Rapid Release of Antipsychotic Drugs From Dopamine D2 Receptors: An Explanation for Low Receptor Occupancy and Early Clinical Relapse Upon Withdrawal of Clozapine or Quetiapine
Abstract
OBJECTIVE: In an attempt to understand the basis of early relapse after antipsychotic withdrawal, the objective of this study was to determine whether the low occupancy of dopamine D2 receptors by clozapine and by quetiapine, as seen by brain imaging, could arise from a rapid release of some of the D2-bound clozapine or quetiapine by the brain imaging compounds and by the action of a physiological concentration of dopamine. METHOD: Human cloned D2 receptors were first pre-equilibrated with the [3H]antipsychotic drug, after which raclopride, iodobenzamide, or dopamine (at the physiological concentration in the synapse) was added, and the time course of release of the [3H]antipsychotic from the D2 receptor was measured. RESULTS: Within 5 minutes, low concentrations of raclopride and iodobenzamide displaced appreciable amounts of [3H]clozapine and [3H]quetiapine from the D2 receptors but, during the course of 1 hour, did not displace any of the other antipsychotic [[3H]ligands. [3H]Clozapine and [3H]quetiapine, moreover, were displaced by dopamine (100 nM) at least 100 times faster than the other antipsychotic [3H]ligands. CONCLUSIONS: Clozapine and quetiapine are loosely bound to the D2 receptor, and the injected radioactive ligand at its peak concentration may displace some of the D2-bound antipsychotic drug, resulting in apparently low D2 occupancies. Therefore, under clinical brain imaging conditions with [11C]raclopride, D2 occupancies by clozapine and by quetiapine may be higher than currently estimated. These considerations may result in high levels of the D2 receptors being occupied by therapeutic doses of clozapine or quetiapine. The rapid release of clozapine and quetiapine from D2 receptors by endogenous dopamine may contribute to low D2 receptor occupancy and to early clinical relapse upon withdrawal of these medications.
In the treatment of patients with schizophrenia, withdrawal of clozapine often results in early clinical relapse with psychotic symptoms within 1–14 days (1–11). A similar clinical situation appears to occur with quetiapine (12). Ordinarily, the rate of relapse in patients who have had antipsychotic drugs withdrawn is approximately 6%–10% per month for those who have been taking traditional antipsychotics such as phenothiazines, haloperidol, loxapine, and flupenthixol (13, 14). The relapse rate for those taking clozapine or quetiapine, however, is approximately five times higher (7, 9, 12). The rapid relapse after clozapine withdrawal has been variously attributed to clozapine-induced supersensitivity of the receptors for dopamine (1, 2), acetylcholine (4, 8), or serotonin (9). The research in the present study, however, illustrates that, more than any of the other antipsychotic drugs, clozapine and quetiapine are rapidly displaced from dopamine D2 receptors by competing compounds, including endogenous dopamine—a factor that may contribute to the early relapse of patients who have had these drugs withdrawn.
A reported feature of clozapine is that therapeutic doses apparently occupy low levels of dopamine D2 receptors, generally between 20% and 50%, as monitored by positron emission tomography (PET) (15–18) or by single photon emission computed tomography (SPECT) (19–28). This is in contrast to all other antipsychotic drugs, which, when given at equally effective therapeutic doses, occupy between 60% and 80% of dopamine D2 receptors, as monitored by PET (29–44) or by SPECT (19–28, 45–47). This feature of low D2 occupancy by clozapine is also seen with quetiapine, therapeutic doses of which occupy 25%–45% of D2 receptors (48–52).
The reports of consistently low occupancy of D2 receptors by clozapine has, therefore, appeared to “support the hypothesis that the mechanism of action of clozapine involves other receptor systems than the D2 subtype” (16; see also reference 44). If this were true, it would have major consequences for the search for the causes of psychosis and for the design of future antipsychotic medications. The research in the present study, however, shows that the low occupancy of D2 receptors by clozapine or by quetiapine may be partly due to rapid displacement of some of the clozapine or quetiapine from the receptors by endogenous dopamine and by the injected [11C]raclopride or [123I]iodobenzamide ([123I]IBZM) used in imaging the brain dopamine D2 receptors.
METHOD
The experiments in this study used human cloned dopamine D2long receptors in Spodoptera frugiperda insect cells (Biosignal, Inc., Montreal) or human cloned dopamine D2short receptors in mouse L cells (Biosignal, Inc.). The two types of tissue yielded identical results.
Displacement Method
The displacement of antipsychotic [3H]ligands from dopamine D2 receptors by raclopride, by iodobenzamide, or by 100 nM dopamine was done as follows. The frozen pellet of cloned D2 receptors was resuspended in 10 ml of buffer (50 mM Tris-HCl, pH 7.4; 1 mM EDTA; 5 mM KCl; 1.5 mM CaCl2; 4 mM MgCl2; and 120 mM NaCl) at a final concentration of 10 µg protein/ml. The final suspension contained one of the following antipsychotic [3H]ligands with or without additional nonradioactive drug added, to match the molarities of antipsychotic drug found in the spinal fluid or in the plasma water of patients: 10 nM [3H]clozapine (84 Ci/mmol; New England Nuclear Corp., Boston) and 300 nM clozapine; 10 nM [3H]quetiapine (14 Ci/mmol; custom-prepared by New England Nuclear Corp.) and 200 nM quetiapine; 2 nM [3H]haloperidol (12 Ci/mmol; New England Nuclear Corp.); 2.5 nM [3H]chlorpromazine (25 Ci/mmol; New England Nuclear Corp.); 10 nM [3H]remoxipride (46 Ci/mmol; prepared in 1991 by Astra Arcus AB, Södertälje, Sweden); 4.6 nM [3H]olanzapine (81 Ci/mmol; Lilly Research Laboratories, Indianapolis); 2.4 nM [3H]raclopride (79 Ci/mmol; New England Nuclear Corp.); or 4.4 nM [3H]sertindole (47 Ci/mmol; H. Lundbeck A/S, Copenhagen-Valby). The final suspension remained at room temperature for 1 hour to permit the [3H]antipsychotic to bind to the dopamine D2 receptors. An aliquot of 0.1 ml, containing raclopride, iodobenzamide, or dopamine, was then added to the 10-ml suspension. After rapid mixing, aliquots of 1 ml were quickly removed from the suspension at various times and immediately filtered under vacuum through presoaked glass fiber filters (Whatman GF/B; Whatman Products, Clifton, N.J.) with the use of a Millipore filter manifold. After the filters were washed rapidly with 5 ml of buffer, they were placed in scintillation minivials (Packard, Chicago) and monitored for tritium 6 hours later in a Packard 4660 scintillation spectrometer at 55% efficiency. Nonspecific binding of each antipsychotic [3H]ligand was done in the presence of 10 µM S-sulpiride.
Competition Method
Raclopride and iodobenzamide were also tested for their ability to compete against [3H]clozapine or [3H]quetiapine by using the traditional competition equilibrium method (53, 54) as follows. Each incubation tube received 0.50 ml of buffer with varying concentrations of raclopride or iodobenzamide, 0.25 ml of [3H]clozapine (final concentration of 10 nM in the presence of a final concentration of 300 nM clozapine) or [3H]quetiapine (final concentration of 10 nM in the presence of a final concentration of 200 nM quetiapine), and 0.25 ml of tissue (10 µg protein per tube). After the tubes were incubated at room temperature for 2 hours, the incubates were filtered by means of a 12-well cell harvester (Titertek, Skatron, Lier, Norway) and buffer-presoaked glass fiber filter mats (number 7034, Skatron, Sterling, Va.). After filtering of the incubates, the filter mat was rinsed with 7.5 ml buffer for 15 seconds. The filters were pressed out, placed in scintillation minivials (Packard), and monitored for tritium as described above. Nonspecific binding was defined as the binding that occurred in the presence of 10 µM S-sulpiride.
