Does Fast Dissociation From the Dopamine D2 Receptor Explain the Action of Atypical Antipsychotics?: A New Hypothesis
Abstract
OBJECTIVE: Although atypical antipsychotics are becoming the treatment of choice for schizophrenia, what makes an antipsychotic “atypical” is not clear. This article provides a new hypothesis about the mechanism of action of atypical antipsychotics. METHOD: Published data regarding the molecular, animal model, neuroimaging, and clinical aspects of typical and atypical antipsychotics were reviewed to develop this hypothesis. Particular attention was paid to data regarding the role of the serotonin 5-HT2 and dopamine D4 receptors in atypicality. RESULTS: Neuroimaging data show that optimal dopamine D2 occupancy is sufficient to produce the atypical antipsychotic effect. Freedom from motor side effects results from low D2 occupancy, not from high 5-HT2 occupancy. If D2 occupancy is excessive, atypicality is lost even in the presence of high 5-HT2 occupancy. Animal data show that a rapid dissociation from the D2 receptor at a molecular level produces the atypical antipsychotic effect. In vitro data show that the single most powerful predictor of atypicality for the current generation of atypical antipsychotics is fast dissociation from the D2 receptor, not its high affinity at 5-HT2, D4, or another receptor. CONCLUSIONS: The authors propose that fast dissociation from the D2 receptor makes an antipsychotic more accommodating of physiological dopamine transmission, permitting an antipsychotic effect without motor side effects, prolactin elevation, or secondary negative symptoms. In contrast to the multireceptor hypotheses, the authors predict that the atypical antipsychotic effect can be produced by appropriate modulation of the D2 receptor alone; the blockade of other receptors is neither necessary nor sufficient.
All antipsychotics block dopamine D2 receptors, but some dissociate from the receptors more quickly than others.
This Orwellian phrase captures the essence of our hypothesis. We propose that a faster dissociation from the D2 receptor, without reference to any other receptor system, explains the most pertinent aspect of what is currently called “atypical” antipsychotic activity. We present our thoughts in five sections. In the first, we define “atypicality” and briefly review some technical concepts regarding receptor pharmacology relevant to our discussion. Next, we review positron emission tomography (PET) studies in patients and derive from them the centrality of D2 occupancy in generating antipsychotic response. The third section reviews data showing how previous hypotheses regarding the role of serotonin 5-HT2 and dopamine D4 were actually driven by underlying low affinity and fast dissociation from the D2 receptor. In the fourth and fifth sections we explain how fast dissociation of a drug from the D2 receptor, at molecular and system levels, leads to the atypical antipsychotic effect. Finally, we point out the limitations of our current hypothesis, how it relates to the role of other receptors, and the implications of this hypothesis for future drug development.
Effect of Atypical Antipsychotics and Fast Dissociation
Atypical Antipsychotics
The use of the term “atypical” is linked inextricably to clozapine (1); often one uses “atypical” as a way of saying “clozapine-like.” A review of several previous definitions and the use of this term (2–9) shows that most authors agree that no or low extrapyramidal side effects and the ability to avoid sustained hyperprolactinemia are central to most definitions. Beyond these two criteria there is no consensus. Since there is almost universal consensus in the use of “atypical” to describe the newer antipsychotics (risperidone, olanzapine, sertindole, and quetiapine), one can derive the definition empirically by asking what really are the differences between these newer drugs and typical antipsychotics. These newer drugs have been extensively tested, and data for more than 7,000 patients have been published and meta-analyzed (10). The biggest difference between the typical and atypical antipsychotics is regarding extrapyramidal side effects; every atypical antipsychotic meets this criterion. The effect size of this difference is 0.30 (this should be interpreted as 30 percentage points more extrapyramidal side effects with typical than atypical antipsychotics) (10). The second biggest difference is in lack of prolactin elevation; three of these four antipsychotics (except risperidone) clearly meet this criterion (11). Beyond that, the differences between typical and atypical antipsychotics are small in magnitude and unreliable. When data from all the newer atypicals are combined, there is no group advantage in the effect on negative symptoms (10). The two atypicals that do show an advantage (risperidone and olanzapine) show an average effect size of 0.06. Even this relatively small difference is observed only when treatment with optimal doses of these drugs are compared to administration of 10–20 mg/day of haloperidol, a comparison that is biased against haloperidol in terms of extrapyramidal side effects and secondary negative symptoms. To our knowledge, the only study (12) that used lower doses of haloperidol in comparison found no difference in the improvement of negative symptoms (10). Furthermore, most patients in these studies had a history of partial response or poor compliance with typical agents to start, thus biasing the studies against the typical antipsychotics in terms of efficacy. Even in this set of patients the superiority of atypical agents in terms of additional improvement in positive symptoms was minimal (average effect size: 0.01).
Thus, low or no extrapyramidal side effects and lack of sustained prolactin elevation are the essential features of atypicality. Although the atypicals’ effects on negative symptoms and refractory psychosis are certainly desirable, they are not as yet reliably generated by all atypical antipsychotics.
Drug-Receptor Interaction
The common parameter used in discussing drug action at a receptor is its affinity: some drugs have a very high affinity for the D2 receptor (e.g., haloperidol); others show a low affinity for the dopamine receptor (e.g., clozapine). Affinity (or, more accurately, Kd) is usually measured by mixing receptor-bearing tissue with varying concentrations of an antipsychotic in a test tube. After 2 hours, when the reaction has reached equilibrium, affinity is measured as the concentration of the drug required to occupy 50% of the receptors. Although affinity measures are useful indicators of the equilibrium state, the dynamic human neurotransmission does not hold steady for 2 hours to allow drug-receptor interactions to reach equilibrium. The dynamic process of the drug-receptor interaction is better captured by more basic parameters: association and dissociation rates.
The binding of an antipsychotic to a receptor is a dynamic process with continuous association and dissociation and can be represented as follows (13, 14):
As captured by this equation, a drug (D) binds to a receptor (R) at a rate that is regulated by the “on” rate constant, kon (unit concentration–1 time–1). The rate at which the drug receptor (DR) complex dissociates is determined by the “off” rate constant, koff (unit time–1). Kd, the usual measure of affinity, is only a ratio of koff/kon. Thus, affinity is dependent on rate constants, not the other way around. Rate constants allow one to predict how the drug receptor system responds to dynamic changes and, therefore, are more relevant parameters for understanding drug action in living systems. We will show shortly that low affinity for the D2 receptor is driven entirely by a fast dissociation from the D2 receptor and is a necessary and sufficient feature of the action of atypical antipsychotics.
