Prediction of Dopamine Transporter Binding Availability by Genotype: A Preliminary Report
Abstract
OBJECTIVE: Evidence of a relationship between genotype and binding availability was assessed for the dopamine and serotonin transporter genes. METHOD: The authors assessed dopamine transporter genotype at the SLC6A3 3′ variable number of tandem repeats (VNTR) polymorphism and serotonin transporter genotype at the SLC6A4 promotor VNTR polymorphism in 30 healthy subjects who also underwent single photon emission computed tomography with [123I]β-CIT. RESULTS: Subjects homozygous for the 10-repeat allele at the SLC6A3 locus demonstrated significantly lower dopamine transporter binding than carriers of the nine-repeat allele. There was no effect of SLC6A4 genotype upon serotonin transporter binding. CONCLUSIONS: These findings suggest that genetic variation at the SLC6A3 3′ VNTR polymorphism may modify dopamine transporter function.
Several polymorphisms of the human dopamine transporter gene (locus: SLC6A3) and serotonin transporter gene (locus: SLC6A4) have been described. At SLC6A3, there is a variable number of tandem repeats (VNTR) polymorphism in the 3′ untranslated region, with repeat numbers ranging from three to 11 (1); allelic variation at this locus has been found to be associated with several psychiatric disorders (2, 3). The promotor region of SLC6A4 contains a VNTR polymorphism with two common alleles differing in length by 44 base pairs; these have been designated as long (16 repeats) and short (14 repeats) according to their relative size (4). In vitro measures have demonstrated that the long allele has higher transcriptional activity than the short allele (4).
The few human studies that have examined the relationship between genetic polymorphisms at these loci and binding availability of the dopamine and serotonin transporter proteins they encode have produced inconsistent results. One postmortem study of suicide victims and comparison subjects found increased serotonin transporter binding in subjects homozygous for the short allele (5). A second postmortem study of midbrain serotonin transporter binding found that ethanol-using carriers of the short allele had higher binding in the dorsal raphe than did comparison subjects who carried the short allele (6). When ethanol users were excluded, heterozygotes had lower serotonin transporter binding than homozygotes for either the short or the long allele (6). In vivo data from healthy and alcohol-dependent subjects have suggested that 1) among healthy subjects, serotonin transporter binding is higher among those homozygous for the long allele than for carriers of the short allele and 2) among those homozygous for the long allele, serotonin transporter binding is lower in alcohol-dependent subjects than in healthy subjects (7). Putamen dopamine transporter binding was lower in subjects heterozygous at the SLC6A3 locus (i.e., who carried a 9/10-repeat allele) than in subjects homozygous for the 10-repeat allele (8).
In the present study, the relationship between SLC6A3 and SLC6A4 genotype and dopamine and serotonin transporter binding availability, respectively, was examined by using [123I]2-carbomethoxy-3-(4-iodophenyl)tropane ([123I]β-CIT) and single photon computed tomography (SPECT). Previous work has shown that striatal [123I]β-CIT binding is displaced by the selective dopamine transporter inhibitor GBR 12909 but not by the selective serotonin transporter inhibitors citalopram or paroxetine, whereas the reverse is true for binding of this ligand in diencephalon and brainstem (9, 10). These findings indicate that striatal [123I]β-CIT binding reflects dopamine transporter binding availability, while diencephalic and brainstem [123I]β-CIT binding reflects serotonin transporter binding availability. On the basis of earlier work (8), we hypothesized that at SLC6A3, subjects homozygous for the 10-repeat allele would show higher dopamine transporter binding availability than carriers of the nine-repeat allele and that at SLC6A4, subjects homozygous for the long allele would demonstrate greater serotonin transporter binding availability than carriers of the short allele.
Method
Subject assessment included the Structured Clinical Interview for DSM-IV Axis I Disorders (11), physical and neurologic examination, routine laboratory testing, and urine toxicology to rule out medical, neurologic, and psychiatric illness, including drug abuse or dependence. Thirty healthy subjects participated (mean age=37.0 years, SD=9.3). Four were African American, 26 were European American, and 13 were female.
All subjects provided written informed consent for participation in this study, which was approved by the Yale and VA Connecticut Healthcare System institutional review boards.