RESULTS
Previous work (53, 54) had shown that clozapine and quetiapine have low affinity for D2 receptors—lower than any other antipsychotic drug—suggesting that the low affinity and loose binding of these drugs at D2 may cause them to be readily displaced from D2 by other drugs, including endogenous dopamine. This was directly examined, therefore, by using [3H]clozapine, [3H]quetiapine, raclopride, and dopamine.
Raclopride Displacement of [3H]Clozapine and [3H]Quetiapine
Raclopride readily displaced 310 nM [3H]clozapine within 5 minutes, but it did not displace D2-bound [3H]haloperidol (2 nM), [3H]chlorpromazine (2.5 nM), or [3H]olanzapine (4.6 nM), as shown in Figure 1. The molarities chosen for each of these [3H]ligands are those which are found in the spinal fluid or in the plasma water of patients receiving therapeutic maintenance doses of antipsychotic drugs (53, 54). The percent occupancy of D2 by each [3H]ligand was calculated as C/(C + K), where C was the [3H]ligand concentration, and K was the real binding constant (independent of the radioligand used [53, 54]) for the antipsychotic drug (44 nM for clozapine, 78 nM for quetiapine, 0.375 nM for haloperidol, 0.7 nM for chlorpromazine, and 2.9 nM for olanzapine [53, 54]). Raclopride did not displace [3H]sertindole, [3H]raclopride, or [3H]remoxipride (data not shown).
Within minutes, raclopride also readily displaced 210 nM [3H]quetiapine (Figure 2, top), including up to 410 nM (data not shown).
A full range of raclopride concentrations was tested on D2-bound [3H]clozapine and [3H]quetiapine. Raclopride concentrations between 0.07 and 0.15 nM displaced D2-bound [3H]clozapine (310 nM) within 10 minutes (Figure 3). Lower concentrations of raclopride (0.03– 0.09 nM) displaced D2-bound [3H]quetiapine (210 nM) within 10 minutes. (In order to simulate the PET procedure, [3H]raclopride was added to D2 receptors that had been pre-incubated with either clozapine or haloperidol at concentrations that bound to the same proportion of D2; the amount of [3H]raclopride binding, however, was consistently higher in the presence of clozapine [data not shown], suggesting that clozapine, not haloperidol, was partly replaced by [3H]raclopride.) Much higher concentrations of raclopride were needed to compete with these ligands when the conventional competition equilibrium method was used (where the tissue was added to tubes containing the ligand and the competing drug) (Figure 3).
Iodobenzamide Displacement of [3H]Clozapine and [3H]Quetiapine
A similar situation occurred with iodobenzamide, which displaced D2-bound [3H]clozapine and D2-bound [3H]quetiapine within minutes (Figure 2, middle), but which did not displace any of the other [3H]ligands (e.g., [3H]olanzapine, Figure 2, bottom). A full range of iodobenzamide concentrations was tested on D2-bound [3H]clozapine and on D2-bound [3H]quetiapine. Iodobenzamide concentrations between 0.02 and 0.1 nM displaced D2-bound [3H]clozapine (310 nM) as well as D2-bound [3H]quetiapine (210 nM) within 10 minutes (Figure 4). Somewhat higher concentrations of iodobenzamide were needed to compete with these ligands when the conventional competition method was used.
Dopamine Displacement of [3H]Clozapine and [3H]Quetiapine
A physiological concentration of dopamine, 100 nM, mimicking the average concentration of dopamine in the synapse (see Discussion), displaced D2-bound [3H]clozapine or [3H]quetiapine about 100 times more quickly than [3H]chlorpromazine, [3H]haloperidol, or [3H]olanzapine (Figure 5).
DISCUSSION
The main finding was that low concentrations of raclopride, iodobenzamide, or dopamine could (within minutes) displace some of the [3H]clozapine or [3H]quetiapine prebound to D2 receptors (Figure 3, Figure 4, Figure 5), in contrast to [3H]haloperidol and other [3H]antipsychotic drugs. This finding is relevant and applicable to clinical brain imaging as follows. The amount of [11C]raclopride injected into human volunteers for determining D2 occupancy by PET is between 15 and 100 nmol, with an average of 36 nmol (A. Wilson and S. Kapur, personal communication, and references 55–59). This average dose, when rapidly distributed in 5 liters of blood, yields a peak molarity in plasma of the order of 7 nM, with a final free concentration in the plasma water of the order of 0.03–0.3 nM raclopride (see references 31, 53, and 54 for data on free molarities of antipsychotic drugs). This concentration range of raclopride may displace some of the clozapine bound to the D2 receptors in a human subject’s striatum within 10 minutes, at which time the brain imaging measurements are started. As shown in Figure 1 and Figure 2, the effect of raclopride was long-lasting and persisted beyond 1 hour. (Under clinical conditions, of course, the patient subsequently resumes his or her medication [clozapine] after brain imaging, and any clozapine-displacing action of the injected raclopride dissipates as the raclopride is removed from the body.)
In particular, for example, the peak [11C]raclopride in striatum corresponds to about 0.005% of the injected radioactivity per gram of striatum (60). Taking the concentration of [11C]raclopride in the cerebellum (F*, according to the terminology of reference 29) as 0.0015% of the injected radioactivity per gram of striatum (at the time of peak striatal activity) as a rough measure of the “nonspecifically bound” tracer in striatum, one arrives at 0.0035% of the injected radioactivity per gram of striatum for the specifically bound [11C]raclopride (B*, according to the terminology of reference 29). Hence, as obtained by Volkow et al. (60), the ratio B*/F* is 2.3, in general agreement with the average value of 3.55 (SD=0.63) obtained by Farde et al. (29) for 15 drug-naive patients. Furthermore, as defined previously (29), receptor occupancy in a drug-treated patient is expressed as “the percent reduction of B*/F* from the value of 3.55.”
Hence, if 36 nmol of [11C]raclopride are injected, the concentration in the striatum will be about 1 pmol/gram, in agreement with values reported earlier (61). If it is assumed that the concentration of the D2 receptors in the human striatum is between 20 and 28 pmol/gram (62–64), the injected [11C]raclopride will occupy between 3% and 5% of the total population of D2 receptors. Thus, for example, an injection of 36 nmol of [11C]raclopride might result in 1 pmol/gram, or 3%, of the total D2 population being occupied in a drug-free subject. This control value of 1 pmol/gram, or 3% of total D2, would be designated as “0% occupancy” (29). For an individual taking a tightly bound antipsychotic drug such as haloperidol, however, the injection of the 36 nmol of [11C]raclopride would result in less specific binding of the [11C]raclopride, with an observed B*/F* value of, say, 1.1 instead of 3.55 (56). This fall in B*/F* would correspond to a D2 occupation by [11C]raclopride of 0.3 pmol/gram, or 1% of total D2. Hence, the drop from 3% to 1% of total D2 would indicate that haloperidol occupied about 67% of the D2 population.