Clinical PET Data Regarding Antipsychotics
PET Studies of D2 Occupancy
In an important 1992 article, Farde et al. (15) examined a number of patients who were taking different antipsychotics and suggested that the threshold for clinical response and extrapyramidal side effects could be separated in terms of different D2 occupancy. This notion of differentiable thresholds was supported by Nordstrom et al. (16), who treated patients with different levels of D2 occupancy, as produced with raclopride, a selective D2/3 antagonist, and found a lower threshold for response than for extrapyramidal side effects. In a recent study (17), we randomly assigned patients to 38%–87% D2 occupancy with haloperidol and found that D2 occupancy significantly predicted response at a threshold of 65%. None of the patients with occupancy below 78% exhibited any extrapyramidal side effects, whereas four of the five with occupancy above 78% showed extrapyramidal side effects. In addition we observed that patients with D2 occupancies below 72% experienced minimal effects on prolactin levels, whereas patients with D2 occupancy higher than 72% had significant prolactin elevation, a relationship confirmed in another study group (18). Thus, even with a relatively typical antipsychotic such as haloperidol, it is possible, although not clinically feasible, to obtain an antipsychotic effect without extrapyramidal side effects just by optimizing D2 occupancy. A similar relationship between high D2 occupancy and extrapyramidal side effects has also been observed in studies using cross-sectional single photon emission computed tomography (19–21).
The newer atypical antipsychotics risperidone and olanzapine achieve robust antipsychotic activity only at doses that occupy 65% or more of D2 receptors, which is similar to the action of haloperidol (22–26). On the other hand, although clozapine and quetiapine show less than 60% D2 occupancy when levels are measured 12 hours after administration of the drug (22, 23, 26), these differences may partially be due to a fast decline in D2 occupancy. Recent studies focusing on the course of D2 occupancy in patients receiving quetiapine found that although patients receiving 300–600 mg/day showed less than 20% D2 occupancy at 12 hours after receiving a dose, at 2 hours they showed occupancies in the 60% range (23, 27). Similarly, a subject receiving clozapine (350 mg/day) showed reasonably high (71%) D2 occupancy 1–2 hours after dose administration; this declined to 55% at 12 hours and to 26% at 24 hours (28). Thus, all antipsychotics, typical or atypical, block a relevant number of D2 receptors, although they may differ in the kinetics of occupancy and the relationship of peak to trough occupancy.
Atypical Antipsychotics and 5-HT2A Occupancy
Although many current atypical antipsychotics (risperidone, olanzapine, clozapine, quetiapine, and ziprasidone) show significantly greater 5-HT2A than D2 occupancy (29–32), 5-HT2A occupancy may not be a necessary condition for atypicality. First, typical antipsychotics such as loxapine (33) and chlorpromazine (34) show equally high 5-HT2A occupancy. Second, it should be noted that atypical antipsychotics produce high 5-HT2A occupancy at doses that are not antipsychotic (e.g., <2 mg/day of risperidone, <5 mg/day olanzapine, and 50 mg/day of clozapine) (30) (Figure 1). Third, these atypical antipsychotics become effective only at doses at which their D2 occupancy exceeds 65%, a threshold of efficacy no different from that of haloperidol, which suggests a continuing importance of D2 (Figure 1). Thus, it would seem that 5-HT2A blockade is neither necessary (since typical antipsychotics achieve response without 5-HT2A blockade) nor sufficient for antipsychotic response (22, 23). This observation is buttressed by recent reports that show that fananserin (36) and MDL-100907 (37), both with high 5-HT2A occupancy but devoid of D2 occupancy, failed to work as antipsychotics.
Does the presence of 5-HT2 occupancy exert an attenuating effect on the D2-extrapyramidal side effects relationship? Although there are indirect animal data suggesting this is the case (38–41), there are no good clinical data addressing this question. Most of the current large-scale clinical trials have used high doses of typical antipsychotics (i.e., 10–20 mg/day of haloperidol, which give rise to greater than 90% D2 occupancy) and compared them to doses of atypical antipsychotics that give rise to less than 80% D2 occupancy. Since the mismatch in D2 occupancy alone can explain the differences in extrapyramidal side effects and prolactin effects between typical and atypical antipsychotics (30, 35), it is unclear whether the superiority of atypicals in these domains should be accorded to their 5-HT2 blockade or to a more appropriate dosing regarding their D2 blockade. Antipsychotics, typical or atypical, give rise to extrapyramidal side effects only when they exceed 78%–80% D2 occupancy; when they do so, concomitant 5-HT2A blockade does not offer immunity from these (33, 35). Since clozapine and quetiapine never exceed this threshold of D2 occupancy, they never give rise to extrapyramidal side effects. Since olanzapine and risperidone exceed this threshold in a dose-dependent fashion, they give rise to extrapyramidal side effects also in a dose-dependent fashion (30).
The Molecular Basis of Atypicality
To our knowledge, all efforts to produce antipsychotic action without D2 blockade have been unsuccessful. Notable recent failures include drugs that act only on 5-HT2 (MDL-100907) (37), D4 (L-745,850) (42), 5-HT2 and D4 (fananserin) (36), and dopamine D1 receptors (SCH-23390) (43). Thus, currently, D2 blockade remains a necessary and sufficient condition for antipsychotic action. Since all antipsychotics block D2 receptors, it has often been thought that atypical antipsychotics must differ by involving a separate receptor mechanism. Two hypotheses, one invoking the role of the 5-HT2 receptor and another proposing a role for the D4 receptor, have been particularly influential.
In an important article that supported the 5-HT2/D2 hypothesis, Meltzer et al. (44) studied the in vitro binding profile of 37 antipsychotics (some were clinically proven; others were preclinical at that time). This report constitutes the cornerstone of the serotonin-dopamine hypothesis of atypicality. It showed that atypicals had a greater difference between their 5-HT2 and D2 affinities than did typicals. However, what is often overlooked is that the greater difference between 5-HT2 and D2 affinities in atypicals was due not to higher 5-HT2 affinity but to lower D2 affinity. For the 20 typicals and 17 atypicals in that report, the mean affinity at the 5-HT2 receptors (expressed as pKi) was 8.37 (SD=0.16) (for typicals) and 8.36 (SD=0.25) (for atypicals); there was no statistical difference between the two. On the other hand, their D2 mean affinities were 8.88 (SD=0.14) (for typicals) and 7.02 (SD=0.25) (for atypicals); the difference was highly significant (F=43.5, df=1, 35, p<0.001). Thus, atypical antipsychotics were shown to differ from typicals by their lower affinity at the D2 receptor, not by their higher affinity at 5-HT2. The same pattern of results was found in in vivo measures of affinity in animal models (41). A discriminant function analysis in the article showed that a low D2 affinity was by far the single biggest contributor to atypicality and that the contribution of high 5-HT2 affinity was clearly secondary (44).
