PET Study Examining Pharmacokinetics, Detection and Likeability, and Dopamine Transporter Receptor Occupancy of Short- and Long-Acting Oral Methylphenidate
Abstract
OBJECTIVE: The abuse potential of methylphenidate has been related to the drug’s capacity to produce a rapid onset of blockade of the presynaptic dopamine transporter in the brain. An oral once-a-day osmotic controlled-release formulation of methylphenidate produces a more gradual rise in plasma methylphenidate concentration, compared with immediate-release methylphenidate. The authors hypothesized that osmotic-release methylphenidate would also produce a slower onset of blockade of the presynaptic dopamine transporter and would be associated with a lower risk for detection and likeability, compared to immediate-release methylphenidate. METHOD: Twelve healthy adults were randomly assigned to receive single doses of immediate-release methylphenidate or osmotic-release methylphenidate. Doses predicted to produce equivalent maximum concentration (Cmax) values were selected (40 mg of immediate-release methylphenidate and 90 mg of osmotic-release methylphenidate). Plasma d-methylphenidate levels and responses to detection/likeability questionnaire items were obtained hourly for 10 hours after administration of methylphenidate on two separate occasions for each subject. Dopamine transporter receptor occupancies were measured at hours 1, 3, 5, and 7 by using a carbon-11-labeled imaging agent (Altropane) and positron emission tomography. RESULTS: Despite similar Cmax values for both formulations, osmotic-release methylphenidate was associated with a longer time to maximum concentration, longer time to maximum CNS dopamine transporter occupancy, and no detection/likeability, compared with immediate-release methylphenidate. CONCLUSIONS: The findings suggest that the abuse potential of oral methylphenidate is strongly influenced by the rate of delivery and not solely by the magnitude of plasma concentration or brain transporter occupancy. These results advance understanding of the underlying central effects of methylphenidate in humans and identify a potentially less abusable methylphenidate formulation.
The stimulant methylphenidate remains a mainstay of treatment for attention deficit hyperactivity disorder (ADHD), with more than 100 studies documenting its safety and efficacy in pediatric and adult ADHD (1). However, because methylphenidate shares with cocaine the mechanism of action consisting of blockade of the presynaptic dopamine transporter (2), concerns have been raised about the addictive potential of methylphenidate in clinical practice.
Volkow et al. (3) showed a close temporal association between subjective reports of the euphoriant effects of methylphenidate and cocaine, whether administered intravenously or by insufflation, and rapid occupancy of the dopamine transporter in the striatum of the human brain. Although the oral administration of therapeutic doses of methylphenidate is associated with a slower uptake to the dopamine transporter in the brain (4), lingering concerns remain about the potential euphoriant effects of orally administered immediate-release methylphenidate, because it is quickly absorbed and reaches the brain rapidly.
Kollins et al. (5) examined the subjective responses to two doses (20 mg, 40 mg) of long-acting, sustained-release oral methylphenidiate and short-acting, immediate-release oral methylphenidate in healthy volunteers. They found that immediate-release methylphenidate was associated with more robust subjective detection and likeability responses, compared to the sustained-release formulation, and they concluded that the immediate-release formulation has a greater abuse potential than the sustained-release formulation. The findings suggested that the potential euphoriant risk associated with methylphenidate may be moderated by the drug’s oral delivery system. However, because methylphenidate plasma levels were not measured in this study, the investigators were unable to separate the effects of rate of onset from those of the magnitude of the peak plasma concentration (Cmax). Thus, studies are needed in which the peak plasma concentrations of the formulations are matched when subjective responses to different formulations of methylphenidate compounds are being compared.
Because of concerns about the effectiveness of the wax-matrix sustained-release formulation of methylphenidate, oral once-a-day osmotic controlled-release methylphenidate was formulated to provide day-long clinical effects. This compound delivers 22% of the methylphenidate dose immediately and the remaining 78% in an ascending fashion. Pharmacokinetic studies of this compound have documented that plasma methylphenidate levels increase over time with a time to maximum concentration (Tmax) of 6–8 hours and a bi-exponential decline consistent with a long-acting formulation (6). In contrast to equivocal clinical benefits of sustained-release methylphenidate (7), osmotic-release methylphenidate has been shown to be highly effective in placebo-controlled, large-scale, randomized clinical studies in children with ADHD (8). As a consequence, osmotic-release methylphenidate is currently one of the most frequently prescribed methylphenidate formulations in the United States. In addition, in contrast to immediate-release and sustained-release methylphenidate, osmotic-release methylphenidate is difficult to crush and its methylphenidate content cannot be easily extracted, making it a potentially less divertable formulation of methylphenidate.
Since the main target of methylphenidate in the brain is the striatal dopamine transporter (4), measurement of central dopamine transporter binding can elucidate the central kinetic effects of methylphenidate compounds. A highly sensitive method has been developed to measure drug occupancy of the dopamine transporter in the brain by using a carbon-11-labeled radioligand (Altropane, Organix Inc., Boston) to label the dopamine transporter and positron emission tomography (PET) for detection (9). Because the time course of decay of the carbon-11 isotope permits repeated imaging, this method allows examination of the kinetics of CNS dopamine transporter receptor occupancy in the living human brain. Similar PET methods have been previously used to document the CNS pharmacokinetics of other psychiatric drugs (10–14).