However, in the case of a patient taking clozapine (or quetiapine), the injection of 36 nmol of [11C]raclopride (with a specific activity of 500–1,000 Ci/mmol) would displace some of the D2-bound clozapine, resulting in a value for B*/F* that would be slightly higher than expected for the true occupancy by clozapine. For example, if clozapine tightly occupied 67% of the D2 receptors, then one would observe the same data that were just mentioned for haloperidol, namely, an occupation of 1% of total D2 by [11C]raclopride. However, if some of the D2-bound clozapine is displaced by [11C]raclopride, the occupation by [11C]raclopride will not be as low as 1% of the total density of D2 receptors. For example, if such a displacement results in 2% of the total D2 being occupied by [11C]raclopride (compared to the control value of 3% of the total D2 density), the apparent occupancy by clozapine would be seen to be only 33% instead of the expected 67%.
A similar situation obtains with [123I]IBZM, used in SPECT, where between 1 and 2 nmol are injected. For example, European studies use [123I]IBZM provided by Cygne B.V. (Eindhoven, The Netherlands) (20–22, 65), which prepares [123I]IBZM by the method of Bobeldijk et al. (66). This method uses carrier-free Na123I and S-benzamide, such that the final specific activity of S-[123I]IBZM is the same as that of the carrier-free Na123I, namely, >3000 Ci/mmol. However, as later noted by Verhoeff et al. (67), to enable the accurate measurement of the specific activity of [123I]IBZM for both in vitro and in vivo studies, a small amount of carrier iodide is added to the labeling mixture, resulting in a specific activity of the prepared [123I]IBZM in the range of 50–250 MBq/nmol. Thus, the Cygne B.V. specifications indicate that the [123I]IBZM has 1 nmol/ml (0.4 µg/ml) of nonradioactive iodobenzamide. Usually, 185 MBq (or 5 mCi) are injected into the patient, and this dose corresponds to 1–2 nmol, depending on the time of injection. After being diluted in the bloodstream, and allowing for the fact that 4.4%–12.3% of the [123I]IBZM is free in the plasma water (68, 69), the final molarity of free iodobenzamide in the plasma water of a human volunteer is of the order of 0.02 nM–0.05 nM. This concentration range would be expected to displace some of the D2-bound clozapine or quetiapine from the human volunteer’s striatum in a matter of minutes, as indicated in Figure 4.
Thus, the data in Figure 4 may account for the low occupancies of 12%–32% of D2 by clozapine, as monitored by the [123I]IBZM used in the European studies (19–22, 51). In other words, according to the data in Figure 4, the D2 occupancies by clozapine in these studies may be underestimated. In the United States, however, the [123I]IBZM used by Pickar et al. (70) was carrier-free, yielding D2 occupancies by clozapine approaching 80%. Hence, the clozapine occupancy of D2 depends on the amount of [123I]IBZM injected, as illustrated in the summary in Figure 6.
Considering that iodobenzamide, as well as raclopride, may displace some of the D2-bound clozapine or quetiapine in the human brain striatum, then clozapine or quetiapine may occupy more dopamine D2 receptors in humans than are currently estimated. This is illustrated in Figure 7, which summarizes the observations that all antipsychotic drugs occupy 60%–80% of D2 receptors, except for clozapine and quetiapine. However, if allowance is made for some displacement of the D2-bound clozapine or quetiapine by the injected radioligand, then the D2 occupancies by clozapine and quetiapine may rise. This conclusion is the same as that derived previously by using the antipsychotic molarities in plasma water and using the ligand-independent dissociation constants of the antipsychotic drugs (54).
A particularly surprising result of this study was the observation that preexposure of the cloned D2 receptors to clozapine caused the receptors to be more readily displaced by raclopride. As shown in Figure 3, when the radioactive clozapine and the nonradioactive raclopride were added simultaneously (as is done in the traditional competition-type method), the K value (dissociation constant) of raclopride was about 1 nM, in agreement with previous data in vitro (53, 54) as well as in vivo in human positron tomography. (Although the raclopride K value in the striatum of human volunteers is between 7.7 and 9.1 nmol/gram of striatum [64, 71], these values convert to 1.5–1.8 nM, given that the tissue/buffer partition coefficient for raclopride is 5 [nmol/gram]/[nmol/ml] [53, 72–74), and L. Farde, personal communication].) However, when the cloned D2 receptors were preexposed to clozapine for 1 hour, raclopride displaced 50% of bound radioactive clozapine at 0.1 nM (Figure 3). When the preexposure was shortened to 10 minutes, the results were the same as the results when the competition method was used (data not shown). In other words, the prolonged exposure of the cloned D2 receptors to clozapine for 1 hour increased the D2 affinity for raclopride. Although the molecular basis of this effect is not known, G protein-linked receptors can interact and cooperate to exist as dimers or multimers with multiple states of affinity for an antagonist, as found for muscarinic receptors (75, 76) and dopamine D2 receptors (77). It is possible, therefore, that preexposure of D2 receptors to clozapine may alter the affinity state of D2 for raclopride, but such speculation must be directly tested in future experiments.
The present data (figures 1–5) are consistent with the time course of D2 occupancy measured clinically. For example, whereas the D2 occupancy by haloperidol in patients is long-lasting (78), the D2 occupancy by clozapine or by quetiapine in patients continues only for a matter of hours (7, 50).
Although the data from this study show that clozapine and quetiapine are readily released from D2, these data do not reveal the time course of release of these antipsychotic drugs from D2 under ordinary clinical conditions (i.e., in the absence of brain imaging experiments). However, the data in Figure 5, showing the rapid displacement of clozapine from D2 by 100 nM dopamine (which is the average level of dopamine in the synaptic space [79]) is consistent with the hypothesis that these antipsychotic drugs are displaced from the patient’s D2 receptors by endogenous dopamine (reviewed in reference 54), thereby contributing to the early reemergence of psychotic symptoms (1–11).
Additional data (not shown) revealed that an extremely high concentration of dopamine (30 µM) displaced 50% of [3H]quetiapine in 4 seconds, 50% of [3H]clozapine in 9 seconds, and 50% of [3H]haloperidol in 7 minutes; the result with [3H]haloperidol is in excellent agreement with the previously known value of 7.5 minutes for the time needed for 50% dissociation of haloperidol from the D2 receptor (80). Lower concentrations of dopamine displace [3H]clozapine more slowly, as just mentioned. The physicochemical factors determining the “loose binding” properties of quetiapine and clozapine are not known, although, presumably, the oil/water (or membrane/buffer) partition coefficients of these drugs are important.
Remoxipride has a K value of 30 nM, close to the values of 44 nM for clozapine and 78 nM for quetiapine (Figure 7), yet it occupies about 70% of D2(56). As summarized in Figure 7, however, it appears that the region between 30 and 40 nM for the antipsychotic dissociation constant is a transition region, above which displacement of the antipsychotic drug appears to occur, but below which displacement of the antipsychotic does not appear to occur. Melperone, however, has a dissociation constant of about 50 nM (54) yet occupies 70% of D2 receptors (56). One of the problems in analyzing the action of melperone is that it has an active metabolite, FG5155 (81), which is also an antagonist at D2 receptors (unpublished data), complicating the interpretation of the pharmacology involved.
