithmetic task were subtracted from activations during the vigilance task ([run 1on–off] – [run 2on–off]), three significant clusters were found. They were localized in the posterior cingulate and bilaterally in the middle temporal gyrus. The coordinates and t values for maximal activation in these clusters were x=–2, y=–45, z=24 (t=5.19) in the posterior cingulate; x=–51, y=–18, z=–9 (t=4.87) in the left middle temporal gyrus; and x=54, y=–15, z=–18 (t=4.57) in the right middle temporal gyrus.
Depression group
Figure 4 shows brain regions with significant activation in the depression group when activations during the vigilance task were subtracted from activations during the mental arithmetic task ([run 2on–off] – [run 1on–off]). Five significant clusters were found. They were localized in the right inferior and middle frontal gyri (Brodmann’s areas 44 and 47), left inferior and right superior parietal lobule, and left cerebellum. The coordinates and t values for maximal activation in these clusters were x=36, y=24, z=9 (t=3.35) for the right inferior frontal gyrus; x=48, y=15, z=27 (t=3.20) for the right middle frontal gyrus; x=–36, y=42, z=36 (t=3.35) for the left inferior parietal lobule/supramarginal gyrus; x=30, y=–63, z=51 (t=2.74) for the right superior parietal lobule; and x=–33, y=–63, z=–24 (t=6.28) for the left cerebellum.
When activations during the mental arithmetic task were subtracted from activations during the vigilance task ([run 1on–off] – [run 2on–off]), no clusters passed the significance threshold.
Between-Group Comparisons: Group-by-Condition Interactions
The between-group comparisons were based on the images that resulted after activations during the vigilance task were subtracted from activations during the mental arithmetic task ([run 2on–off] – [run 1on–off]). In the patients with schizophrenia, relative to the comparison subjects, significant activations were found bilaterally in the inferior parietal lobe and in the cingulate gyrus. The coordinates and t values for maximal activation in these clusters were x=–55, y=–40, z=44 (t=4.04) for the left inferior parietal lobe; x=44, y=–52, z=44 (t=3.55) for the right inferior parietal lobe; and x=4, y=24, z=40 (t=5.29) in the right cingulate gyrus. In the comparison subjects, relative to the patients with schizophrenia, significant activations were found in the right inferior frontal gyrus (Brodmann’s area 46) and the right supramarginal and lingual gyri. The coordinates and t values for maximal activation in these clusters were x=60, y=4, z=8 (t=3.72) for the right inferior frontal gyrus; x=48, y=–52, z=24 (t=4.40) for the right superior temporal gyrus/supramarginal gyrus; and x=4, y=–44, z=–8 (t=3.91) for the right lingual gyrus.
In the patients with depression, relative to the comparison subjects, significant activation was found bilaterally in the middle frontal gyrus. The coordinates and t value for maximal activation were x=–36, y=28, z=44 (t=4.32). In the comparison subjects, relative to the patients with depression, significant activation was found in the right inferior parietal lobule. The coordinates and t value for maximal activation were x=24, y=20, z=56 (t=3.29).
In the patients with schizophrenia, relative to the patients with depression, significant activation was found bilaterally in the right superior frontal lobe/posterior cingulate. The coordinates and t value for maximal activation were x=4, y=52, z=28 (t=4.40). In the patients with depression, relative to the patients with schizophrenia, significant activation was found bilaterally medially in the left superior frontal lobe. The coordinates and t value for maximal activation were x=–20, y=0, z=56 (t=3.80).
Discussion
A lower level of prefrontal activation is a typical and frequent finding in schizophrenia, particularly in relation to demanding information processing tasks (see references 24 and 25 for reviews). The present findings support these previous results. It should be noted, however, that not all studies have reported low prefrontal activation in patients with schizophrenia. For example, Callicott et al. (26) found greater activation in patients with schizophrenia, relative to healthy comparison subjects. This finding could perhaps have been related to differences in activation between the groups during the baseline period, which was not the case in our study. In contrast to the present findings, prefrontal activation abnormalities have been reported for depression (27, 28). Elliott (29) and Elliott and Dolan (30) reviewed brain imaging data that support a role of the medial prefrontal cortex in relation to dysfunctional modulation of emotional behavior in depression. Other involved regions included the orbitofrontal, infralimbic cortex and the anterior cingulate. It should be noted however that Elliott et al. (31) used a highly demanding executive task (Tower of London) to demonstrate attenuated activation in the lateral prefrontal cortex and anterior cingulate.