The evaluation of whether different formulations of methylphenidate differ in their rate of onset of methylphenidate action (as measured by plasma concentration and dopamine transporter brain occupancy) has a high level of clinical, scientific, and public health relevance. Because the rate of delivery is a key factor previously associated with detection and likeability of methylphenidate (3–5), the identification of a methylphenidate formulation with a more favorable detection and likeability profile could be of great value to clinicians and to the public in selecting the most appropriate methylphenidate treatment for ADHD in clinical practice. Linking plasma kinetics with brain activity of methylphenidate could further understanding of the underlying central effects of methylphenidate in humans.
The main goal of this study was to assess whether the abuse potential of methylphenidate is moderated by differences in the oral delivery system and whether a formulation in which the drug is delivered over a longer time period may be less likely to be abused than an immediate-release formulation. We compared effects of orally administered therapeutic doses of a short-acting, immediate-release formulation of methylphenidate with those of a long-acting, osmotic-release formulation of methylphenidate in the following areas: 1) pharmacokinetic profile of methylphenidate, including the rate of onset of methylphenidate action (indexed by plasma levels), 2) dopamine transporter receptor occupancy in the brain (indexed by PET measurement of radioligand binding), and 3) abuse liability (indexed by subjective ratings of detection and likeability).
To disentangle the effects of rate of onset of methylphenidate action and dopamine transporter receptor occupancy in the brain on levels of detection and likeability, we selected doses of the two formulations of methylphenidate that would result in similar peak plasma concentrations (Cmax) and indirectly measured CNS dopamine transporter receptor occupancy over time. We tested the following hypotheses: 1) osmotic-release methylphenidate would have a longer Tmax than immediate-release methylphenidate; 2) maximum CNS dopamine transporter receptor occupancy would be similar for these doses of immediate-release methylphenidate and osmotic-release methylphenidate, and maximum dopamine transporter occupancy for osmotic-release methylphenidate would be achieved later than that of immediate-release methylphenidate; and 3) the subjective effects of detection and likeability would be greater for immediate-release methylphenidate than for osmotic-release methylphenidate, despite similar Cmax values and maximum dopamine transporter binding potentials for the two formulations.
Method
Subjects
The subjects consisted of 12 Caucasian volunteers between ages 18 and 55 years. Six were men, and six were women. All subjects were right-handed and in good health. Six were given immediate-release methylphenidate, and six were given osmotic-release methylphenidate. The two groups were of similar age (mean=24 years, SD=2.4, versus mean=25 years, SD=3.9; F=0.31, df=1, 10, p=0.59), sex (χ2=1.3, df=1, p=0.25), and socioeconomic status as measured with the Hollingshead Four-Factor Index of Social Status, on which lower values indicate higher socioeconomic status (mean=1.8, SD=0.41, versus mean=1.5, SD=0.55; F=1.43, df=1, 10, p=0.26). Each subject was assessed with a complete medical and psychiatric history and physical examination before imaging. None had any DSM-IV axis I disorders, including ADHD and current or past drug or alcohol abuse. In addition, none had a history of exposure to psychotropic medicines (including stimulants) or tobacco. For female subjects, inquiry about the subject’s current reproductive status was made, and a pregnancy test was conducted. All subjects were assessed with an ECG, full blood count, blood chemistries, and urinalysis.
Procedures
Subjects underwent PET imaging before and after administration of oral doses of immediate-release methylphenidate or osmotic-release methylphenidate. Assignment of medication was randomized by the pharmacy, and the subjects were blind to the medication assignment. As recommended by Kollins et al. (5), we matched the Cmax of the immediate-release methylphenidate and osmotic-release methylphenidate formulations. On the basis of available pharmacokinetic modeling data (unpublished 2004 data, McNeil Consumer & Specialty Pharmaceuticals, Fort Washington, Penn.), we expected that oral therapeutic doses of 40 mg of immediate-release methylphenidate and 90 mg of osmotic-release methylphenidate would achieve comparable Cmax values. Subjects underwent a total of five PET imaging sessions on three different days. On day 1, baseline scanning (one scan) was completed. On day 2, scanning was done at 1 and 3 hours after administration of methylphenidate (two scans). On day 3, scanning was done at 5 and 7 hours after administration of methylphenidate (two scans). On days 2 and 3, venous blood was drawn hourly for quantification of plasma concentration of d-methylphenidate hourly over 10 hours. We measured plasma d-methylphenidate because this enantiomer has been shown to be the active moiety (15). Although none of the methylphenidate metabolites are active (16), we also measured plasma levels of the major metabolite of d-methylphenidate, d-ritalinic acid.
PET Imaging
Images were acquired by using an HR+ PET camera (CTI, Knoxville, Tenn.). The primary imaging parameters of the HR+ camera are in-plane and axial resolution of 4.5 mm full width at half maximum and 63 contiguous slices of 2.5-mm separation. Images were acquired in three-dimensional mode and reconstructed by using an iterative algorithm to an in-plane resolution of 4.5 mm full width at half maximum. Photon attenuation measurements were made with rotating pin sources containing germanium-68.