Genomic DNA was extracted from whole blood, and the polymerase chain reaction was used to amplify the variable segment of the 3′ untranslated region of the SLC6A3 gene (1). Amplification was performed by using the primers T7-3aLong (CTT CCT GGA GGT CAC GGC TCA AGG) and James5 (AG GAA ATT CTG TTT ATG TTC TTG), which amplify a segment 91 base pairs longer than that amplified by other primers (1). The number of repeats contained in each allele, determined from polymerase chain reaction product size, was reflected in the numeric designation of each allele. The SLC6A4 promotor VNTR polymorphism was genotyped as described previously (12). Alleles were designated according to their relative size: short (14 repeats) and long (16 repeats).
SPECT scanning was performed 21.7 hours (SD=1.4) after injection of 6.0 mCi (SD=0.2) of [123I]β-CIT (13). A Picker PRISM 3000 camera (Picker International, Inc., Cleveland) was used to obtain simultaneous acquisition of a transmission scan and a 15-minute emission scan, followed by a 24-minute emission scan. Emission scans were acquired in continuous mode (128 × 128 matrix, 3.56-mm slice thickness, 120° angular range, 3° angular step, 40 steps, 36 seconds per step, and a 15.5-cm radius of rotation). An axial magnetic resonance imaging (MRI) scan (acquisition matrix=256 × 192, number of excitations=1, flip angle=45°, TE=5 msec, TR=24 msec, field of view=24 cm2, slice thickness=3.0 mm) was acquired from all subjects.
SPECT emission scans were reconstructed from photo peak counts (20% energy window centered on 159 kiloelectron volts) by using a Butterworth filter (power factor=10, cutoff=0.24 cycles per second). Transmission scans were reconstructed by using a Bayesian algorithm and a smoothing Gibbs prior (14). The emission images were coregistered, and the same three-dimensional transformation applied to the transmission image. Nonuniform attenuation correction of the second emission image was performed (14). MRI scans were coregistered to the SPECT transmission scan and reoriented such that the axial plane was parallel to the intercommissural plane. This three-dimensional transformation was applied to the emission image. The coregistered, reoriented MRI was used to guide placement of standardized two-dimensional region of interest templates for the right and left striatum (eight slices), diencephalon (four slices), brainstem (eight slices), and right and left cerebellum (six slices). Three dimensional volumes of interest were then created for striatum (sum of eight right and left striatal regions of interest, 9.5 cm each), diencephalon (sum of four slices, 1.9 cm3), brainstem (sum of eight slices, 3.7 cm3), and cerebellum (sum of six right and left cerebellar regions of interest, 43.2 cm3 each). The three-dimensional volumes of interest were transferred to the coregistered, reoriented SPECT emission scan to determine regional activity (counts per minute per pixel).
Scans from nine subjects measured by two raters (L.K.J. and J.K.S.) and remeasured by a single rater revealed inter- and intrarater reliabilities (intraclass correlation coefficients [15]), respectively, of 0.99 and 0.98 for striatal activity, 0.96 and 0.99 for diencephalic activity, 0.91 and 0.99 for brainstem activity, and 0.97 and 0.99 for cerebellar activity. All data were analyzed by a single rater who was blind to genotype. The cerebellum, which is nearly devoid of dopamine and serotonin transporters, was used as a measure of nondisplaceable uptake (13). Dopamine transporter binding availability (V3″) was estimated by using regional radioactivities measured from striatal and cerebellar volumes of interest in the following equation: V3″=(striatum – cerebellum)/cerebellum. Serotonin transporter binding availability was estimated by substituting, separately, diencephalic and brainstem for striatal regional radioactivities in this equation (9).
Dopamine and serotonin transporter binding availability tended to be inversely correlated with age (striatum: r=–0.40, df=28, p=0.03; diencephalon: r=–0.30, df=28, p=0.10; brainstem: r=–0.32, df=28, p=0.09). Therefore, the relationship between binding availability and dopamine and serotonin transporter genotype was examined by using analysis of covariance (ANCOVA), with genotype as the independent variable, [123I]β-CIT binding (at striatum for dopamine transporter binding and at diencephalon and brainstem for serotonin transporter binding) as the dependent variable, and age as the covariate.
Results
For one subject, polymerase chain reaction failed to amplify the variable segment of the 3′ untranslated region of the SLC6A3 gene. One subject carried a three-repeat and one an 11-repeat SLC6A3 allele. These subjects were excluded from statistical analyses. Only two subjects were homozygous for the nine-repeat allele; thus, homozygous and heterozygous carriers of the nine-repeat allele were grouped and compared to subjects homozygous for the 10-repeat allele.