The present data, indicating that [3H]clozapine binds loosely to the D2 receptor, are also consistent with the observation that clozapine occupies more D2 receptors in the cerebral cortex of patients than in the striatum (82). That is, the higher output of endogenous dopamine in the striatum readily displaces more D2-bound clozapine in the striatum, compared with the lower output of dopamine in the cerebral cortex (54).
The summary in Figure 7 omits the important drug risperidone. The risperidone dose range of 2–6 mg/day occupies between 60% and 80% of D2 receptors, with “mild” parkinsonism occurring in patients taking 6 mg/day and having D2 occupancies higher than 75% (35, 37, 44, , 83, 84). Such extrapyramidal signs, however, are generally low or negligible in patients taking 2–4 mg/day of risperidone and having D2 occupancies between 60% and 75% (35, 37, 44, , 83, 84).
The observations in the present study (figures 1–4) also illustrate the principle that D2 occupancy by an antipsychotic drug depends on the radioligand used (53, 54). Other studies have also shown that a radioligand should have a lower affinity than the drug for which a site’s occupancy is being probed (85–88). PET receptor occupancy studies are relevant to clinical realities, but technical aspects must be recognized before results can be usefully interpreted.
In conclusion, with respect to the apparently low D2 occupancy of clozapine, it may not be that “less is better” for the patient (89), but rather that “loose is better.”
Received July 22, 1998; revisions received Oct. 26 and Nov. 30, 1998; accepted Dec. 10, 1998. From the Departments of Psychiatry and Pharmacology, University of Toronto. Address reprint requests to Dr. Seeman, Departments of Psychiatry and Pharmacology, Medical Science Building, Rm. 4344, University of Toronto, 8 Taddle Creek Road, Toronto, Ont., Canada M5S 1A8; [email protected] (e-mail). Supported by Mr. and Mrs. R. Peterson; the Ontario Mental Health Foundation; the Medical Research Council of Canada; the Medland family; the National Institute on Drug Abuse; the Stanley Foundation of the National Alliance for the Mentally Ill Research Institute; Eli Lilly Canada, Inc.; and Zeneca Pharmaceuticals. Dr. Tallerico has been supported by a fellowship of the Medical Research Council of Canada in partnership with the Schizophrenia Society of Canada. The authors thank Dr. H.-C. Guan, Prof. Jim Wells, Dr. Shitij Kapur, Prof. Alan Wilson, Dr. B. Küfferle, and Dr. L. Pilowsky for comments and discussion; Lilly Research Laboratories for providing [ 3H]olanzapine; Astra Arcus AB for providing [3H]remoxipride; and H. Lundbeck A/S for providing [3H]sertindole.
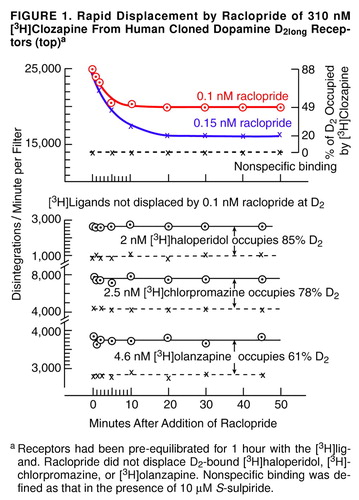
FIGURE 1. Rapid Displacement by Raclopride of 310 nM [3H]Clozapine From Human Cloned Dopamine D2long Receptors (top)a
aReceptors had been pre-equilibrated for 1 hour with the [3H]ligand. Raclopride did not displace D2-bound [3H]haloperidol, [3H]- chlorpromazine, or [3H]olanzapine. Nonspecific binding was defined as that in the presence of 10 µM S-sulpiride.
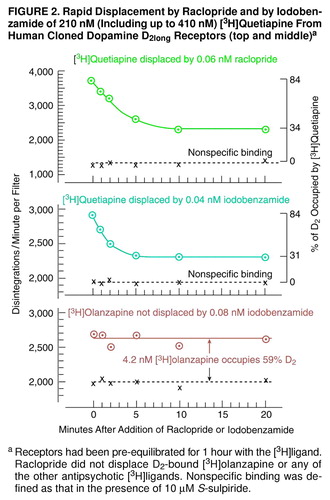
FIGURE 2. Displacement by Raclopride Concentrations Between 0.07 and 0.15 nM of D2-Bound [ 3H]Clozapine (310 nM) Within 10 Minutesa
aLower concentrations of raclopride (0.03–0.09 nM) displaced D2-bound [3H]quetiapine (210 nM) within 10 minutes. Much higher concentrations of raclopride were needed to compete with these ligands when the conventional competition method was used. The dashed vertical lines indicate the raclopride concentration range occurring in the plasma water of patients undergoing positron emission tomography.
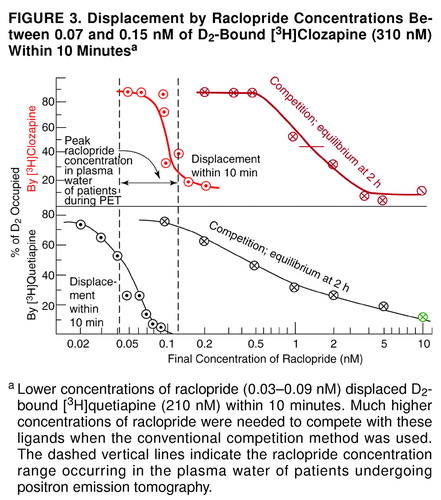
FIGURE 3. Displacement by Iodobenzamide Concentrations Between 0.02 and 0.1 nM of D2-Bound [ 3H]Clozapine (310 nM) and D2-Bound [ 3H]Quetiapine (210 nM) Within 10 Minutesa
aSomewhat higher concentrations of iodobenzamide were needed to compete with these ligands when the conventional competition method was used. The dashed vertical lines indicate the iodobenzamide concentration range occurring in the plasma water of patients undergoing single photon emission computed tomography.
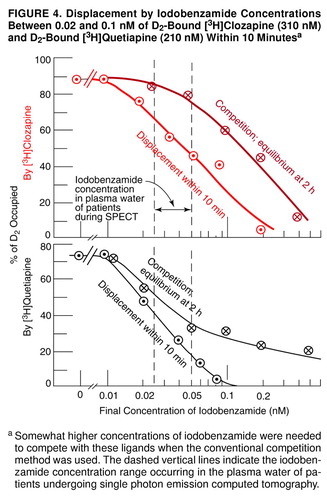
FIGURE 4. Rapid Displacement by Raclopride and by Iodobenzamide of 210 nM (Including up to 410 nM) [3H]Quetiapine From Human Cloned Dopamine D2long Receptors (top and middle)a
aReceptors had been pre-equilibrated for 1 hour with the [3H]ligand. Raclopride did not displace D2-bound [3H]olanzapine or any of the other antipsychotic [3H]ligands. Nonspecific binding was defined as that in the presence of 10 µM S-sulpiride.
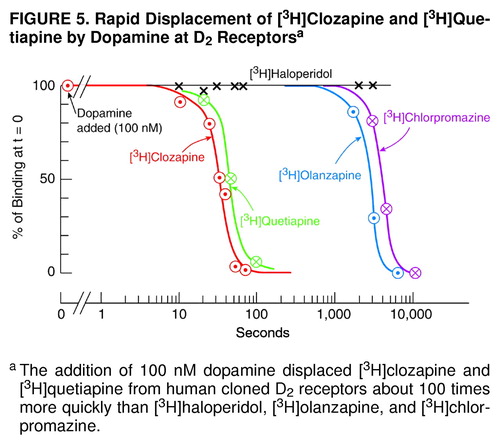
FIGURE 5. Rapid Displacement of [3H]Clozapine and [3H]Quetiapine by Dopamine at D2 Receptorsa
aThe addition of 100 nM dopamine displaced [3H]clozapine and [3H]quetiapine from human cloned D2 receptors about 100 times more quickly than [3H]haloperidol, [3H]olanzapine, and [3H]chlorpromazine.