For the sake of argument, one could suggest that even though low D2 affinity drives atypicality, the presence of high 5-HT2 affinity is still necessary (the 5-HT2/D2 ratio argument [44]). Several lines of evidence refute this possibility. First, atypical antipsychotics such as amisulpride (available in Europe) (34, 45, 46) and remoxipride (withdrawn from clinical use because of association with aplastic anemia) (47) provide atypical clinical benefits with no relevant affinity for the 5-HT2 receptor. Amisulpride is particularly interesting since it has been shown to be as effective as haloperidol in the treatment of positive symptoms; it has fewer extrapyramidal side effects (46) and more effectiveness in treating negative symptoms (48, 49), thus matching the clinical profile of multireceptor atypicals such as risperidone and olanzapine but without any effects at other receptors. Second, the 5-HT2/D2 ratio argument is not compatible with human PET data. Subtherapeutic doses of olanzapine (<5 mg/day) and risperidone (<2 mg/day) have high 5-HT2 versus D2 occupancy ratios; however, these doses are not even antipsychotic, let alone atypical. Thus, high 5-HT2 occupancy seems neither necessary nor sufficient for the atypical antipsychotic effect.
Antagonism at the D4 receptor has also been mentioned as a basis for atypicality. However, when this hypothesis was considered in the context of other typical and atypical drugs, it also suffered from same problems as the 5-HT2/D2-ratio hypothesis. The affinity of typical drugs such as haloperidol and chlorpromazine for the D4 receptor is actually higher than that of drugs such as clozapine or olanzapine (50). Thus, typical antipsychotics are more potent than atypicals, not less potent, at the D4 receptor. In light of this, a D4 basis of atypicality cannot be sustained unless one links it to D2 and then argues for a high D4/D2 ratio (51). The D4/D2 ratio argument (like the argument for the 5-HT2/D2 ratio) also falls short of evidence. First, the differences in the ratio are driven by differences in D2, not by differences in D4, since haloperidol is more potent than clozapine at the D4 receptor (50). Second, drugs with practically no affinity for the D4 receptor, for example, quetiapine and amisulpride (50–52), are atypical antipsychotics. Finally, clinical trials of drugs selective for the D4 receptor (42) or those that combine 5-HT2 and D4 selectivity (36) do not show evidence of antipsychotic efficacy. Thus, high D4 affinity is neither necessary nor sufficient for producing atypical antipsychotic activity.
We propose that a low affinity at the D2 receptor in and of itself, without reference to any other receptor profile, is sufficient for producing atypical antipsychotic activity. Previous hypotheses regarding a role for the 5-HT2/D2 and D4/D2 ratios seemed valid because they shared a common denominator: a low affinity for D2. The current generation of atypical antipsychotics are atypical not because they have high levels of 5-HT2 or D4 activity but because they have a low affinity at the D2 receptor. That raises the question, why should a low affinity at the D2 receptor give rise to atypicality?
Affinity (more precisely, Kd) is, by definition, the ratio of koff/kon (the rate at which the drug moves off of and on to the receptor). In theory, either a difference in kon or a difference in koff could lead to low affinity. To examine whether kon or koff drives the differences in D2 affinity between typical and atypical antipsychotics; we measured the affinity, kon and koff, for a series of typical and atypical antipsychotics (53). Although affinity for the D2 receptor varied nearly a thousand-fold, from 0.025 nM for nemonapride to 155 nM for quetiapine, 99% of the difference in affinity of the antipsychotics was driven by differences in their koff at the D2 receptor. Differences in kon did not account for any significant differences in affinity (53). All antipsychotics (typical or atypical) attach to the D2 receptor with a similar rate constant; they differ only in how fast they come off of the receptor. We propose that this relationship between fast koff and low affinity is the critical underlying molecular feature that explains why low affinity at the D2 receptor leads to the atypical antipsychotic effect.
In test tube experiments, dissociation of an antipsychotic from the receptor is determined mainly by the molecular properties of the drug. In the living brain, this molecular property of fast dissociation displays itself on a stage of fluctuating plasma/brain levels of the drug. For example, clozapine, even at steady-state levels, shows a peak of 850 ng/ml and a trough of 300 ng/ml daily. Thus, in a living system the dissociation of a drug from a receptor is determined by phenomena at two levels: events at a molecular level in the synapse (determined mainly by koff) and events at a system level (which is a complex function of plasma half-life, brain half-life, and koff). We will distinguish between these two levels of fast dissociation (one molecular and one systemic) and point out the relevance of each to atypicality.
Implications of Fast Dissociation at the Molecular Level
In living systems, antipsychotics compete with endogenous dopamine. It is estimated that at baseline some 25%–40% of D2 receptors are occupied by endogenous dopamine (54, 55). Antipsychotics are thought to exert their effects by modulating dopaminergic transmission. In this section we will illustrate how drugs with a fast koff modulate dopamine transmission differently from drugs with a slow koff.
Consequences of Fast Dissociation
Although an antipsychotic binds to receptors without competition in test tube experiments (usually), in the brain, antipsychotics are always competing with endogenous dopamine. The rate at which a drug dissociates from the receptor, or its koff, is the most important determinant of how drugs and dopamine compete. The faster the koff, the more quickly the drug responds to dopamine surges. The slower the koff, the slower is the drug in responding to changes in endogenous dopamine. In patients, antipsychotics with low affinity are given in proportionally higher doses. For example, a usual clinical dose is 2–4 mg/day of haloperidol versus 200–400 mg/day of clozapine (which has a 100-times lower affinity than haloperidol). Although the dose size does not change the behavior of the individual antipsychotic molecule, it has interesting consequences for the system. By inserting kon, koff, and concentrations of haloperidol and clozapine (53) into a competition model, one finds that clozapine reaches equilibrium 100 times faster than haloperidol. Once at equilibrium, clozapine goes on to and off of the receptors 100 times in the time it takes haloperidol to do so once. Finally, when the concentration of endogenous dopamine rises in response to physiological stimuli, drugs like clozapine (which have a nearly 100 times faster koff) decrease their occupancy much faster and provide much more access to surges of dopamine (56). In summary, antipsychotics with a fast koff under clinical conditions give rise to a fast-on, fast-turnover, and fast-off blockade of D2 receptors.
Responsiveness to Endogenous Competition
“Clozapine causes a blockade of striatal dopamine receptors which is of the surmountable type in contrast to that produced by cataleptogenic neuroleptics.” In 1972 Bartholini et al. (57) made this statement even before D2 receptors were defined, but their thought regarding “surmountable” D2 blockade is a reasonable conceptual ancestor to our present hypothesis. Dopamine exerts its action by means of synaptic release (leading to a fast increase in dopamine levels) and reabsorption (leading to a decrease in dopamine levels). Fast-scan voltametry studies in animals (58, 59) have suggested that surges of several hundred percent from baseline can be recorded when animals are exposed to physiological conditions such as novelty; this increase equals the dopamine surges produced by phasic electrical stimulation. In humans, by means of the displacement of [11C]raclopride as an index of synaptic dopamine release, it has been shown that physiological acts such as playing a video game (60) can cause a dopamine release lasting several minutes and longer that is equal in magnitude to that observed with pharmacological challenges with substances such as amphetamine (61). Thus, current evidence suggests that for normal physiological functioning, baseline dopamine levels are punctuated with task-related several-fold increases in dopamine levels that last from seconds to minutes. In this time scale, the koff of an antipsychotic becomes critical.