ANCOVA, controlling for age, revealed no effect of SLC6A4 genotype upon serotonin transporter binding (diencephalic binding: short/short mean=2.2 [SD=0.4], short/long mean=2.2 [SD=0.3], long/long mean=2.3 [SD=0.4]; brainstem binding: short/short mean=1.0 [SD=0.2], short/long mean=1.0 [SD=0.2], long/long mean=0.9 [SD=0.2]). In contrast, SLC6A3 genotype exerted a significant effect upon dopamine transporter binding (Figure 1. ), with subjects homozygous for the 10-repeat allele (mean striatal binding=7.1, SD=1.0) demonstrating 13.4% lower striatal binding than carriers of the nine-repeat allele (mean striatal binding=8.2, SD=1.0). When race was added as a second covariate, the results were unchanged (F=8.78, df=1, 23, p=0.007).
Caudate and putamen volumes were quantified by using a previously described method (16) in 14 subjects from the present study group and 17 subjects from a separate group of cocaine-dependent patients who also underwent genotyping. This exploratory analysis revealed no evidence of an effect of SLC6A3 genotype on volume of caudate or putamen (repeated measures ANCOVA with diagnosis and age as covariates; F values [df=1, 27] ranged from 0.04 to 0.46), which suggests that the observed effect of genotype on dopamine transporter binding availability was not mediated by an effect of genotype on caudate or putamen morphology.
Discussion
In this examination of the relationship between SLC6A3 and SLC6A4 genotype and binding availability of their corresponding products, the dopamine and serotonin transporters, subjects homozygous for the 10-repeat allele at the SLC6A3 locus demonstrated significantly lower dopamine transporter binding than carriers of the nine-repeat allele. Evidence supporting a relationship between genotype at the SLC6A4 locus and serotonin transporter binding availability was not observed. Discrepancies between the present findings and those from previous work may stem from effects of postmortem interval or from differences in the quality of psychiatric and pharmacological history that can be ascertained in in vivo versus postmortem studies. In addition, differences in subject groups studied, methods for controlling effects of diagnosis and previous drug exposure, and methods of image analysis may have contributed to differences in the findings observed (5–8).
Limitations of this study include small group size, which precluded our ability to examine potential interactions between race, SLC6A3 allele frequency (which varies in frequency across race [17]), and dopamine transporter binding availability. Other limitations include the poor spatial resolution of SPECT and use of a radiotracer that has both dopamine and serotonin transporter binding capability. Although previous work has demonstrated specific displacement of [123I]β-CIT in striatum by dopamine transporter-binding pharmaceuticals and in diencephalon and brainstem by serotonin transporter-binding pharmaceuticals (9, 10), a dopamine transporter contribution to brainstem [123I]β-CIT binding, particularly in the region of the substantia nigra, cannot be completely ruled out.
If replicated in a larger sample, with a higher resolution imaging modality, such as positron emission tomography, and a radiotracer with greater dopamine transporter specificity, these findings would suggest that the SLC6A3 3" VNTR may be in linkage disequilibrium with another polymorphism that modifies dopamine transporter function or that it may itself affect gene regulation. If replicated, the observed relationship between SLC6A3 genotype and dopamine transporter binding availability could be used to predict dopamine transporter binding availability in neuropsychiatric disorders associated with alleles at this locus. For example, given that a number of studies have demonstrated an association between the 10-repeat SLC6A3 allele and attention deficit hyperactivity disorder (ADHD) (3, 18–20), dopamine transporter binding availability could be expected to be lower in patients with ADHD relative to age- and population-matched healthy subjects. This would be consistent with the mouse dopamine transporter gene knockout—which has been considered a model of ADHD, since the animals exhibit spontaneous hyperlocomotion (21)—and would suggest that decreased dopamine transporter function or expression may render children vulnerable to the development of ADHD.
Received Jan. 27, 2000; revision received April 13, 2000; accepted April 20, 2000. From the Department of Psychiatry, Yale University School of Medicine, New Haven, Conn., and the VA Connecticut Healthcare System. Address correspondence to Dr. Jacobsen, Psychiatry 116A, VA Connecticut Healthcare System, 950 Campbell Ave., West Haven, CT 06516; [email protected] (e-mail).Supported in part by grants from NIH (RR-00125), NIMH (MH-01387), the National Institute on Alcohol Abuse and Alcoholism (AA-11330), and the National Institute on Drug Abuse (DA-00167, DA-04060, DA-10219, and DA-09250) and by the U.S. Department of Veterans Affairs (the VA Medical Research Program and the VA Connecticut–Massachusetts Mental Illness Research, Education and Clinical Center).The authors thank Jonathan S. Feinstein, Ph.D., for statistical consultation, Louis A. Amici for radiotracer synthesis, Quinn Ramsby for computer support, Ann Marie Lacobelle and Roy Capper for technical assistance, and Drs. Nora D. Volkow and Andreas Heinz for their comments on an earlier draft of this article.