For each scan, approximately 5 mCi of carbon-11-labeled radioligand was injected intravenously over 30 seconds, and serial PET images were acquired. Dynamic image collection started at the same time as the infusion, and images were acquired in 15-second frames for the first 2 minutes, in 1-minute frames for the next 4 minutes, and in 2-minute frames for the last 54 minutes, for a total of 39 frames over 60 minutes. On the second and third days of scanning, the initial scans were followed by a 2-hour period to allow for decay of residual radioactivity, and then the radioligand injection and imaging procedures were repeated.
All projection data were corrected for nonuniformity of detector response, dead time, random coincidences, and scattered radiation. Regions of interest representing the striatum (the left and right caudate nucleus and the left and right putamen) and cerebellum were drawn manually on PET images. This procedure was repeated for all slices in which the structures were visualized at full intensity (away from edge slices). For each frame, regions of interest of like structures were averaged to yield average striatal and cerebellar time activity curves. We determined that the reference (cerebellum) time activity curves were not affected by the dopamine transporter inhibitor.
The binding potential values (BP, or Bmax/Kd) for the carbon-11-labeled radioligand were calculated by using a kinetic model that compared data from the striatum and cerebellum (17). Dopamine transporter receptor occupancy was calculated by using the following equation: (BP baseline – BP methylphenidate/BP baseline) × 100. To estimate the plasma methylphenidate level required to occupy 50% of the dopamine transporter receptor sites, the percentage of dopamine transporter occupancy (P) was linearized by plotting the logarithm of P divided by (100 – P) versus the logarithm of the plasma level of d-methylphenidate (ng/ml). The linear regression permitted the determination of the plasma d-methylphenidate level associated with 50% occupancy of the dopamine transporter, which, in turn, corresponds to the 0.0 value on the y-axis in a scattergram showing the slope of the relationship between plasma d-methylphenidate concentration and dopamine transporter occupancy.
On the days that subjects received methylphenidate, they completed three items from the Drug Rating Questionnaire, a subjective measure of drug effects that has been used to assess the abuse potential of drugs. One item measured the subject’s level of feeling an effect from the drug. In addition, an item about liking the drug was used to assess the drug’s potential to produce euphoria, and an item about disliking the drug was used to assess the drug’s potential to produce both dysphoria and withdrawal. Subjects rated the items on a scale from 1 to 29, with higher scores indicating a stronger subjective experience. Constituent elements of the scale were standardized by comparison to responses to known drugs of abuse and validated against observer ratings and physiological changes (18). This measure and related scales have been used in numerous published studies assessing the abuse liability of methylphenidate (18–20). Subjects completed the Drug Rating Questionnaire hourly for 10 hours on each of the 2 days they received methylphenidate.
Statistical Analysis
Categorical data were analyzed with chi-square tests, continuous parametric data with unpaired t or F tests, and nonparametric data with the rank sum test. Associations between continuous variables were evaluated by using Pearson’s product-moment correlation. We controlled for multiple comparisons using Holm’s sequential Bonferroni method (21). In computing Holm’s test, we used Nyholt’s method (22) to adjust the total number of tests that were assumed.
This study was approved by the Massachusetts General Hospital Institutional Review Board. After complete description of the study to the subjects, written informed consent was obtained.
Role of the Funding Sources
This study was initiated by the investigators. McNeil Consumer & Specialty Pharmaceuticals provided funding, assisted in study design, and provided comments on the manuscript to the first author (Dr. Spencer) but did not assist in the collection, analysis, or interpretation of data. NIMH grant RO1 MH-064019 (Dr. Spencer) supported the evaluation of dopamine transporter binding in subjects with and without ADHD.
Results
Pharmacokinetic Profile of Methylphenidate
To relate plasma levels of d-methylphenidate to dopamine transporter occupancy, plasma d-methylphenidate was measured hourly after subjects received 90 mg of osmotic-release methylphenidate or 40 mg of immediate-release methylphenidate (Figure 1). As expected, plasma d-methylphenidate levels were higher for the immediate-release formulation than for the osmotic-release formulation at hours 1–3, the levels for the two formulations converged at hour 4, and the levels were lower for immediate-release methylphenidate than for osmotic-release methylphenidate at hours 5–10 (Figure 1). The Cmax for d-methylphenidate was higher for osmotic-release methylphenidate than for immediate-release methylphenidate, although the difference did not reach statistical significance (mean=17.7 ng/ml, SD=4.7, versus mean=14.1, SD=3.7; F=4.2, df=1, 22, p=0.05). Moreover, the Tmax for d-methylphenidate was 3.5 times longer for osmotic-release methylphenidate than for immediate-release methylphenidate (mean=7.5 hours, SD=1.2, versus mean=2.2 hours, SD=0.8; F=170, df=1, 22, p<0.0001). The plasma levels of d-ritalinic acid were highly correlated to d-methylphenidate levels (r=0.83). In addition, the ratio of the d-ritalinic acid Cmax for osmotic-release methylphenidate to that for immediate-release methylphenidate was 1.19, indistinguishable from the 1.18 ratio of the d-methylphenidate Cmax values for the two formulations.