The differences in parietal versus frontal lobe activation between the patients with schizophrenia and the patients with depression may be a theoretically significant finding, considering that their accuracy rates and reaction times did not differ. Thus, despite the fact that the two patient groups performed similarly, they achieved similar results by engaging different neuronal activation networks. These findings support the existence of a double dissociation of parietal and frontal lobe activation between schizophrenia and depression. The greater parietal lobe activation seen in the schizophrenia group could be thought of as a compensatory mechanism for the failure to recruit cognitive processes that involve frontal lobe areas. The failure to suppress, or inhibit, activity in the frontal lobes during execution of a cognitive task may be apparent in clinical observations that patients with schizophrenia have problems when they are required to focus on a specific stimulus source and to suppress attention to input from other simultaneous stimuli. In the present study, this clinical characteristic may have been reflected in the patients with schizophrenia in the temporal lobe and posterior cingulate activation during the vigilance task (run 1) that remained after the activation during the mental arithmetic task (run 2) had been subtracted out. Lower activation in the posterior cingulate in the depressed patients may be due to attenuation of a cortical network that relates to mood and affect (30, 31). The differences in brain activation between the patient groups, especially in the schizophrenia group, and the healthy comparison group may alternatively have been due to a deficit related to an inability to internally rehearse the numbers seen on the screen, which would result in reduced prefrontal activation (in Broca’s area). Other studies have found hypoactivation of the parietal lobe in schizophrenia (32). However, this observation was made in studies that used different paradigms and tasks than those used in our study and was related to severity of psychomotor poverty (32), which was not seen in the subjects in our study.
The two patient groups showed opposite patterns of hemisphere activations in the superior frontal areas, with the schizophrenia group showing signs of a left hemisphere deficit, and the depression group showing signs of a right hemisphere deficit. This finding is in agreement with other studies comparing neurocognitive function in patients with schizophrenia and depression, which have found impaired left hemisphere function in schizophrenia (33–37).
Online monitoring of continuously presented stimulus materials would seem especially critical in a mental calculation task, where the participant has to actively keep the last digit in memory and at the same time dispose of the previously presented digit. In our study, the comparison group and the schizophrenia group also activated areas in the supramarginal gyrus and angular gyrus, respectively. These areas have been found to be activated in simple calculations and number processing (9–11). The depressed patients showed greater prefrontal activation, relative to both the patients with schizophrenia and the comparison subjects. The schizophrenia patients appeared to have failed to monitor the ongoing events adequately because of frontal lobe impairment. The greater parietal lobe activation in the schizophrenia patients suggests hyperactivation of this part of the neuronal circuitry in order to keep track of the task to be performed. Thus, a specific deficit, rather than a global nonspecific impairment, was demonstrated. This finding is supported by the performance data, which implied that the patients with schizophrenia were able to perform the task (compare with Price and Friston [38]).
Both patient groups were impaired in both response accuracy and reaction time, relative to the comparison participants. Thus, differences in response accuracy between the patient groups and the comparison group could not be explained as a speed-accuracy tradeoff, which would suggest that the patients had lower accuracy because they did not attempt to perform the tasks. If so, they would also have had shorter reaction times. The differences in brain activation were thus observed despite the fact that both patient groups performed at the same level with regard to the performance measures. This finding points toward dissociation between behavioral performance and activated brain areas for schizophrenia and depression.
The study had some potential limitations that should be considered in interpreting the findings. The effects on cognitive performance of the various medications the patients were taking could have mediated some of the results. However, it is unlikely that this factor alone could explain the differences between the schizophrenia group and the comparison group or between the depression group and the comparison group, since no studies showing a specific effect of medication in enhancing parietal lobule activation in schizophrenia have been reported. A second limitation is that the mental arithmetic task (run 2) could have confounded working memory with the ability to perform mental arithmetic. Working memory is, however, an intrinsic aspect of all cognitive activity that involves the mental manipulation of stimuli across brief periods of time. Nevertheless, in considering everyday use of arithmetic abilities, it is not useful to conceptualize mental arithmetic “free” of working memory. All normal execution of an arithmetic operation involves an aspect of working memory. To force a separation of the two functions in designing a study would, in our view, make the study task substantially different from the everyday action of adding two numbers to get to the sum.