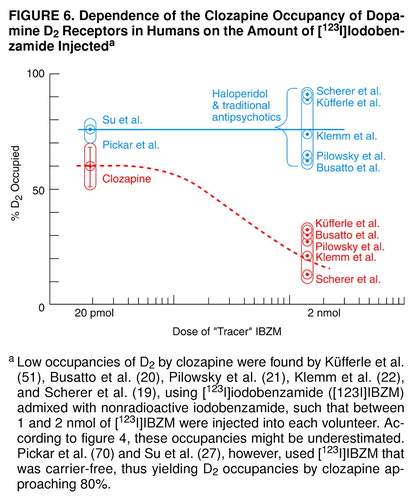
FIGURE 6. Dependence of the Clozapine Occupancy of Dopamine D2 Receptors in Humans on the Amount of [123I]Iodobenzamide Injecteda
aLow occupancies of D2 by clozapine were found by Küfferle et al. (51), Busatto et al. (20), Pilowsky et al. (21), Klemm et al. (22), and Scherer et al. (19), using [123I]iodobenzamide ([123I]IBZM) admixed with nonradioactive iodobenzamide, such that between 1 and 2 nmol of [123I]IBZM were injected into each volunteer. According to figure 4, these occupancies might be underestimated. Pickar et al. (70) and Su et al. (27), however, used [123I]IBZM that was carrier-free, thus yielding D2 occupancies by clozapine approaching 80%.
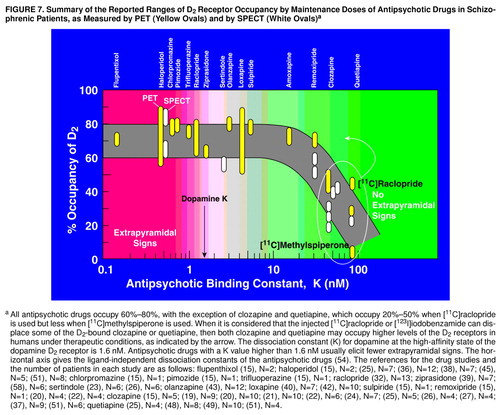
FIGURE 7. Summary of the Reported Ranges of D2 Receptor Occupancy by Maintenance Doses of Antipsychotic Drugs in Schizophrenic Patients, as Measured by PET (Yellow Ovals) and by SPECT (White Ovals)a
aAll antipsychotic drugs occupy 60%–80%, with the exception of clozapine and quetiapine, which occupy 20%–50% when [11C]raclopride is used but less when [11C]methylspiperone is used. When it is considered that the injected [11C]raclopride or [123I]iodobenzamide can displace some of the D2-bound clozapine or quetiapine, then both clozapine and quetiapine may occupy higher levels of the D2 receptors in humans under therapeutic conditions, as indicated by the arrow. The dissociation constant (K) for dopamine at the high-affinity state of the dopamine D2 receptor is 1.6 nM. Antipsychotic drugs with a K value higher than 1.6 nM usually elicit fewer extrapyramidal signs. The horizontal axis gives the ligand-independent dissociation constants of the antipsychotic drugs (54). The references for the drug studies and the number of patients in each study are as follows: flupenthixol (15), N=2; haloperidol (15), N=2; (25), N=7; (36), N=12; (38), N=7; (45), N=5; (51), N=8; chlorpromazine (15), N=1; pimozide (15), N=1; trifluoperazine (15), N=1; raclopride (32), N=13; ziprasidone (39), N=7; (58), N=6; sertindole (23), N=6; (26), N=6; olanzapine (43), N=12; loxapine (40), N=7; (42), N=10; sulpiride (15), N=1; remoxipride (15), N=1; (20), N=4; (22), N=4; clozapine (15), N=5; (19), N=9; (20), N=10; (21), N=10; (22), N=6; (24), N=7; (25), N=5; (26), N=4; (27), N=4; (37), N=9; (51), N=6; quetiapine (25), N=4; (48), N=8; (49), N=10; (51), N=4.
1. Chouinard G, Jones BD, Annable L: Neuroleptic-induced supersensitivity psychosis. Am J Psychiatry 1978; 135:1409–1410Google Scholar
2. Ekblom B, Eriksson K, Lindström LH: Supersensitivity psychosis in schizophrenic patients after sudden clozapine withdrawal. Psychopharmacology (Berl) 1984; 83:293–294Crossref, Medline, Google Scholar
3. Parsa MA, Al-Lahham YH, Ramirez LF, Meltzer HY: Prolonged psychotic relapse after abrupt clozapine withdrawal. J Clin Psychopharmacol 1993; 13:154–155Crossref, Medline, Google Scholar
4. De Leon J, Henighan V, Stanilla JK, Simpson GM: Clozapine levels after clozapine discontinuation. J Clin Psychopharmacol 1996; 16:193–194Crossref, Medline, Google Scholar
5. Dickson R, Williams R, Dalby JT: Dystonic reaction and relapse with clozapine discontinuation and risperidone initiation (letter). Can J Psychiatry 1994; 39:184Crossref, Medline, Google Scholar
6. Lacey RL, Preskorn SH, Jerkovich GS: Is risperidone a substitute for clozapine for patients who do not respond to neuroleptics? (letter). Am J Psychiatry 1995; 152:1401Medline, Google Scholar
7. Shore D: Clinical implications of clozapine discontinuation: report of an NIMH workshop. Schizophr Bull 1995; 21:333–338Crossref, Medline, Google Scholar
8. Shiovitz TM, Welke TL, Tigel PD, Anand R, Hartman RD, Sramek JJ, Kurtz NM, Cutler NR: Cholinergic rebound and rapid onset psychosis following abrupt clozapine withdrawal. Schizophr Bull 1996; 22:591–595Crossref, Medline, Google Scholar
9. Meltzer HY, Lee MA, Ranjan R, Mason EA, Cola PA: Relapse following clozapine withdrawal: effect of neuroleptic drugs and cyproheptadine. Psychopharmacology (Berl) 1996; 124:176–187Crossref, Medline, Google Scholar
10. Tanríverdi N, Yazící KM: Immediate recurrence of psychotic symptoms after discontinuation of clozapine: a case report. Biol Psychiatry 1996; 39:814–815Crossref, Medline, Google Scholar
11. Stanilla JK, de Leon J, Simpson GM: Clozapine withdrawal resulting in delirium with psychosis: a report of three cases. J Clin Psychiatry 1997; 58:252–255Crossref, Medline, Google Scholar
12. Thyrum PT, Yeh C, Potkin SG, Maguire G, Bera R, Carreon D, Arvanitis LA: Safety and tolerability of switching from conventional antipsychotic therapy to “Seroquel” (quetiapine fumarate) followed by abrupt withdrawal from “Seroquel.” Am College of Neuropsychopharmacology Abstracts 1997; 36:293Google Scholar
13. Gilbert PL, Harris MJ, McAdams LA, Jeste DV: Neuroleptic withdrawal in schizophrenic patients. Arch Gen Psychiatry 1995; 52:173–188Crossref, Medline, Google Scholar
14. Davis JM: Overview: maintenance therapy in psychiatry, I: schizophrenia. Am J Psychiatry 1975; 132:1237–1245Google Scholar
15. Farde L, Nordström A-L: PET analysis indicates atypical central dopamine receptor occupancy in clozapine-treated patients. Br J Psychiatry 1992; 160(suppl 17):30–33Google Scholar
16. Nordström A-L, Farde L, Halldin C: High 5-HT2 receptor occupancy in clozapine-treated patients demonstrated by PET. Psychopharmacology (Berl) 1993; 110:365–367Crossref, Medline, Google Scholar
17. Farde L, Nordström A-L, Nyberg S, Halldin C, Sedvall G: D1-, D2-, and 5-HT2-receptor occupancy in clozapine-treated patients. J Clin Psychiatry 1994; 55(Sept suppl B):67–69Google Scholar
18. Nordström A-L, Farde L, Nyberg S, Karlsson P, Halldin C, Sedvall G: D1, D2, and 5-HT2 receptor occupancy in relation to clozapine serum concentration: a PET study of schizophrenic patients. Am J Psychiatry 1995; 152:1444–1449Google Scholar