Dopamine Transporter Occupancy in Striatum
d-Methylphenidate occupancy of brain dopamine transporter receptors was measured at 1, 3, 5, and 7 hours after administration of 90 mg of osmotic-release methylphenidate or 40 mg of immediate-release methylphenidate. Figure 2 and Figure 3 show that at 1 hour, dopamine transporter occupancy with immediate-release methylphenidate was greater than that with osmotic-release methylphenidate (F=5.19, df=1, 10, p<0.05). At hours 3 and 5, the occupancy values for the two groups converged, and at 7 hours dopamine transporter occupancy with immediate-release methylphenidate was lower than that with osmotic-release methylphenidate (F=57.01, df=1, 10, p<0.001). Average maximum dopamine transporter occupancy was similar for immediate-release methylphenidate and osmotic-release methylphenidate (mean=71.5, SD=7.7, versus mean=67.6, SD=5.5; F=1.0, df=1, 10, p=0.34). The average time to maximum occupancy was three times longer with osmotic-release methylphenidate than with immediate-release methylphenidate (mean=5 hours, SD=2.5, versus mean=1.7, SD=1.0; F=8.9, df=1, 10, p<0.02).
Dopamine transporter occupancy was significantly correlated with plasma concentration of d-methylphenidate (correlation coefficient=0.7, t=6.6, df=45, p<0.001) (Figure 4, upper panel). In an analysis with log transformation of the dopamine transporter data, similar to the analysis of dopamine transporter findings by Volkow et al. (4), we found that a plasma concentration of 5.7 ng/ml of d-methylphenidate was associated with 50% blockade of dopamine transporter (Figure 4, center panel). Furthermore, the slope of the relationship between plasma d-methylphenidate concentration and dopamine transporter occupancy was significantly greater for the immediate-release formulation than for the osmotic-release formulation, and the interaction of plasma methylphenidate concentration and drug formulation was significant (t=20.4, df=43, p<0.0001) (Figure 4, lower panel).
Detection and Likeability
Compared to the subjects who received the osmotic-release formulation, the subjects who received the immediate-release formulation reported a greater subjective response on all three scales of the Drug Rating Questionnaire across all 10 hours after receiving a methylphenidate dose (“feel an effect”: z=2.6, p<0.01; “like the drug effect”: z=2.5, p<0.05; “dislike the drug effect”: z=2.8, p<0.01). The highest scores for questions 1 (“feel an effect”) and 2 (“like the drug effect)” were observed at 2 hours for both formulations. The highest scores for question 3 (“dislike the drug effect”) were observed at 4 hours for immediate-release methylphenidate group and 2 hours for osmotic-release methylphenidate group (Figure 5). For individual hour comparisons, we used an alpha level of 0.0077 to correspond to an overall adjusted number of subjects of 6.5 for all tests (22). Higher scores were recorded for the immediate-release group than for the osmotic-release group at all times, but only the difference in the ratings at 4 hours survived statistical correction for multiple comparisons for all three scales (z>2.8, p<0.006). It is noteworthy that the osmotic-release group had a negligible subjective response to the drug after hour 4, although this group had maximum plasma concentration of d-methylphenidate at 7.5 hours after receiving the drug and maximum dopamine transporter occupancy at 5 hours.
Discussion
This study examined the peripheral and central pharmacokinetic properties of two formulations of methylphenidate with different oral delivery systems and the effect of these properties on the abuse potential of the two formulations. Unlike immediate-release methylphenidate, osmotic-release methylphenidate was designed to release d-methylphenidate in escalating amounts throughout the day. Although similar plasma concentrations of the two formulations were reached, osmotic-release methylphenidate required a longer period of time to reach maximum plasma concentration and maximum occupancy of the dopamine transporter in the brain and engendered no detection/likeability interoceptive (subjective) effects, compared to immediate-release methylphenidate. These results support the hypotheses that peripheral and brain pharmacokinetic profiles and the level of detection/likeability of methylphenidate can be accurately predicted on the basis of the delivery system profile of the formulation. They also suggest that the osmotic controlled-release delivery system may diminish the abuse potential of methylphenidate.
Because we selected doses that produced similar peak plasma concentrations in the two formulations of methylphenidate we evaluated, we were able to disentangle the spectrum of pharmacokinetics, dopamine transporter occupancy, and detection/likeability effects that were due to the rate of delivery from those that were due to the magnitude of the d-methylphenidate concentration. This is an important methodological feature of this study.
The similarity in maximum plasma d-methylphenidate concentrations observed for the 40-mg dose of immediate-release methylphenidate and the 90-mg dose of osmotic-release methylphenidate is consistent with the results of previous pharmacokinetic modeling for both formulations (unpublished 2004 data, McNeil Consumer & Specialty Pharmaceuticals, Fort Washington, Penn.). Our results showing that plasma d-methylphenidate concentrations peaked earlier in subjects who received immediate-release methylphenidate and later in subjects who received osmotic-release methylphenidate are also consistent with the predicted pharmacokinetic profiles for an immediate-release and a long-acting formulation.
The results for dopamine transporter occupancy paralleled the results for the Cmax and Tmax pharmacokinetic properties of both formulations. Although maximum dopamine transporter occupancies were similar for immediate-release methylphenidate and osmotic-release methylphenidate, the average time to maximum dopamine transporter occupancy was approximately three times longer for osmotic-release methylphenidate than for immediate-release methylphenidate. This difference was consistent with the effects of a long-acting formulation.