Figure 2 shows the results of a simulation that examined the effects of a dopamine surge after achievement of equal levels of occupancy with two different antipsychotics. In the first condition, 66% of the receptors were occupied by an antipsychotic with a koff comparable to that of haloperidol; in the second condition, 66% of the receptors were occupied by an antipsychotic with a koff comparable to that of clozapine. Figure 2 clearly illustrates how despite equal levels of occupancy to start, drugs with a different koff permit different levels of phasic dopamine transmission. For the slow-koff drug (Figure 2, haloperidol), there was no significant impact of the dopamine surge, thus distorting physiological dopamine transmission. On the other hand, the fast-koff drug (clozapine) allowed attenuated physiologic transmission to continue. Thus, drugs with a fast koff competitively attenuated physiological transmission, whereas drugs with a slow koff distorted and extinguished it.
Implications of Fast Dissociation at the System Level
The relevance of a fast dissociation at the system level was hinted at by Burki, who suggested that
although occupation of striatal D2 receptors by [clozapine and fluperlapine] developed rapidly, the duration was considerably shorter than that of haloperidol…the low incidence of extrapyramidal side-effects is probably due to their weak and relatively brief action on brain DA systems. (62, emphasis added)
Clozapine, the quintessential atypical antipsychotic, shows clear evidence of a definite, yet transient, effect by means of the D2 system. Clozapine causes a clear dose-dependent elevation of dopamine turnover and release, indicating relevant dopamine blockade (62, 63). However, the effects of atypicals are much more transient than those of typical antipsychotics. In studies examining brain occupancy it has been shown that clozapine shows a rapid and transient D2 occupancy (62, 63), whereas haloperidol shows prolonged occupancy in the same models. As an example, Saller and Salama (63) found that 40 mg/kg of intraperitoneal clozapine produced 61% D2 occupancy within 30 minutes; this decreased to 0% in 4 hours. Haloperidol (1 mg/kg intraperitoneal) produced 57% occupancy at 30 minutes and was still at 62% at 4 hours. Similar and parallel evidence of transience is found with prolactin elevation. For example, both clozapine (20 mg/kg intraperitoneal) and haloperidol (0.25 mg/kg intraperitoneal) led to robust prolactin elevation in rats (from a baseline of <10 ng/ml to a peak of 70 ng/ml for both clozapine and haloperidol at 30 minutes [64]). However, the clozapine-induced prolactin elevation was completely back to normal at 2 hours, whereas the haloperidol level was still elevated (approximately 70 ng/ml) at 4 hours after receipt of the drug (64).
More recently, we replicated this pattern of findings in patients and produced significant transient prolactin elevations with the atypical antipsychotics clozapine, quetiapine, and olanzapine (23, 65). We found that even with prolactin-sparing atypicals such as olanzapine, clozapine, and quetiapine, almost all patients showed prolactin elevation (some of them in the abnormal range) 2–6 hours after receiving the last dose of drug. However, the prolactin levels declined rapidly and returned to normal by 12–24 hours after receipt of the last dose (23, 65). Thus, it is not that atypical antipsychotics do not cause prolactin elevation; they just cause a transient prolactin elevation, indicative of the transient functional effects of D2 blockade. Since the prolactin elevation subsides by the time of the next dose, it does not lead to drug accumulation and sustained high levels, as has been observed with prolactin-raising antipsychotics.
In short time frames, i.e., seconds to minutes, dissociation is largely determined by the koff of a drug. However, over longer time frames, i.e., hours and days, drug levels in the brain also change. Thus, in addition to fast dissociation at a molecular level, drugs also show different patterns of system-level occupancy, depending largely on the manner in which their brain levels change with drug administration. Even for a given drug (i.e., with molecular koff fixed), different temporal patterns of system-level occupancy have important differential consequences. Kashihara et al. (66) administered a given dose of haloperidol by means of a daily injection (which gives rise to transiently high system-level occupancy) or with a subcutaneous pump (which provides sustained system-level occupancy) and found that the sustained mode of administration led to significantly higher D2 receptor up-regulation and induced tolerance to subsequent dopamine blockade. See and Ellison (67) found that sustained administration led to the development of tardive dyskinesia-like motor symptoms (suggestive of up-regulation), whereas intermittent (weekly) administration did not produce these motor symptoms and, if anything, led to dystonia-like acute extrapyramidal symptoms suggestive of greater sensitivity to dopamine blockade. In several other studies (66–69) a similar pattern was observed: sustained blockade led to tolerance and up-regulation, whereas transient occupancy avoided tolerance and up-regulation and made the system more sensitive to the antidopaminergic effects of antipsychotics. This differential response to transient versus sustained occupancy probably reflects an underlying property of the dopamine system, since a similar pattern of changes has also been observed with dopaminergic agonists in the context of Parkinson’s disease or drug abuse (70–72).
Implications of the Fast Dissociation Hypothesis
Other Features of Atypicality
Typical antipsychotics give rise to a significant and reliable improvement in negative symptoms (10). This is a fact that has often been overlooked in debates over typicals versus atypicals. As an example, in a study of more than 1,000 patients in a comparison of haloperidol (10 mg/day) and risperidone (1–16 mg/day) (73), haloperidol improved negative subscale scores on the Positive and Negative Syndrome Scale by a score of 4.8, whereas risperidone improved them by a score of 4.5–5.5 depending on dose, with none of the differences found to be reliably different (73). The superiority of the newer atypicals over haloperidol is rather small: two of the four newer atypicals do not show this at present (10), and even some well-controlled studies of clozapine did not find this improvement (74). Third, even for the drugs for which this superiority was observed, it has only been shown in trials using high doses of haloperidol, doses that are known to induce secondary negative symptoms (10). Any drug that improves psychosis should, and does, improve overall negative symptoms (75). In addition to this effect we propose that antipsychotics with a low affinity and fast koff allow for more physiological dopamine transmission (Figure 2) and because of their lesser propensity to cause extrapyramidal side effects, give rise to an even greater improvement in negative symptoms. We cannot say whether fast dissociation is responsible for improvement in primary negative symptoms because it is still a matter of debate whether such a differentiation (primary versus negative) is feasible (76) and whether any antipsychotic (even clozapine) can improve primary negative symptoms (74, 77).