19. Scherer J, Tatsch K, Schwarz J, Oertel WH, Konjarczyk M, Albus M: D2-dopamine receptor occupancy differs between patients with and without extrapyramidal side effects. Acta Psychiatr Scand 1994; 90:266–268Crossref, Medline, Google Scholar
20. Busatto GF, Pilowsky LS, Costa DC, Ell PJ, Verhoeff LPLG, Kerwin RW: Dopamine D2 receptor blockade in vivo with the novel antipsychotics risperidone and remoxipride—an 123I-IBZM single photon emission tomography (SPET) study. Psychopharmacology (Berl) 1995; 117:55–61Crossref, Medline, Google Scholar
21. Pilowsky LS, Busatto GF, Taylor M, Costa DC, Sharma T, Sigmundsson T, Ell PJ, Nohria V, Kerwin RW: Dopamine D2 receptor occupancy in vivo by the novel atypical antipsychotic olanzapine—a 123I IBZM single photon emission tomography (SPET) study. Psychopharmacology (Berl) 1996; 124:148–153Crossref, Medline, Google Scholar
22. Klemm E, Grünwald F, Kasper S, Menzel C, Broich K, Danos P, Reichmann K, Krappel C, Rieker O, Briele B, Hotze AL, Möller H-J, Biersack H-J: [123I]IBZM SPECT for imaging of striatal D2 dopamine receptors in 56 schizophrenic patients taking various neuroleptics. Am J Psychiatry 1996; 153:183–190Link, Google Scholar
23. Kasper S, Tauscher J, Küfferle B, Barnas C, Hesselmann B, Asenbaum S, Podreka I, Brücke T: Sertindole and dopamine D2 receptor occupancy in comparison to risperidone, clozapine and haloperidol. Psychopharmacology (Berl) 1998; 136:367–373Crossref, Medline, Google Scholar
24. Su T-P, Breier A, Coppola R, Hadd K, Elman I, Alder C, Malhotra AK, Watsky E, Gorey J, Hough CJ, Weinberger D, Pickar D: D2 receptor occupancy during risperidone and clozapine treatment in chronic schizophrenia: relationship to blood level, efficacy and EPS. Abstracts of the Society for Neuroscience 1996; 22:265Google Scholar
25. Tauscher J, Küfferle B, Barnas C, Asenbaum S, Brücke T, Kasper S: Dopamine-2 receptor imaging in psychotic patients treated with quetiapine, clozapine, risperidone and haloperidol (abstract). Biol Psychiatry 1997; 42(suppl):15SGoogle Scholar
26. Kasper S, Tauscher J, Küfferle B, Asenbaum S, Barnas C, Hesselmann B, Podreka I, Brücke T: Sertindole and dopamine D2 receptor occupancy (abstract). Biol Psychiatry 1997; 42(suppl):161SGoogle Scholar
27. Su T-P, Malhotra AK, Hadd K, Breier A, Pickar D: D2 dopamine receptor occupancy: a crossover comparison of risperidone with clozapine therapy in schizophrenic patients. Arch Gen Psychiatry 1997; 54:972–973Crossref, Medline, Google Scholar
28. Schlösser R, Schlegel S, Hiemke C, Nickel O, Bockish A, Rao ML, Hahn K: [123I[IBZM SPECT in patients treated with typical and atypical neuroleptics: relationship to drug plasma levels and extrapyramidal side effects. Psychiatry Res Neuroimaging 1997; 75:103–114Crossref, Medline, Google Scholar
29. Farde L, Wiesel F-A, Halldin C, Sedvall G: Central D2-dopamine receptor occupancy in schizophrenic patients treated with antipsychotic drugs. Arch Gen Psychiatry 1988; 45:71–76Crossref, Medline, Google Scholar
30. Farde L, Wiesel F-A, Nordström A-L, Sedvall G: D1- and D2-dopamine receptor occupancy during treatment with conventional and atypical neuroleptics. Psychopharmacology (Berl) 1989; 99(suppl):S28–S31Google Scholar
31. Farde L, Pauli S, Hall H, Eriksson L, Halldin C, Högberg T, Nilsson L, Sjögren I, Stone-Elander S: Stereoselective binding of 11C-raclopride in living human brain—a search for extrastriatal central D2-dopamine receptors by PET. Psychopharmacology (Berl) 1988; 94:471–478Crossref, Medline, Google Scholar
32. Nordström A-L, Farde L, Wiesel F-A, Forslund K, Pauli S, Halldin C, Uppfeldt G: Central D2-dopamine receptor occupancy in relation to antipsychotic drug effects: a double-blind PET study of schizophrenic patients. Biol Psychiatry 1993; 33:227–235Crossref, Medline, Google Scholar
33. Nyberg S, Farde L, Eriksson L, Halldin C, Eriksson B:5-HT2 and D2 dopamine receptor occupancy in the living human brain. Psychopharmacology (Berl) 1993; 110:265–272Google Scholar
34. Farde L, Nyberg S, Nordström A-L, Halldin C, Sedvall G: Dopamine and serotonin receptor occupancy examined by PET in antipsychotic drug treated patients. Am College of Neuropsychopharmacology Abstracts 1993; 32:38Google Scholar
35. Farde L, Nyberg S, Oxenstierna G, Nakashima Y, Halldin C, Ericsson B: Positron emission tomography studies on D2 and 5-HT2 receptor binding in risperidone-treated schizophrenic patients. J Clin Psychopharmacol 1995; 15(suppl 1):19S–23SGoogle Scholar
36. Nyberg S, Nordström A-L, Halldin C, Farde L: Positron emission tomography studies on D2 dopamine receptor occupancy and plasma antipsychotic drug levels in man. Int Clin Psychopharmacol 1995; 10(suppl 3):81–85Google Scholar
37. Kapur S, Remington G, Zipursky RB, Wilson AA, Houle S: The D2 dopamine receptor occupancy of risperidone and its relationship to extrapyramidal symptoms: a PET study. Life Sci 1995; 57:103–107Crossref, Medline, Google Scholar
38. Kapur S, Remington G, Jones C, Wilson A, DaSilva J, Houle S, Zipursky R: High levels of dopamine D2 receptor occupancy with low-dose haloperidol treatment: a PET study. Am J Psychiatry 1996; 153:948–950Link, Google Scholar
39. Miceli JJ, Gunn KP, Rubin RH, Frackowiak RSJ, Williams SA, Fischman A:5HT2 and D2 receptor occupancy of ziprasidone in healthy volunteers. Am College of Neuropsychopharmacology Abstracts 1996; 35:269Google Scholar
40. Kapur S, Zipursky RB, Jones C, Remington GJ, Wilson AA, DaSilva J, Houle S: The D2 receptor occupancy profile of loxapine determined using PET. Neuropsychopharmacology 1996; 15:562–566Crossref, Medline, Google Scholar