It is noteworthy that our dopamine transporter occupancy findings are highly consistent with those reported by Volkow et al. (4) for comparable doses of oral immediate-release methylphenidate, despite methodological differences between studies in the timing of the first measurement (1 hour versus 2 hours after oral administration of methylphenidate) and radioligand used (carbon-11-labeled Altropane versus carbon-11-labeled cocaine). Because a 90-mg dose of osmotic-release methylphenidate releases approximately 20 mg of methylphenidate immediately, our 1-hour results with 90 mg of osmotic-release methylphenidate and 40 mg of immediate-release methylphenidate (Figure 2) closely approximate the results of Volkow et al. (4) for occupancies of 20 mg of immediate-release methylphenidate (54%) and 40 mg of immediate-release methylphenidate (72%). The 24% difference in dopamine transporter occupancy at 1 hour between immediate-release methylphenidate and osmotic-release methylphenidate in our study is almost identical to the 25% difference at 2 hours reported by Volkow et al. for comparable doses of methylphenidate. It is remarkable that the plasma concentration associated with 50% blockade of the dopamine transporter was nearly identical to that reported by Volkow et al. (∼6.0 ng/ml in both studies).
Although plasma concentrations predicted dopamine transporter occupancy with both formulations of methylphenidate, there was a stronger correlation between plasma d-methylphenidate levels and dopamine transporter occupancy with the immediate-release formulation than with the osmotic-release formulation. The reasons for this finding are unclear, but it is possible that the plasma kinetics of two formulations produce different physiological outcomes due to differences in rates of transport across the blood-brain barrier and the effects of CNS kinetics with dopamine transporter binding itself. More work is needed to confirm this finding and elucidate its meaning.
As reviewed by Kollins et al. (5), the rate of rise of a drug’s plasma concentration has been thought to indicate abuse liability in studies of cocaine (23, 24), alprazolam (25), diazepam (26), and pentobarbital (27), as well as methylphenidate (4, 5). In our study, subjects who received immediate-release methylphenidate reported a greater subjective response on all three detection/likeability Drug Rating Questionnaire scales, compared to subjects who received osmotic-release methylphenidate. These results are consistent with those of the only other study that compared immediate-release methylphenidate with a first-generation long-acting methylphenidate formulation (a sustained-release formulation) (5). In that study, the investigators demonstrated a greater subjective response to immediate-release methylphenidate than to sustained-release methylphenidate by using doses of methylphenidate that were similar to those used in our study (20 mg and 40 mg). In both studies, the subjects who received immediate-release methylphenidate reported greater rates of subjective awareness of medication effects soon after receiving the drug (within 4 hours). In our study at 4 hours, the plasma level of immediate-release methylphenidate was descending and that of osmotic-release methylphenidate was rising. It is not surprising that the immediate-release group’s dislike of the drug would be greater at 4 hours (due to rebound effects), but it is noteworthy that liking was also greater at 4 hours. Kollins et al. (5) also found that liking initiated by immediate-release methylphenidate persisted into the phase of descending plasma levels. In both studies, subjects reported little response from either formulation in the later hours, despite rising or high plasma levels with the long-acting formulations. These findings suggest that the slow rise in plasma level with the osmotic-release formulation is associated with little liking or disliking, regardless of Cmax values.
Our results showing that the subjective response to methylphenidate was moderated by the kinetics of the methylphenidate formulation and not simply by the plasma concentration or dopamine transporter occupancy at a single point in time are consistent with those of Volkow et al. (3), who reported a strong association between dopamine transporter occupancy and subjective response to intravenous methylphenidate. Taken together, these results support the hypothesis that the velocity of plasma and CNS uptake of a drug is more critical than its concentration for detection/likeability risk, regardless of the mode of delivery.
Our finding that the majority of subjects who received immediate-release methylphenidate reported subjective detection/likeability effects is consistent with previous research. Subjective drug experience, especially euphoria, has been thought to be an indicator of risk of abuse (18, 19). In their review of the literature, Kollins et al. (19) found that in 18 (72%) of 25 studies in which detection/likeability was evaluated, subjective responses to oral immediate-release methylphenidate were reported. However, our detection/likeability results for oral immediate-release methylphenidate differed from those of Volkow et al. (4). In that study, in which doses ranged from 10 to 60 mg, only one subject reported detection/likeability effects while receiving immediate-release methylphenidate. Although these differences could be due to methodological issues, more work is needed to understand the variance in findings between these studies.
Although the maximum plasma concentration and dopamine transporter occupancy at 7 hours with osmotic-release methylphenidate were the same as those associated with the high ratings of detection/likeability at 1 hour in subjects who received immediate-release methylphenidate, osmotic-release methylphenidate was not associated with detection/likeability (Figure 5). These findings suggest that the slow ramp-up of methylphenidate levels in the osmotic-release formulation exerts a protective effect against a subsequent subjective response of detection/likeability regardless of peak plasma levels and dopamine transporter occupancy.
Our results should be viewed in light of some methodological limitations. One limitation is the small number of subjects. In addition, the dose of immediate-release methylphenidate (40 mg) was chosen as part of the experimental design. Typically, lower single doses are used clinically. For female subjects, we did not determine the phase of the menstrual cycle, which may be a source of variability in dopamine transporter occupancy (28). Because we did not do magnetic resonance imaging (MRI) scans, we could not rule out pathological processes in the brain. However, pathological processes are unlikely in healthy subjects. Although regions of interest can be manually drawn by using MRI data, salient structures (such as the striatum and cerebellum) can be clearly outlined in PET scans with the radioligand that we used (Altropane). The regions of interest were chosen to be well inside the structure boundaries to avoid partial volume effects. The same regions of interest were used for each subsequent scan of each individual subject. Moreover, our data on dopamine transporter occupancies with immediate-release methylphenidate matched those of Volkow et al. (4).