One of the unique features of clozapine is its effectiveness in patients whose illness is refractory to treatment with typical antipsychotics (78). It has yet to be established whether any of the other atypical antipsychotics replicates this feature (79). On the other hand, patients who do not respond to the newer antipsychotics (risperidone and olanzapine) do show a good response to clozapine (80). The precise reason for the preferential effect of clozapine in patients with refractory illness is not known, since studies of newer atypical as have shown that they have a high affinity at almost all the receptors at which clozapine has a high affinity (e.g., 5-HT2, D4, and muscarinic) without replicating clozapine’s efficacy in those patients. Our hypothesis does not formally deal with this aspect of treatment. However, one idea merits mention. It has been shown that with repeated transient blockade, the dopamine system becomes more sensitive to the effects of dopamine blockade, whereas with continuous dopamine blockade, the system becomes tolerant and up-regulates (66–69). By leading to fast dissociation at a molecular as well as at a system level, clozapine may sensitize the system, a property that has been suggested as a basis for its effect in treating patients with refractory illness (81). At the same time it is equally likely that the action of clozapine on some other receptors is the key to this added efficacy in the treatment of refractory symptoms. Until there is a nonclozapine antipsychotic that can replicate clozapine’s efficacy in treating refractory symptoms, both options should be considered.
Optimal Parameters for Atypicality
Like most biological systems, fast koff and transient occupancy are expected to work best under a defined set of parameters. At a molecular level, if a drug is too fast in its actions at the D2 receptor, it will be too transient to provide any alteration in dopamine transmission and will likely not be an antipsychotic. Clearly then, there must be some limits on how fast fast dissociation should be. On one issue the new hypothesis is clear: we do not propose periods without any D2 occupancy (such as a drug holiday). In fact, we think that a continuous D2 occupancy at a system level is essential. However, we question whether that D2 occupancy needs to be held at a steady, fixed, or high level or whether an antipsychotic effect may be obtained by only transiently high occupancy (23). What the optimal value of koff is and what the best relationship of peak and trough D2 occupancy are can only be defined by future research.
Implications for Drug Research
In minimizing the role of receptors other than D2, our hypothesis has particular implications for drug development. First, we propose that the most straightforward path for atypical antipsychotic activity is by means of a specific low-affinity D2 blocker with a fast koff and a fast pharmacokinetic profile. Second, it has often been conjectured that partial agonists/antagonists may lead to a transient or more balanced blockade with respect to endogenous dopamine (82). By proposing that appropriate action at the D2 receptor alone is sufficient to explain atypicality, our hypothesis keeps open the possibility that other efforts at balanced blockade at the D2 receptor (e.g., partial agonist/antagonist or preferential presynaptic versus postsynaptic) may also provide the atypical antipsychotic action. Third, our hypothesis does not in any way imply that other receptors cannot make relevant contributions. Schizophrenia is a complex disease with psychotic, mood, and cognitive features. Our hypothesis fully anticipates that some of the nonpsychotic aspects (or even the refractory psychosis) of schizophrenia may be amenable to other molecular strategies. However, our hypothesis does imply that the true relevance of any other system (e.g., 5-HT2, 5-HT6/7, D4, and adrenergic α1) can be ascertained only if one first fully controls for the effect of D2 occupancy. Finally, our hypothesis also suggests that if blockade of other receptors is to be combined with that of D2 receptors, then the D2 blockade should be of the fast koff type. Animal experiments show that even if clozapine (with all its additional molecular properties) is combined with a D2 blocker such as haloperidol, it cannot prevent the development of dopamine receptor up-regulation and supersensitivity (83).
Summation
The fast dissociation hypothesis suggests that the combination of a fast koff at the molecular level and transient D2 occupancy at the system level is sufficient to provide an atypical antipsychotic effect. One does not need to invoke activity in other receptors. Previous claims regarding the importance of 5-HT2 and D4 receptors were confounded by the simultaneous presence of fast dissociation at the D2 receptor. It is proposed that drugs with fast dissociation when used in doses that lead to appropriately high D2 blockade modulate the dopamine system in a manner that allows for more appropriate functioning of physiological systems and that this leads to what is currently called the atypical antipsychotic effect.
Received Feb. 15, 2000; revisions received June 7 and July 19, 2000; accepted Aug. 1, 2000. From the Schizophrenia Program and the PET Centre of the Centre for Addiction and Mental Health; and the Department of Psychiatry and the Department of Pharmacology, University of Toronto. Address reprint requests to Dr. Kapur, Clarke Division, CAMH, 250 College St., Toronto, Ont., Canada M5T 1R8; [email protected] (e-mail).Supported by the Medical Research Council of Canada, the EJLB Foundation (Canada), the Stanley Foundation, and the National Alliance for Research on Schizophrenia and Depression. The authors thank their colleagues in the Schizophrenia Program (particularly Drs. Robert Zipursky, Gary Remington, and Mary Seeman) for their discussions and comments.
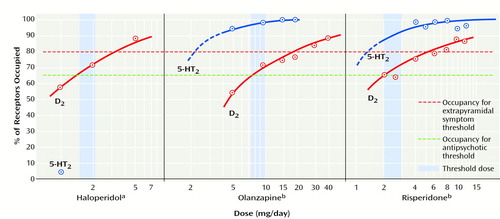
Figure 1. Relation of Threshold for Clinical Response to Occupancy of Dopamine D2 and Serotonin 5-HT2 Receptors for Haloperidol, Olanzapine, and Risperidone
aThreshold for response to haloperidol is 65% (1.5–2.1 mg/day).
bOlanzapine (7.5–10 mg/day) and risperidone (2 mg/day) also reach their thresholds of effectiveness only when their D2 occupancy reaches 65%, despite the fact that haloperidol has a negligible effect at the 5-HT2 receptor and olanzapine and risperidone show high 5-HT2 occupancy (29, 30, 35).
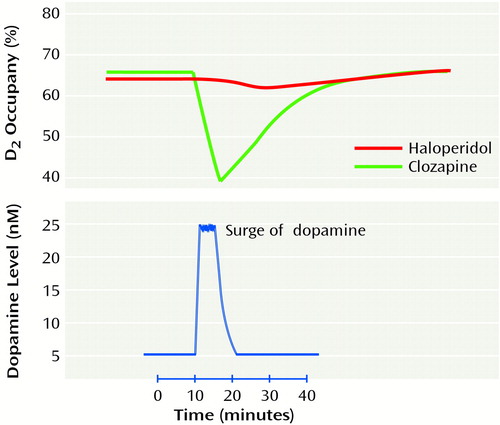
Figure 2. Effects on D2 Occupancy of a Surge of Dopamine After Achievement of Equal Levels of Occupancy by Haloperidol and by Clozapinea
aSimulation performed by means of STELLA model-simulation software (High Performance Systems, Hanover, N.H.). Haloperidol occupancy is unchanged by physiological dopamine transmission. Clozapine occupancy decreases to allow physiological dopamine transmission.