![]() |
![]() |
Presented in part at the 7th Annual Meeting of the Organization for Human Brain Mapping, Brighton, U.K., June 10–14, 2001. Received April 28, 2003; revision received July 9, 2003; accepted July 15, 2003. From the Departments of Biological and Medical Psychology, General Psychology, and Psychiatry, University of Bergen; the Institute of Psychology, University of Oslo, Oslo, Norway; and the Department of Clinical Engineering, Haukeland University Hospital, Bergen, Norway. Address reprint requests to Dr. Hugdahl, Department of Biological and Medical Psychology, University of Bergen, Jonas Lies vei 91, N-5001 Bergen, Norway; [email protected] (e-mail). Supported by grants to Dr. Rund from the Research Council of Norway (number 122974/320) and the National Council for Mental Health Research (number 1997/7) and to Dr. Hugdahl from Haukeland University Hospital (Innovest a/s) and the Research Council of Norway (number 130636/300). The authors thank Roger Barndon, Jarle Iversen, and Susanne Weiss for assistance with the MR scanning, radiological evaluation, and data analysis.
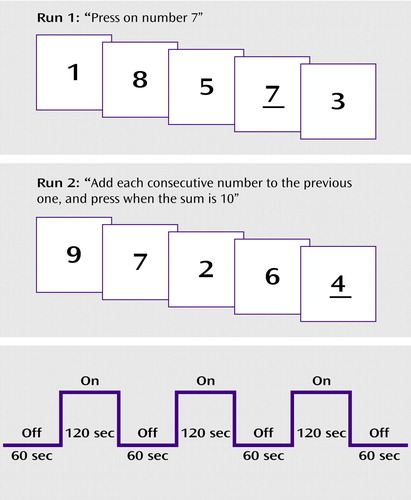
Figure 1. Schematic Outline of the Stimuli Presented in a Vigilance Task (run 1) and a Mental Arithmetic Task (run 2) to Patients With Schizophrenia or Major Depression and Healthy Comparison Subjectsa
aRun 1 tested participants’ visual perception and vigilance. Run 2 tested number manipulation and simple mental arithmetic in addition to visual perception and vigilance. The lower panel shows the time course for the “on” and “off” blocks in the boxcar design for each run. Stimuli were presented during the “on” blocks. During the “off” blocks, the participants were instructed to relax and not to think about the digits seen during the “on” blocks. The first 10 volume images (from the first “off” block) in each run were discarded before data analysis. See Method section for further details.
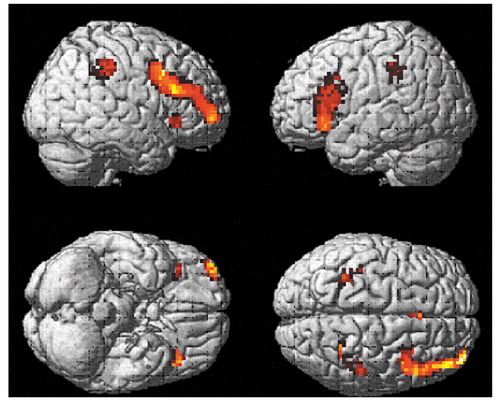
Figure 2. Brain Regions With Significant Activation During Performance of Mental Arithmetic, Relative to Performance of a Visual Perception and Vigilance Task, in Healthy Comparison Subjects (N=12)
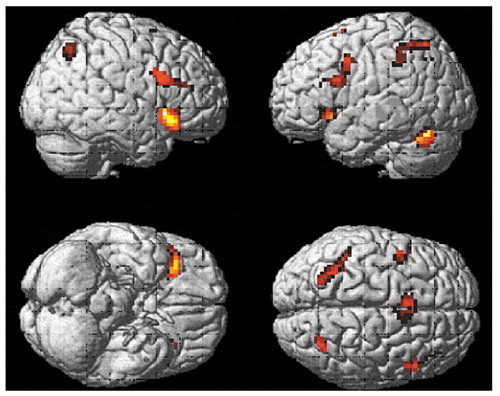
Figure 3. Brain Regions With Significant Activation During Performance of Mental Arithmetic, Relative to Performance of a Visual Perception and Vigilance Task, in Patients With Schizophrenia (N=12)
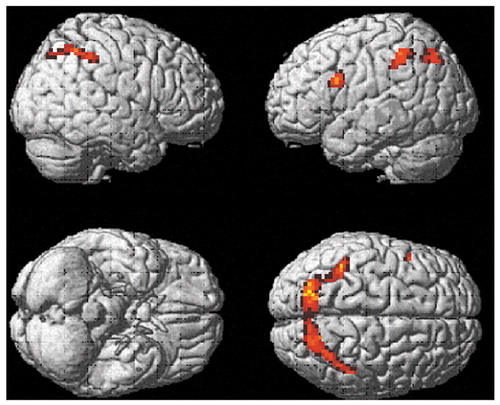
Figure 4. Brain Regions With Significant Activation During Performance of Mental Arithmetic, Relative to Performance of a Visual Perception and Vigilance Task, in Patients With Major Depression (N=12)
1. Kremen WS, Seidman LJ, Faraone SV, Pepple JR, Lyons MJ, Tsuang MT: The “3Rs” and neuropsychological function in schizophrenia: a test of the matching fallacy in biological relatives. Psychiatry Res 1995; 56:135–143Crossref, Medline, Google Scholar
2. Kiefer M, Apel A, Weisbrod M: Arithmetic fact retrieval and working memory in schizophrenia. Schizophr Res 2002; 53:219–227Crossref, Medline, Google Scholar
3. Allen DN, Huegel SG, Seaton BE, Goldstein G, Gurklis JJA, van Kammen DP: Confirmatory factor analysis of the WAIS-R in patients with schizophrenia. Schizophr Res 1998; 34:87–94Crossref, Medline, Google Scholar
4. Ashcraft MH, Yamashita TS, Aram DM: Mathematical performance in left and right brain-lesioned children and adolescents. Brain Cogn 1992; 19:208–252Crossref, Medline, Google Scholar