Although the difference did not reach statistical significance, the peak plasma concentration of d-methylphenidate was 23% higher for osmotic-release methylphenidate than for immediate-release methylphenidate. However, this difference would be expected to bias the study findings toward greater dopamine transporter occupancy and stronger subjective feelings in subjects who received osmotic-release methylphenidate, contrary to our hypothesis. Although the parameters of detection and likeability are thought to be proxies of abuse potential, the closeness of this association has been controversial (19). For example, some detection may result from peripheral and not CNS effects and may not index euphoriant risk. Similarly, the sensation of liking a drug could occur in the context of setting and expectation and may not index risk of euphoria. These considerations suggest that the actual abuse potential of both methylphenidate formulations used in our study may be less than we estimated. The results of this study do not prove that osmotic-release methylphenidate is not abusable but suggest that it may be less abusable than immediate-release methylphenidate. In addition, our study addresses the risk of abuse to achieve euphoria and not the risk of abuse for purposes of weight loss or extended wakefulness.
Despite these considerations, our results show that peripheral and brain pharmacokinetic profiles and the detection/likeability of methylphenidate can be accurately predicted on the basis of the delivery system profile of the formulation. The findings also indicate that the abuse potential of methylphenidate is due to the rate of delivery and not the magnitude of plasma concentration or receptor occupancy in the brain. These results advance understanding of the underlying central effects of methylphenidate in humans and identify a potentially less abusable methylphenidate formulation.
Presented as a poster at the 43rd annual meeting of the American College of Neuropharmacology, Dec. 12–16, 2004, San Juan, P.R. Received Feb. 9, 2005; revision received June 23, 2005; accepted Nov. 7, 2005. From the Pediatric Psychopharmacology Unit, Psychiatry Service, Massachusetts General Hospital; the Department of Psychiatry, Harvard Medical School, Boston; McNeil Consumer & Specialty Pharmaceuticals, Fort Washington, Penn.; the Division of Neurochemistry, New England Primate Research Center, Boston; and the Department of Nuclear Medicine, Massachusetts General Hospital and Harvard Medical School, Boston. Address correspondence and reprint requests to Dr. Spencer, Pediatric Psychopharmacology Unit (ACC-725), Massachusetts General Hospital, YAW 6900, 55 Fruit St., Boston, MA 02114; [email protected] (e-mail).This study was supported by funding from NIMH grant RO1 MH-064019 (Dr. Spencer) and McNeil Consumer & Specialty Pharmaceuticals.
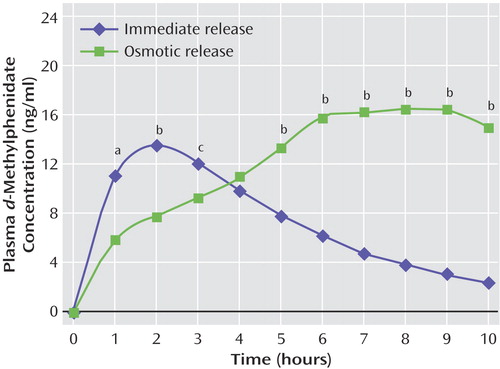
Figure 1. Mean Plasma d-Methylphenidate Concentrations in Healthy Subjects After Receipt of a Single Dose of Immediate-Release (N=6) or Osmotic-Release (N=6) Methylphenidate
aSignificant difference between groups (F=8.62, df=1, 22, p<0.01).
bSignificant difference between groups (F=16.3–198, df=1, 20–22, p<0.001).
cSignificant difference between groups (F=6.27, df=1, 22, p<0.05).
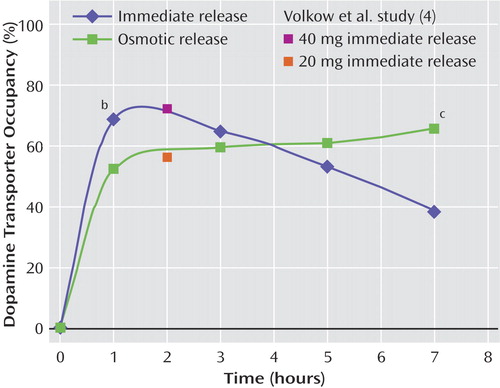
Figure 2. Mean Striatal Dopamine Transporter Receptor Occupancy in Healthy Subjects After Receipt of a Single Dose of Immediate-Release (N=6) or Osmotic-Release (N=6) Methylphenidatea
aMean dopamine transporter occupancy values obtained in healthy subjects 2 hours after receipt of 40 mg and 20 mg of immediate-release methylphenidate in a study by Volkow et al. (4) are shown for comparison.
bSignificant difference between groups (F=5.19, df=1, 10, p<0.05).
cSignificant difference between groups (F=57.01, df=1, 10, p<0.001).