41. Farde L, Mack RJ, Nyberg S, Halldin C: D2 occupancy, extrapyramidal side effects and antipsychotic drug treatment: a pilot study with sertindole in healthy subjects. Int Clin Psychopharmacology (Berl) 1997; 12(suppl 1):S3–S7Google Scholar
42. Kapur S, Zipursky R, Remington G, Jones C, McKay G, Houle S: PET evidence that loxapine is an equipotent blocker of 5-HT2 and D2 receptors: implications for the treatment of schizophrenia. Am J Psychiatry 1997; 154:1525–1529Google Scholar
43. Kapur S, Zipursky RB, Remington G, Jones C, DaSilva J, Wilson AA, Houle S:5-HT2 and D2 receptor occupancy of olanzapine in schizophrenia: a PET investigation. Am J Psychiatry 1998; 155:921–928Google Scholar
44. Remington G, Kapur S, Zipursky R: The relationship between risperidone plasma levels and dopamine D2 occupancy: a position emission tomographic study. J Clin Psychopharmacol 1998; 18:82–83Crossref, Medline, Google Scholar
45. Knable MB, Heinz A, Coppola R, Corey J, Weinberger DR: IBZM SPECT measurement of D2 receptor occupancy by haloperidol and risperidone (abstract). Biol Psychiatry 1996; 39:515Crossref, Google Scholar
46. Knable MB, Heinz A, Raedler T, Weinberger DR: Extrapyramidal side effects with risperidone and haloperidol at comparable D2 receptor occupancy levels. Psychiatry Res Neuroimaging 1997; 75:91–101Crossref, Medline, Google Scholar
47. Raedler T, Knable MB, Lafargue T, Pickar D, Weinberger DR: Striatal dopamine D2 receptor occupancy in patients treated with olanzapine. Abstracts of the Society for Neuroscience 1997; 23:560Google Scholar
48. Gefvert O, Lindstrom LH, Langstrom B, Bergstrom M, Lundberg T, Yates RA, Larsson SD, Tuersley MD: Time course for dopamine and serotonin receptor occupancy in the brain of schizophrenic patients following dosing with 150 mg Seroquel (ICI 204,636) TID. Am College of Neuropsychopharmacology Abstracts 1995; 34:276Google Scholar
49. Gefvert O, Lundberg T, Wieselgren I-M, Hagström P, Bergström M, Långström B, Wiesel F-A, Yates R, Lindström LH: D2 and 5HT2A receptor binding of different doses of quetiapine in schizophrenics: a PET study. Am College of Neuropsychopharmacology Abstracts 1997; 36:168Google Scholar
50. Gefvert O, Bergström M, Långström B, Lundberg T, Lindström L, Yates R: Time course of central nervous dopamine-D2 and 5-HT2 receptor blockade and plasma drug concentrations after discontinuation of quetiapine (Seroquel) in patients with schizophrenia. Psychopharmacology (Berl) 1998; 135:119–126Crossref, Medline, Google Scholar
51. Küfferle B, Tauscher J, Asenbaum S, Vesely C, Podreka I, Brücke T, Kasper S: IBZM SPECT imaging of striatal dopamine-2 receptors in psychotic patients treated with the novel antipsychotic substance quetiapine in comparison to clozapine and haloperidol. Psychopharmacology (Berl) 1997; 133:323–328Crossref, Medline, Google Scholar
52. Tauscher J, Küfferle B, Asenbaum S, Brücke T, Kasper S: Previous treatment as a confounding variable in studies with novel antipsychotics: two cases of high dopamine-2 receptor occupancy with quetiapine. Psychopharmacology (Berl) 1997; 133:102–105Crossref, Medline, Google Scholar
53. Seeman P, Corbett R, Van Tol HHM: Atypical neuroleptics have low affinity for dopamine D2 receptors or are selective for D4. Neuropsychopharmacology 1997; 16:93–110Crossref, Medline, Google Scholar
54. Seeman P, Tallerico T: Antipsychotic drugs which elicit little or no parkinsonism bind more loosely than dopamine to brain D2 receptors, yet occupy high levels of these receptors. Mol Psychiatry 1998; 3:123–134Crossref, Medline, Google Scholar
55. Farde L, Wiesel F-A, Jansson P, Uppfeldt A, Wahlen A, Sedvall G: An open label trial of raclopride in acute schizophrenia: confirmation of D2-dopamine receptor occupancy by PET. Psychopharmacology (Berl) 1988; 94:1–7Crossref, Medline, Google Scholar
56. Farde L, Nordström A-L, Wiesel F-A, Pauli S, Halldin C, Sedvall G: Positron emission tomographic analysis of central D1 and D2 dopamine receptor occupancy in patients treated with classical neuroleptics and clozapine: relation to extrapyramidal side effects. Arch Gen Psychiatry 1992; 49:538–544Crossref, Medline, Google Scholar
57. Farde L, Hall H, Pauli S, Halldin C: Variability in D2-dopamine receptor density and affinity: a PET study with [11C]raclopride in man. Synapse 1995; 20:200–208Crossref, Medline, Google Scholar
58. Bench CJ, Lammertsma AA, Grasby PM, Dolan RJ, Warrington SJ, Boyce M, Gunn KP, Brannick LY, Frackowiak RSJ: The time course of binding to striatal dopamine D2 receptors by the neuroleptic ziprasidone (CP-88,059-01) determined by positron emission tomography. Psychopharmacology (Berl) 1996; 124:141–147Crossref, Medline, Google Scholar
59. Kapur S, Zipursky R, Roy P, Jones C, Remington G, Reed K, Houle S: The relationship between D2 receptor occupancy and plasma levels on low dose oral haloperidol: a PET study. Psychopharmacology (Berl) 1997; 131:148–152Crossref, Medline, Google Scholar
60. Volkow ND, Fowler JS, Wang GJ, Dewey SL, Schlyer D, MacGregor R, Logan J, Alexoff D, Shea C, Hitzmann R, Angrist B, Wolf AP: Reproducibility of repeated measures of carbon-11-raclopride binding in the human brain. J Nucl Med 1993; 34:609–613Medline, Google Scholar
61. Farde L, Hall H, Ehrin E, Sedvall G: Quantitative analysis of D2 dopamine receptor binding in the living human brain by PET. Science 1986; 231:258–261Crossref, Medline, Google Scholar
62. Luabeya MK, Maloteaux JM, Laduron PM: Regional and cortical laminar distribution of serotonin S2, benzodiazepine, muscarinic and dopamine D2 receptors in human brain. J Neurochem 1984; 43:1068–1071Google Scholar
63. Seeman P, Guan H-C, Van Tol HHM: Dopamine D4 receptors elevated in schizophrenia. Nature 1993; 365:441–445Crossref, Medline, Google Scholar
64. Farde L, Hall H, Paull S, Halldin C: Variability in D2-dopamine receptor density and affinity: a PET study with [11C]raclopride in man. Synapse 1995; 20:200–208Crossref, Medline, Google Scholar