5. Boller F, Grafman J: Acalculia: historical development and current significance. Brain Cogn 1983; 2:205–223Crossref, Medline, Google Scholar
6. Grafman J, Passafiume D, Faglione P, Boller F: Calculation disturbances in adults with focal hemispheric damage. Cortex 1982; 18:37–50Crossref, Medline, Google Scholar
7. Levin HS, Scheller R, Rickard T, Grafman J, Martinkowski K, Winslow M, Mirvis S: Dyscalculia and dyslexia after right hemisphere injury in infancy. Arch Neurol 1996; 53:88–96Crossref, Medline, Google Scholar
8. Whalen J, McCloskey M, Lesser RP, Gordon B: Localizing arithmetic processes in the brain: evidence from a transient deficit during cortical stimulation. J Cogn Neurosci 1997; 9:409–417Crossref, Medline, Google Scholar
9. Warrington EK, James M, Maciejewski C: The WAIS as a lateralising and localising diagnostic instrument: a study of 656 patients with cerebral lesions. Neuropsychologia 1986; 24:223–239Crossref, Medline, Google Scholar
10. Jackson M, Warrington EK: Arithmetric skills in patients with unilateral cerebral lesions. Cortex 1986; 22:610–622Crossref, Google Scholar
11. Jonides J, Schumacher E, Smith E, Lauber E, Awh E, Minoshima S, Koeppe RA: Verbal working memory load affects regional brain activation as measured by PET. J Cogn Neurosci 1997; 9:462–475Crossref, Medline, Google Scholar
12. Rickard TC, Romero SG, Basso G, Wharton C, Flitman S, Grafman J: The calculating brain: an fMRI study. Neuropsychologia 2000; 38:325–335Crossref, Medline, Google Scholar
13. Chochon F, Cohen L, van der Moortele PF, Dehaene S: Differential contributions of the left and right inferior parietal lobules to number processing. J Cogn Neurosci 1999; 11:617–630Crossref, Medline, Google Scholar
14. Burbaud P, Degreze P, Lafon P, Franconi JM, Bouligand B, Bioulac B, Caille JM, Allard M: Lateralization of prefrontal activation during internal mental calculation: a functional magnetic resonance imaging study. J Neurophysiol 1995; 74:2194–2200Crossref, Medline, Google Scholar
15. Dehaene S, Tzourio N, Frak V, Raynaud L, Cohen L, Mehler J, Mazoyer B: Cerebral activations during number multiplication and comparison: a PET study. Neuropsychologia 1996; 34:1097–1106Crossref, Medline, Google Scholar
16. Rueckert L, Lange N, Partiot A, Appollonio I, Litvan I, LeBihan D, Grafman J: Visualizing cortical activation during mental calculation with functional MRI. Neuroimage 1996; 3:97–103Crossref, Medline, Google Scholar
17. Zago L, Pesenti M, Mellet E, Crivello F, Mazoyer B, Tzourio-Mazoyer N: Neural correlates of simple and complex mental calculation. Neuroimage 2001; 13:314–327Crossref, Medline, Google Scholar
18. Landrø NI, Rund BR, Lund A, Sundet K, Mjellem N, Asbjørnsen A, Thomsen T, Ersland L, Lundervold A, Smievoll AI, Egeland J, Stordal K, Roness A, Sundberg H, Hugdahl K: Honig’s model of working memory and brain activation: an fMRI study. Neuroreport 2001; 12:4047–4054Crossref, Medline, Google Scholar
19. Egeland J, Sundet K, Rund BR, Asbjørnsen A, Hugdahl K, Landrø NI, Lund A, Roness A, Stordal KI: Sensitivity and specificity of memory dysfunction in schizophrenia: a comparison with major depression. J Clin Exp Neuropsychol 2003; 25:79–93Crossref, Medline, Google Scholar
20. Hamilton M: A rating scale for depression. J Neurol Neurosurg Psychiatry 1960; 23:56–62Crossref, Medline, Google Scholar
21. Montgomery SA, Åsberg M: A new depression scale designed to be sensitive to change. Br J Psychiatry 1979; 134:382–389Crossref, Medline, Google Scholar