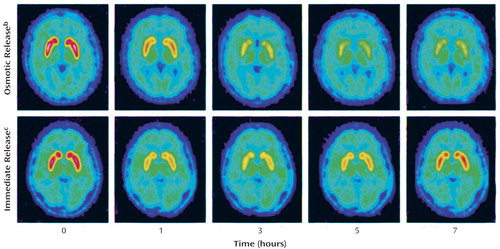
Figure 3. Serial PET Brain Images Showing Striatal Dopamine Transporter Receptor Occupancy After Receipt of a Single Dose of Immediate-Release or Osmotic-Release Methylphenidate in Two Healthy Subjectsa
aDopamine transporter receptor occupancy in the striatum was assessed by measuring binding of a carbon-11-labeled imaging agent (Altropane).
bPlasma d-methylphenidate concentrations in this subject were 5.8 ng/ml at 1 hour, 9.2 ng/ml at 3 hours, 13.3 ng/ml at 5 hours, and 16.1 ng/ml at 7 hours.
cPlasma d-methylphenidate concentrations in this subject were 11.1 ng/ml at 1 hour,12.2 ng/ml at 3 hours, 7.9 ng/ml at 5 hours, and 4.8 ng/ml at 7 hours.
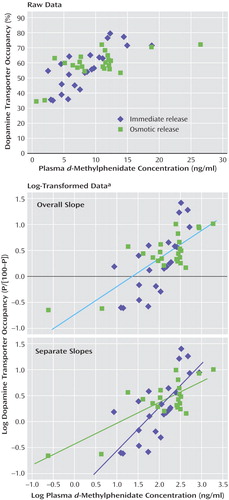
Figure 4. Correlation of Dopamine Transporter Receptor Occupancy and Plasma d-Methylphenidate Concentration in Healthy Subjects After Receipt of a Single Dose of Immediate-Release (N=6) or Osmotic-Release (N=6) Methylphenidate
aDopamine transporter occupancy was determined for each subject at hours 1, 3, 5, and 7 after methylphenidate administration. The number of correlations for each condition was ~23 because of missing data. The log-transformed data were derived by using the following equation: P/(100-P), where P is the percentage of dopamine transporter occupancy. The linear regression analysis of these data permitted the determination of the plasma d-methylphenidate concentration required for 50% occupancy of the dopamine transporter receptor sites (concentration=5.7 ng/ml, which corresponds to the 0.0 value on the y-axis in the center panel).
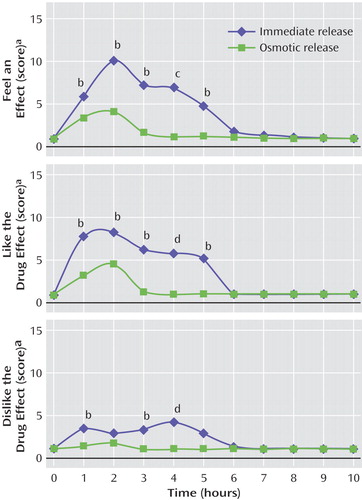
Figure 5. Mean Scores of Healthy Subjects on Three Items of the Drug Rating Questionnaire After Receipt of a Single Dose of Immediate-Release (N=6) or Osmotic-Release (N=6) Methylphenidate
aRated on a scale from 1 to 29, with higher scores indicating a stronger subjective experience.
bSignificant difference between groups (p<0.05, rank sum test, z=2.0–2.4).
cSignificant difference between groups (p<0.01, rank sum test, z=2.8).
dSignificant difference between groups (p<0.005, rank sum test, z=3.0).
1. Multimodal Treatment Study of Children With ADHD (MTA) Cooperative Group: A 14-month randomized clinical trial of treatment strategies for attention-deficit/hyperactivity disorder. Arch Gen Psychiatry 1999; 56:1073–1086Crossref, Medline, Google Scholar
2. Volkow ND, Wang GJ, Fowler JS, Logan J, Franceschi D, Maynard L, Ding YS, Gatley SJ, Gifford A, Zhu W, Swanson JM: Relationship between blockade of dopamine transporters by oral methylphenidate and the increases in extracellular dopamine: therapeutic implications. Synapse 2002; 43:181–187Crossref, Medline, Google Scholar
3. Volkow N, Wang G, Fischman M, Foltin R, Fowler J, Abumrad N, Vitkun S, Logan J, Gatley S, Pappas N, Hitzemann R, Shea C: Relationship between subjective effects of cocaine and dopamine transporter occupancy. Nature 1997; 386:827–830Crossref, Medline, Google Scholar
4. Volkow ND, Wang G-J, Fowler JS, Gatley SJ, Logan J, Ding Y-S, Hitzemann R, Pappas N: Dopamine transporter occupancies in the human brain induced by therapeutic doses of oral methylphenidate. Am J Psychiatry 1998; 155:1325–1331Link, Google Scholar
5. Kollins SH, Rush CR, Pazzaglia PJ, Ali JA: Comparison of acute behavioral effects of sustained-release and immediate-release methylphenidate. Exp Clin Psychopharmacol 1998; 6:367–374Crossref, Medline, Google Scholar
6. Modi NB, Lindemulder B, Gupta SK: Single- and multiple-dose pharmacokinetics of an oral once-a-day osmotic controlled-release OROS (methylphenidate HCl) formulation. J Clin Pharmacol 2000; 40:379–388Crossref, Medline, Google Scholar
7. Greenhill L, Halperin J, Abikoff H: Stimulant medications. J Am Acad Child Adolesc Psychiatry 1998; 38:503–512Crossref, Google Scholar
8. Wolraich ML, Greenhill LL, Pelham W, Swanson J, Wilens T, Palumbo D, Atkins M, McBurnett K, Bukstein O, Aug G: Randomized, controlled trial of OROS methylphenidate once a day in children with attention-deficit/hyperactivity disorder. Pediatrics 2001; 108:883–892Crossref, Medline, Google Scholar