1. Hippius H: A historical perspective of clozapine. J Clin Psychiatry 1999; 60(suppl 12):22–23Google Scholar
2. Gerlach J, Peacock L: New antipsychotics: the present status. Int Clin Psychopharmacol 1995; 10:39–48Medline, Google Scholar
3. Waddington JL, O’Callaghan E: What makes an antipsychotic “atypical”? conserving the definition. CNS Drugs 1997; 7:341–346Crossref, Google Scholar
4. Copolov D: New name for atypical antipsychotics? (letter). Am J Psychiatry 1997; 154:439Medline, Google Scholar
5. Hippius H: The history of clozapine. Psychopharmacology (Berl) 1989; 99(suppl):S3–S5Google Scholar
6. Deutch AY, Moghaddam B, Innis RB, Krystal JH, Aghajanian GK, Bunney BS, Charney DS: Mechanisms of action of atypical antipsychotic drugs. Schizophr Res 1991; 4:121–156Crossref, Medline, Google Scholar
7. Kinon BJ, Lieberman JA: Mechanisms of action of atypical antipsychotic drugs: a critical analysis. Psychopharmacology (Berl) 1996; 124:2–34Crossref, Medline, Google Scholar
8. Lieberman JA: Understanding the mechanism of action of atypical antipsychotic drugs: a review of compounds in use and development. Br J Psychiatry Suppl 1993; 22:7–18; correction, Br J Psychiatry 1994; 164:709Google Scholar
9. Kerwin RW: The new atypical antipsychotics: a lack of extrapyramidal side-effects and new routes in schizophrenia research. Br J Psychiatry 1994; 164:141–148Crossref, Medline, Google Scholar
10. Leucht S, Pitschel-Walz G, Abraham D, Kissling W: Efficacy and extrapyramidal side-effects of the new antipsychotics olanzapine, quetiapine, risperidone, and sertindole compared to conventional antipsychotics and placebo: a meta-analysis of randomized controlled trials. Schizophr Res 1999; 35:51–68Crossref, Medline, Google Scholar
11. Peuskens J (ed): Prolactin in Schizophrenia. London, Franklin Scientific Projects Ltd, 1997, pp 1–44Google Scholar
12. Zimbroff DL, Kane JM, Tamminga CA, Daniel DG, Mack RJ, Wozniak PJ, Sebree TB, Wallin BA, Kashkin KB (Sertindole Study Group): Controlled, dose-response study of sertindole and haloperidol in the treatment of schizophrenia. Am J Psychiatry 1997; 154:782–791Link, Google Scholar
13. Limbird L: Cell Surface Receptors: A Short Course on Theory and Methods. Boston, Kluwer Academic, 1986Google Scholar
14. Kenakin T: Pharmacologic Analysis of Drug-Receptor Interaction. Philadelphia, Lippincott-Raven, 1997Google Scholar
15. Farde L, Nordstrom AL, Wiesel FA, Pauli S, Halldin C, Sedvall G: Positron emission tomographic analysis of central D1 and D2 dopamine receptor occupancy in patients treated with classical neuroleptics and clozapine: relation to extrapyramidal side effects. Arch Gen Psychiatry 1992; 49:538–544Crossref, Medline, Google Scholar
16. Nordstrom AL, Farde L, Wiesel FA, Forslund K, Pauli S, Halldin C, Uppfeldt G: Central D2-dopamine receptor occupancy in relation to antipsychotic drug effects: a double-blind PET study of schizophrenic patients. Biol Psychiatry 1993; 33:227–235Crossref, Medline, Google Scholar
17. Kapur S, Zipursky R, Jones C, Remington G, Houle S: Relationship between dopamine D2 occupancy, clinical response, and side effects: a double-blind PET study of first-episode schizophrenia. Am J Psychiatry 2000; 157:514–520Link, Google Scholar
18. Daskalakis Z, Christensen B, Zipursky R, Zhang-Wong J, Beiser M: Relationship between D2 occupancy and prolactin levels in first episode psychosis (abstract). Biol Psychiatry 1998; 43:113SCrossref, Google Scholar
19. Schlegel S, Schlosser R, Hiemke C, Nickel O, Bockisch A, Hahn K: Prolactin plasma levels and D-2-dopamine receptor occupancy measured with IBZM-SPECT. Psychopharmacology (Berl) 1996; 124:285–287Crossref, Medline, Google Scholar
20. Schroder J, Silvestri S, Bubeck B, Karr M, Demisch S, Scherrer S, Geider FJ, Sauer H: D-2 dopamine receptor up-regulation, treatment response, neurological soft signs, and extrapyramidal side effects in schizophrenia: follow-up study with I-123-iodobenzamide single photon emission computed tomography in the drug-naive state and after neuroleptic treatment. Biol Psychiatry 1998; 43:660–665Crossref, Medline, Google Scholar
21. Scherer J, Tatsch K, Schwarz J, Oertel WH, Konjarczyk M, Albus M: D2-dopamine receptor occupancy differs between patients with and without extrapyramidal side effects. Acta Psychiatr Scand 1994; 90:266–268Crossref, Medline, Google Scholar
22. Kapur S: A new framework for investigating antipsychotic action in humans: lessons from PET imaging. Mol Psychiatry 1998; 3:135–140Crossref, Medline, Google Scholar
23. Kapur S, Zipursky R, Jones C, Shammi CS, Remington G, Seeman P: A positron emission tomography study of quetiapine in schizophrenia: a preliminary finding of an antipsychotic effect with only transiently high dopamine D2 receptor occupancy. Arch Gen Psychiatry 2000; 57:553–559Crossref, Medline, Google Scholar
24. Knable MB, Heinz A, Raedler T, Weinberger DR: Extrapyramidal side effects with risperidone and haloperidol at comparable D2 receptor occupancy levels. Psychiatr Res Neuroimaging 1997; 75:91–101Crossref, Medline, Google Scholar
25. Scherer J, Tatsch K, Schwarz J, Oertel W, Kirsch MC, Albus M: Striatal D2-dopamine receptor occupancy during treatment with typical and atypical neuroleptics. Biol Psychiatry 1994; 36:627–629Crossref, Medline, Google Scholar
26. Tauscher J, Kufferle B, Asenbaum S, Fischer P, Pezawas L, Barnas C, Tauscher-Wisniewski S, Brucke T, Kasper S: In vivo 123I IBZM SPECT imaging of striatal dopamine-2 receptor occupancy in schizophrenic patients treated with olanzapine in comparison to clozapine and haloperidol. Psychopharmacology (Berl) 1999; 141:175–181Crossref, Medline, Google Scholar
27. Gefvert O, Bergstrom M, Langstrom B, Lundberg T, Lindstrom L, Yates R: Time course of central nervous dopamine-D-2 and 5-HT2 receptor blockade and plasma drug concentrations after discontinuation of quetiapine (Seroquel) in patients with schizophrenia. Psychopharmacology (Berl) 1998; 135:119–126Crossref, Medline, Google Scholar