22. Bentsen H, Munkvold OG, Notland TH, Boye B, Bjørge H, Lersbryggen AB, Oskarsson KH, Berg-Larsen R, Malt UF: The interrater reliability of the Positive and Negative Syndrome Scale (PANSS). Int J Meth Psychiatr Res 1996; 6:227–235Crossref, Google Scholar
23. Talairach J, Tournoux P: Co-Planar Stereotaxic Atlas of the Human Brain: Three-Dimensional Proportional System. New York, Thieme Medical, 1988Google Scholar
24. Blakemore S-J, Frith CD: Functional neuroimaging studies of schizophrenia, in Brain Mapping: The Disorders. Edited by Mazziotta JC, Toga AW, Frackowiak RSJ. San Diego, Academic Press, 2000, pp 523–544Google Scholar
25. Liddle P, Pantelis C: Brain imaging in schizophrenia, in Schizophrenia, 2nd ed. Edited by Hirsch SR, Weinberger D. Oxford, UK, Blackwell Publishers; 2003, pp 403–420Google Scholar
26. Callicott JH, Bertolino A, Mattay VS, Langheim FJP, Duyn J, Coppola R, Goldberg TE, Weinberger DR: Physiological dysfunction of the dorsolateral prefrontal cortex in schizophrenia revisited. Cereb Cortex 2000; 10:1078–1092Crossref, Medline, Google Scholar
27. Dolan RJ, Bench CJ, Brown RG, Scott LC, Frackowiak RSJ: Neuropsychological dysfunction in depression: the relationship to regional cerebral blood flow. Psychol Med 1994; 24:180–182Google Scholar
28. Baker SC, Frith CD, Dolan RJ: The interaction between mood and cognitive function studied with PET. Psychol Med 1997; 27:565–578Crossref, Medline, Google Scholar
29. Elliott R: The neuropsychological profile in unipolar depression. Trends Cogn Sci 1998; 2:447–454Crossref, Medline, Google Scholar
30. Elliott R, Dolan RJ: Functional neuroimaging of depression: a role for medial prefrontal cortex, in Handbook of Affective Sciences. Edited by Davidson RJ, Scherer KR, Hill Goldsmith H. Oxford, UK, Oxford University Press, 2002, pp 117–128Google Scholar
31. Elliott R, Baker SC, Rogers RD, O’Leary DA, Paykel ES, Frith CD, Dolan RJ, Sahakian BJ: Prefrontal dysfunction in depressed patients performing a planning task: a study using positron emission tomography. Psychol Med 1997; 27:931–942Crossref, Medline, Google Scholar
32. Liddle PF, Friston KJ, Frith CD, Hirsch SR, Jones T, Frackowiak RS: Patterns of cerebral blood flow in schizophrenia. Br J Psychiatry 2000; 160:179–186Crossref, Google Scholar
33. Kayser J, Bruder GE, Friedman D: Brain event-related potentials (ERPs) in schizophrenia during a word recognition memory task. Int J Psychophysiol 1999; 34:249–265Crossref, Medline, Google Scholar
34. Crow TJ: Schizophrenia as a failure of the hemispheric dominance for language. Trends Neurosci 1997; 20:339–343Crossref, Medline, Google Scholar
35. Davidson RA, Tomarken AJ: Laterality and emotion: an electrophysiological approach, in Handbook of Neuropsychology. Edited by Grafman J. New York, Elsevier, 1989, pp 419–441Google Scholar
36. Bruder GE: Cerebral laterality and psychopathology: perceptual and event-related potential asymmetries in affective and schizophrenic disorders, in Brain Asymmetry. Edited by Davidson RJ, Hugdahl K. Cambridge, Mass, MIT, 1995, pp 661–692Google Scholar
37. Bruder GE, Towey JP, Stewart JW, Friedman D, Tenke C, Quitkin FM: Event-related potentials in depression: influence of task, stimulus hemifield and clinical features of on P3 latency. Biol Psychiatry 1991; 28:92–98Google Scholar
38. Price CJ, Friston KJ: Scanning patients with tasks that they can perform. Hum Brain Mapp 1999; 8:102–108Crossref, Medline, Google Scholar