9. Fischman AJ, Bonab AA, Babich JW, Livni E, Alpert NM, Meltzer PC, Madras BK: [(11)C, (127)I]Altropane: a highly selective ligand for PET imaging of dopamine transporter sites. Synapse 2001; 39:332–342Crossref, Medline, Google Scholar
10. Fischman AJ, Bonab AA, Babich JW, Alpert NM, Rauch SL, Elmaleh DR, Shoup TM, Williams SA, Rubin RH: Positron emission tomographic analysis of central 5-hydroxytryptamine2 receptor occupancy in healthy volunteers treated with the novel antipsychotic agent, ziprasidone. J Pharmacol Exp Ther 1996; 279:939–947Medline, Google Scholar
11. Fischman AJ, Alpert NM, Rubin RH: Pharmacokinetic imaging: a noninvasive method for determining drug distribution and action. Clin Pharmacokinet 2002; 41:581–602Crossref, Medline, Google Scholar
12. Fischman AJ, Alpert NM, Babich JW, Rubin RH: The role of positron emission tomography in pharmacokinetic analysis. Drug Metab Rev 1997; 29:923–956Crossref, Medline, Google Scholar
13. Salazar DE, Fischman AJ: Central nervous system pharmacokinetics of psychiatric drugs. J Clin Pharmacol 1999; Aug suppl:10S-12SGoogle Scholar
14. Christian BT, Livni E, Babich JW, Alpert NM, Dischino DD, Ruediger E, Salazar DE, Ford NF, Fischman AJ: Evaluation of cerebral pharmacokinetics of the novel antidepressant drug, BMS-181101, by positron emission tomography. J Pharmacol Exp Ther 1996; 279:325–331Medline, Google Scholar
15. Ding YS, Fowler JS, Volkow ND, Dewey SL, Wang GJ, Logan J, Gatley SJ, Pappas N: Chiral drugs: comparison of the pharmacokinetics of [11C]d-threo and l-threo-methylphenidate in the human and baboon brain. Psychopharmacology (Berl) 1997; 131:71–78Crossref, Medline, Google Scholar
16. Patrick SK, Mueller RA, Gualtieri CT, Breese GR: Pharmacokinetics and actions of methylphenidate, in Psychopharmacology: The Third Generation of Progress. Edited by Meltzer HY. New York, Raven Press, 1987, pp 1387-1396Google Scholar
17. Bonab AA, Fischman AJ, Alpert NM: Comparison of 4 methods for quantification of dopamine transporters by SPECT with [123I]IACFT. J Nucl Med 2000; 41:1086–1092Medline, Google Scholar
18. Jasinski DR, Henningfield JE: Human abuse liability assessment by measurement of subjective and physiological effects. NIDA Res Monogr 1989; 92:73–100Medline, Google Scholar
19. Kollins SH, MacDonald EK, Rush CR: Assessing the abuse potential of methylphenidate in nonhuman and human subjects: a review. Pharmacol Biochem Behav 2001; 68:611–627Crossref, Medline, Google Scholar
20. Jasinski DR: An evaluation of the abuse potential of modafinil using methylphenidate as a reference. J Psychopharmacol 2000; 14:53–60Crossref, Medline, Google Scholar
21. Holm S: A simple sequentially rejective multiple test procedure. Scandinavian Journal of Statistics 1979; 6:65–70Google Scholar
22. Nyholt DR: A simple correction for multiple testing for single-nucleotide polymorphisms in linkage disequilibrium with each other. Am J Hum Genet 2004; 74:765–769Crossref, Medline, Google Scholar
23. Cone EJ: Pharmacokinetics and pharmacodynamics of cocaine. J Anal Toxicol 1995; 19:459–478Crossref, Medline, Google Scholar
24. Resnick RB, Kestenbaum RS, Schwarz LK: Acute systemic effects of cocaine in man: a controlled study by intranasal and intravenous routes. Science 1977; 195:696–698Crossref, Medline, Google Scholar
25. Mumford GK, Evans SM, Fleishaker JC, Griffiths RR: Alprazolam absorption kinetics affects abuse liability. Clin Pharmacol Ther 1995; 57:356–365Crossref, Medline, Google Scholar
26. de Wit H, Dudish S, Ambre J: Subjective and behavioral effects of diazepam depend on its rate of onset. Psychopharmacology (Berl) 1993; 112:324–330Crossref, Medline, Google Scholar
27. de Wit H, Bodker B, Ambre J: Rate of increase of plasma drug level influences subjective response in humans. Psychopharmacology (Berl) 1992; 107:352–358Crossref, Medline, Google Scholar
28. Lammers CH, D’Souza U, Qin ZH, Lee SH, Yajima S, Mouradian MM: Regulation of striatal dopamine receptors by estrogen. Synapse 1999; 34:222–227Crossref, Medline, Google Scholar