65. Pilowsky LS, Costa DC, Ell PJ, Murray RM, Verhoeff NPLG, Kerwin RW: Clozapine, single photon emission tomography, and the D2 dopamine receptor blockade hypothesis of schizophrenia. Lancet 1992; 340:199–202; correction, 340:380Crossref, Medline, Google Scholar
66. Bobeldijk M, Verhoeff NPLG, Vekemans JAJM, Buck HM, van Doremalen PAPM, van Hoof JJ, Janssen AGM: A simple and high-yield synthesis of (S)-BZM, (R)-BZM and (S)-IBZM for the preparation of (S)-123I-IBZM. J Labelled Compounds and Radiopharmaceuticals 1990; 28:1247–1256Google Scholar
67. Verhoeff NPLG, Bobeldijk M, Feenstra MGP, Boer GJ, Maas MAW, Erdtsieck-Ernste E, De Bruin K, Van Royen EA: In vitro and in vivo D2-dopamine receptor binding with [123I]S(–)iodobenzamide ([123I]IBZM) in rat and human brain. Nucl Med Biol 1991; 18:837–846Google Scholar
68. Seibyl JP, Woods SW, Zoghbi SS, Baldwin RM, Dey HM, Goddard AW, Zea-Ponce Y, Zubal G, Germine M, Smith EO, Heninger GR, Charney DS, Kung HF, Alavi A, Hoffer PB, Innis RB: Dynamic SPECT imaging of dopamine D2 receptors in human subjects with iodine-123-IBZM. J Nucl Med 1992; 33:1964–1971Google Scholar
69. Kung HF, Alavi A, Chang W, Kung M-P, Keyes JW Jr, Velchik MG, Billings J, Pan S, Noto R, Rausch A, Reilley J: In vivo SPECT imaging of CNS D-2 dopamine receptors: initial studies with iodine-123-IBZM in humans. J Nucl Med 1990; 31:573–579Medline, Google Scholar
70. Pickar D, Su T-P, Weinberger DR, Coppola R, Malhotra AK, Knable MB, Lee KS, Gorey J, Bartko JJ, Breier A, Hsiao J: Individual variation in D2 dopamine receptor occupancy in clozapine-treated patients. Am J Psychiatry 1996; 153:1571–1578Google Scholar
71. Farde L, Wiesel F-A, Stone-Elander S, Halldin C, Nordström A-L, Hall H, Sedvall G: D2 dopamine receptors in neuroleptic-naive schizophrenic patients. Arch Gen Psychiatry 1990; 47:213–219Crossref, Medline, Google Scholar
72. Seeman P, Van Tol HHM: Deriving the therapeutic concentrations for clozapine and haloperidol: the apparent dissociation constant of a neuroleptic at the dopamine D2 or D4 receptor varies with the affinity of the competing radioligand. Eur J Pharmacol 1995; 291:59–66Crossref, Medline, Google Scholar
73. Seeman P, Corbett R, Nam D, Van Tol HHM: Dopamine and serotonin receptors: amino acid sequences, and clinical role in neuroleptic parkinsonism. Jpn J Pharmacol 1996; 71:187–204Crossref, Medline, Google Scholar
74. Seeman P: Therapeutic receptor-blocking concentrations of neuroleptics. Int Clin Psychopharmacol 1995; 10(suppl 3):5–13Google Scholar
75. Wreggett KA, Wells JW: Cooperativity manifest in the binding properties of purified cardiac muscarinic receptors. J Biol Chem 1995; 270:22488–22499Google Scholar
76. Chidiac P, Green MA, Pawagi AB, Wells JW: Cardiac muscarinic receptors: cooperativity as the basis for multiple states of affinity. Biochemistry 1997; 36:7361–7379Google Scholar
77. Ng G, O’Dowd BF, Lee SP, Chung HT, Brann MR, Seeman P, George SR: Dopamine D2 receptor dimers and receptor-blocking peptides. Biochem Biophys Res Commun 1996; 227:200–204Crossref, Medline, Google Scholar
78. Nordström A-L, Farde L, Halldin C: Time course of D2-dopamine receptor occupancy examined by PET after single oral doses of haloperidol. Psychopharmacology (Berl) 1992; 106:433–438Crossref, Medline, Google Scholar
79. Kawagoe KT, Garris PA, Wiedemann DJ, Wightman RM: Regulation of transient dopamine concentration gradients in the microenvironment surrounding nerve terminals in the rat striatum. Neuroscience 1992; 1:55–64Crossref, Google Scholar
80. Leysen JE, Gommeren W: The dissociation rate of unlabelled dopamine antagonists and agonists from the dopamine-D2 receptor, application of an original filter method. J Receptor Res 1984; 4:817–845Crossref, Medline, Google Scholar
81. Wiesel F-A, Bjerkenstedt L, Skett P: Effect of melperone, two of its metabolites and thiothixene on central monoamine metabolism and prolactin levels in rodents. Acta Pharmacol Toxicol 1978; 43:129–136Crossref, Medline, Google Scholar
82. Pilowsky LS, Mulligan RS, Acton PD, Ell PJ, Costa DC, Kerwin RW: Limbic selectivity of clozapine. Lancet 1997; 350:490–491Crossref, Medline, Google Scholar
83. Nyberg S, Eriksson B, Oxenstierna G, Halldin C, Farde L: PET study of D2- and 5-HT2 receptor occupancy induced by risperidone in schizophrenic patients. Am College of Neuropsychopharmacology Abstracts 1996; 35:184Google Scholar
84. Kapur S, Zipursky RB, Remington G: Clinical and theoretical implications of 5-HT2 and D2 receptor occupancy of clozapine, risperidone, and olanzapine in schizophrenia. Am J Psychiatry 1999; 156:286–293Abstract, Google Scholar
85. Gatley SJ, Volkow ND, Chen R, Fowler JS, Carroll FI, Kuhar MJ: Displacement of RTI-55 from the dopamine transporter by cocaine. Eur J Pharmacol 1996; 296:145–151Crossref, Medline, Google Scholar
86. Votaw JR, Kessler RM, De Paulis T: Failure of the three-compartment model to describe the pharmacokinetics in brain of a high-affinity substituted benzamide. Synapse 1993; 15:177–190Crossref, Medline, Google Scholar
87. Fowler JS, Volkow ND, Logan J, Gatley SJ, Pappas N, King P, Ding YS, Wang GJ: Measuring dopamine transporter occupancy by cocaine in vivo: radiotracer considerations. Synapse 1998; 28:111–116Crossref, Medline, Google Scholar
88. Gifford AN, Gatley SJ, Volkow ND: Evaluation of the importance of rebinding to receptors in slowing the approach to equilibrium of high-affinity PET and SPECT radiotracers. Synapse 1998; 28:167–175Crossref, Medline, Google Scholar
89. Carpenter WT Jr, Zito JM, Vitrai J, Volavka J: Hypothesis testing: is clozapine’s superior efficacy dependent on moderate D2 receptor occupancy? Biol Psychiatry 1989; 43:79–83Google Scholar