28. Jones C, Kapur S, Remington G, Zipursky RB: Transient dopamine D2 occupancy in low EPS-incidence drugs: PET evidence (abstract). Biol Psychiatry 2000; 47(8 suppl):112Google Scholar
29. Kapur S, Zipursky RB, Remington G, Jones C, DaSilva J, Wilson AA, Houle S:5-HT2 and D2 receptor occupancy of olanzapine in schizophrenia: a PET investigation. Am J Psychiatry 1998; 155:921–928Google Scholar
30. Kapur S, Zipursky RB, Remington G: Clinical and theoretical implications of 5-HT2 and D2 receptor occupancy of clozapine, risperidone, and olanzapine in schizophrenia. Am J Psychiatry 1999; 156:286–293Abstract, Google Scholar
31. Nyberg S, Farde L, Eriksson L, Halldin C, Eriksson B:5-HT2 and D2 dopamine receptor occupancy in the living human brain: a PET study with risperidone. Psychopharmacology (Berl) 1993; 110:265–272Google Scholar
32. Nyberg S, Farde L, Halldin C: A PET study of 5-HT2 and D2 dopamine receptor occupancy induced by olanzapine in healthy subjects. Neuropsychopharmacology 1997; 16:1–7Crossref, Medline, Google Scholar
33. Kapur S, Zipursky R, Remington G, Jones C, McKay G, Houle S: PET evidence that loxapine is an equipotent blocker of 5-HT2 and D2 receptors: implications for the treatment of schizophrenia. Am J Psychiatry 1997; 154:1525–1529Google Scholar
34. Trichard C, Paillère-Martinot M-L, Attar-Levy D, Recassens C, Monnet F, Martinot J-L: Binding of antipsychotic drugs to cortical 5-HT2A receptors: a PET study of chlorpromazine, clozapine, and amisulpride in schizophrenic patients. Am J Psychiatry 1998; 155:505–508Link, Google Scholar
35. Kapur S, Zipursky R, Jones C, Remington G, Houle S: Relationship between dopamine D2 occupancy, clinical response, and side effects: a double-blind PET study of first-episode schizophrenia. Am J Psychiatry 2000; 157:514–520Link, Google Scholar
36. Truffinet P, Tamminga CA, Fabre LF, Meltzer HY, Rivière ME, Papillon-Downey C: Placebo-controlled study of the D4/5-HT2A antagonist fananserin in the treatment of schizophrenia. Am J Psychiatry 1999; 156:419–425Abstract, Google Scholar
37. Report S: Management decisions on priority pipeline products: MDL 100907 (Company Report). Frankfurt, Germany, Hoechst Marion Roussel, 1999, pp 2–3Google Scholar
38. Kapur S:5-HT2 antagonism and EPS benefits: is there a causal connection? Psychopharmacology (Berl) 1996; 124:35–39Google Scholar
39. Kapur S, Remington G: Serotonin-dopamine interaction and its relevance to schizophrenia. Am J Psychiatry 1996; 153:466–476Link, Google Scholar
40. Meltzer HY, Matsubara S, Lee JC: The ratios of serotonin-2 and dopamine-2 affinities differentiate atypical and typical antipsychotic drugs. Psychopharmacol Bull 1989; 25:390–392Medline, Google Scholar
41. Stockmeier CA, DiCarlo JJ, Zhang Y, Thompson P, Meltzer HY: Characterization of typical and atypical antipsychotic drugs based on in vivo occupancy of serotonin 2 and dopamine 2 receptors. J Pharmacol Exp Ther 1993; 266:1374–1384Google Scholar
42. Bristow LJ, Kramer MS, Kulagowski J, Patel S, Ragan CI, Seabrook GR: Schizophrenia and L-745, 870, a novel dopamine D4 receptor antagonist. Trends Pharmacol Sci 1997; 18:186–188Crossref, Medline, Google Scholar
43. Gessa GL, Canu A, Del Zompo M, Burrai C, Serra G: Lack of acute antipsychotic effect of Sch 23390, a selective dopamine D1 receptor antagonist (letter). Lancet 1991; 337:854–855Crossref, Medline, Google Scholar
44. Meltzer HY, Matsubara S, Lee JC: Classification of typical and atypical antipsychotic drugs on the basis of dopamine D1, D2 and serotonin2 pKi values. J Pharmacol Exp Ther 1989; 251:238–246Medline, Google Scholar
45. Puech A, Fleurot O, Rein W (Amisulpride Study Group): Amisulpride, an atypical antipsychotic, in the treatment of acute episodes of schizophrenia: a dose-ranging study vs haloperidol. Acta Psychiatr Scand 1998; 98:65–72Crossref, Medline, Google Scholar
46. Freeman HL: Amisulpride compared with standard neuroleptics in acute exacerbations of schizophrenia: three efficacy studies. Int Clin Psychopharmacol 1997; 12:S11–S17Google Scholar
47. Lewander T: Overcoming the neuroleptic-induced deficit syndrome: clinical observations with remoxipride. Acta Psychiatr Scand Suppl 1994: 380:64–67Google Scholar
48. Paillère-Martinot M-L, Lecrubier Y, Martinot J-L, Aubin F: Improvement of some schizophrenic deficit symptoms with low doses of amisulpride. Am J Psychiatry 1995; 152:130–133Link, Google Scholar
49. Loo H, Poirier-Littre MF, Theron M, Rein W, Fleurot O: Amisulpride versus placebo in the medium-term treatment of the negative symptoms of schizophrenia. Br J Psychiatry 1997; 170:18–22Crossref, Medline, Google Scholar
50. Seeman P, Van Tol HHM: Dopamine receptor pharmacology. Trends Pharmacol Sci 1994; 15:264–270Crossref, Medline, Google Scholar
51. Seeman P, Corbett R, Van Tol HHM: Atypical neuroleptics have low affinity for dopamine D2 receptors or are selective for D4 receptors (reply). Neuropsychopharmacology 1997; 16:127–135Crossref, Google Scholar
52. Scatton B, Claustre Y, Cudennec A, Oblin A, Perrault G, Sanger DJ, Schoemaker H: Amisulpride: from animal pharmacology to therapeutic action. Int Clin Psychopharmacol 1997; 12(suppl 2):S29–S36Google Scholar
53. Kapur S, Seeman P: Antipsychotic agents differ in how fast they come off the dopamine D2 receptors: implications for atypical antipsychotic action. J Psychiatry Neurosci 2000; 25:161–166Medline, Google Scholar
54. Laruelle M, D’Souza CD, Baldwin RM, Abi-Dargham A, Kanes SJ, Fingado CL, Seibyl JP, Zoghbi SS, Bowers MB, Jatlow P, Charney DS, Innis RB: Imaging D2 receptor occupancy by endogenous dopamine in humans. Neuropsychopharmacology 1997; 17:162–174Crossref, Medline, Google Scholar
55. Ginovart N, Farde L, Halldin C, Swahn CG: Effect of reserpine-induced depletion of synaptic dopamine on [11C]raclopride binding to D2-dopamine receptors in the monkey brain. Synapse 1997; 25:321–325Crossref, Medline, Google Scholar
56. Seeman P, Tallerico T: Rapid release of antipsychotic drugs from dopamine D2 receptors: an explanation for low receptor occupancy and early clinical relapse upon withdrawal of clozapine or quetiapine. Am J Psychiatry 1999; 156:876–884Link, Google Scholar
57. Bartholini G, Haefely W, Jalfre M, Keller HH, Pletscher A: Effects of clozapine on cerebral catecholaminergic neurone systems. Br J Pharmacol 1972; 46:736–740Crossref, Medline, Google Scholar
58. Rebec GV, Christensen JR, Guerra C, Bardo MT: Regional and temporal differences in real-time dopamine efflux in the nucleus accumbens during free-choice novelty. Brain Res 1997; 776:61–67Crossref, Medline, Google Scholar
59. Garris PA, Christensen JR, Rebec GV, Wightman RM: Real-time measurement of electrically evoked extracellular dopamine in the striatum of freely moving rats. J Neurochem 1997; 68:152–161Crossref, Medline, Google Scholar
60. Koepp MJ, Gunn RN, Lawrence AD, Cunningham VJ, Dagher A, Jones T, Brooks DJ, Bench CJ, Grasby PM: Evidence for striatal dopamine release during a video game. Nature 1998; 393:266–268Crossref, Medline, Google Scholar
61. Breier A, Su TP, Saunders R, Carson RE, Kolachana BS, de Bartolomeis A, Weinberger DR, Weisenfeld N, Malhotra AK, Eckelman WC, Pickar D: Schizophrenia is associated with elevated amphetamine-induced synaptic dopamine concentrations: evidence from a novel positron emission tomography method. Proc Natl Acad Sci USA 1997; 94:2569–2574Google Scholar
62. Burki HR: Effects of fluperlapine on dopaminergic systems in rat brain. Psychopharmacology (Berl) 1986; 89:77–84Crossref, Medline, Google Scholar
63. Saller CF, Salama AI: Seroquel: biochemical profile of a potential atypical antipsychotic. Psychopharmacology (Berl) 1993; 112:285–292Crossref, Medline, Google Scholar
64. Gudelsky GA, Koenig JI, Simonovic M, Koyama T, Ohmori T, Meltzer HY: Differential effects of haloperidol, clozapine, and fluperlapine on tuberoinfundibular dopamine neurons and prolactin secretion in the rat. J Neural Transm 1987; 68:227–240Crossref, Medline, Google Scholar
65. Turrone P, Kapur S, Seeman MV, Brown GM, Rahman MZ, Flint A: Olanzapine and clozapine elevate prolactin after every daily dose (abstract). Biol Psychiatry 2000; 47(8 suppl):155Google Scholar
66. Kashihara K, Sato M, Fujiwara Y, Harada T, Ogawa T, Otsuki S: Effects of intermittent and continuous haloperidol administration on the dopaminergic system in the rat brain. Biol Psychiatry 1986; 21:650–656Crossref, Medline, Google Scholar
67. See RE, Ellison G: Intermittent and continuous haloperidol regimens produce different types of oral dyskinesias in rats. Psychopharmacology (Berl) 1990; 100:404–412Crossref, Medline, Google Scholar
68. Csernansky JG, Bellows EP, Barnes DE, Lombrozo L: Sensitization versus tolerance to the dopamine turnover-elevating effects of haloperidol: the effect of regular/intermittent dosing. Psychopharmacology (Berl) 1990; 101:519–524Crossref, Medline, Google Scholar
69. Masuda Y, Murai S, Itoh T: Tolerance and reverse tolerance to haloperidol catalepsy induced by the difference of administration interval in mice. Jpn J Pharmacol 1982; 32:1186–1188Google Scholar
70. Blanchet PJ, Calon F, Martel JC, Bedard PJ, Di Paolo T, Walters RR, Piercey MF: Continuous administration decreases and pulsatile administration increases behavioral sensitivity to a novel dopamine D2 agonist (U-91356A) in MPTP-exposed monkeys. J Pharmacol Exp Ther 1995; 272:854–859Medline, Google Scholar
71. Reith ME, Benuck M, Lajtha A: Cocaine disposition in the brain after continuous or intermittent treatment and locomotor stimulation in mice. J Pharmacol Exp Ther 1987; 243:281–287Medline, Google Scholar
72. Torres G, Rivier C: Differential effects of intermittent or continuous exposure to cocaine on the hypothalamic-pituitary-adrenal axis and c-fos expression. Brain Res 1992; 571:204–211Crossref, Medline, Google Scholar
73. Peuskens J: Risperidone in the treatment of patients with chronic schizophrenia: a multi-national, multi-centre, double-blind, parallel-group study versus haloperidol. Br J Psychiatry 1995; 166:712–726Crossref, Medline, Google Scholar
74. Buchanan RW, Breier A, Kirkpatrick B, Ball P, Carpenter WT Jr: Positive and negative symptom response to clozapine in schizophrenic patients with and without the deficit syndrome. Am J Psychiatry 1998; 155:751–760Link, Google Scholar
75. Carpenter WT Jr, Heinrichs DW, Wagman AMI: Deficit and nondeficit forms of schizophrenia: the concept. Am J Psychiatry 1988; 145:578–583Link, Google Scholar
76. Flaum M, Andreasen NC: The reliability of distinguishing primary versus secondary negative symptoms. Compr Psychiatry 1995; 36:421–427Crossref, Medline, Google Scholar
77. Carpenter WT Jr, Conley R, Kirkpatrick B: On schizophrenia and new generation drugs. Neuropsychopharmacology 2000; 22:660–664Crossref, Medline, Google Scholar
78. Kane J, Honigfeld G, Singer J, Meltzer H: Clozapine for the treatment-resistant schizophrenic: a double-blind comparison with chlorpromazine. Arch Gen Psychiatry 1988; 45:789–796Crossref, Medline, Google Scholar
79. Fleischhacker WW: Clozapine: a comparison with other novel antipsychotics. J Clin Psychiatry 1999; 60(suppl 12):30–34Google Scholar
80. Conley RR, Tamminga CA, Kelly DL, Richardson CM: Treatment-resistant schizophrenic patients respond to clozapine after olanzapine non-response. Biol Psychiatry 1999; 46:73–77Crossref, Medline, Google Scholar
81. Stevens JR, Denney D, Szot P: Sensitization with clozapine: beyond the dopamine hypothesis. Biol Psychiatry 1997; 42:771–780Crossref, Medline, Google Scholar
82. Carlsson A, Waters N, Carlsson ML: Neurotransmitter interactions in schizophrenia-therapeutic implications. Biol Psychiatry 1999; 46:1388–1395Google Scholar
83. Carvey PM, Nath ST, Kao LC, Zhang TJ, Lin DH, Singh R, Amdur RL, Klawans HL: Clozapine fails to prevent the development of haloperidol-induced behavioral hypersensitivity in a cotreatment paradigm. Eur J Pharmacol 1990; 184:43–53Crossref, Medline, Google Scholar