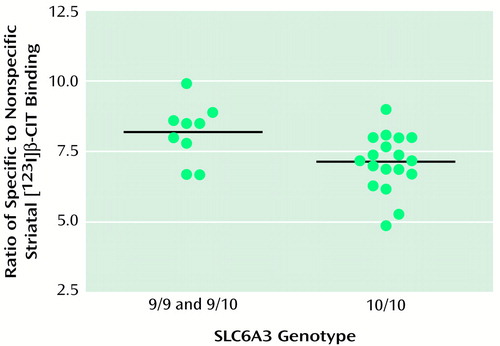
Figure 1. Ratio of Specific to Nonspecific Striatal [123I]β-CIT Binding by SLC6A3 Genotype in 27 Healthy Subjectsa
aStriatal [123I]β-CIT binding reflects dopamine transporter binding availability, which was significantly lower for subjects who were homozygous for the 10-repeat allele at the SLC6A3 locus than for homozygous and heterozygous carriers of the nine-repeat allele (F=9.43, df=1, 24, p=0.005).
1. Vandenbergh DJ, Persico AM, Hawkins AL, Griffin CA, Li X, Jabs EW, Uhl GR: Human dopamine transporter gene (DAT1) maps to chromosome 5p15.3 and displays a VNTR. Genomics 1992; 14:1104–1106Google Scholar
2. Gelernter J, Kranzler HR, Satel SL, Rao PA: Genetic association between dopamine transporter protein alleles and cocaine-induced paranoia. Neuropsychopharmacology 1994; 11:195–200Crossref, Medline, Google Scholar
3. Cook EH, Stein MA, Krasowski MD, Cox NJ, Olkon DM, Kieffer JE, Leventhal BL: Association of attention-deficit disorder and the dopamine transporter gene. Am J Hum Genet 1995; 56:993–998Medline, Google Scholar
4. Lesch KP, Bengel D, Heils A, Sabol SZ, Greenberg B, Petri S, Benjamin J, Muller CR, Hamer DH, Murphy DL: Association of anxiety-related traits with a polymorphism in the serotonin transporter gene regulatory region. Science 1996; 274:1527–1531Google Scholar
5. Du L, Faludi G, Palkovits M, Demeter E, Bakish D, Lapierre YD, Sotonyi P, Hrdina PD: Frequency of long allele in serotonin transporter gene is increased in depressed suicide victims. Biol Psychiatry 1999; 46:196–201Crossref, Medline, Google Scholar
6. Little KY, McLaughlin DP, Zhang L, Livermore CS, Dalack GW, McFinton PR, DelProposto ZS, Hill E, Cassin BJ, Watson SJ, Cook EH: Cocaine, ethanol, and genotype effects on human midbrain serotonin transporter binding sites and mRNA levels. Am J Psychiatry 1998; 155:207–213Link, Google Scholar
7. Heinz A, Jones D, Mazzanti C, Goldman D, Ragan P, Hommer D, Linnoila M, Weinberger DR: A relationship between serotonin transporter genotype and in vivo protein expression and alcohol neurotoxicity. Biol Psychiatry 2000; 47:643–649Crossref, Medline, Google Scholar
8. Heinz A, Goldman D, Jones DW, Palmour R, Hommer D, Gorey JG, Lee KS, Linnoila M, Weinberger DR: Genotype influences in vivo dopamine transporter availability in human striatum. Neuropsychopharmacology 2000; 22:133–139Crossref, Medline, Google Scholar
9. Laruelle M, Baldwin RM, Malison RT, Zea-Ponce Y, Zoghbi SS, Al-Tikriti M, Sybirska EH, Zimmermann RC, Wisniewski G, Neumeyer JL, Milius RA, Wang S, Smith EO, Roth RH, Charney DS, Hoffer PB, Innis RB: SPECT imaging of dopamine and serotonin transporter with [123I]β-CIT: pharmacological characterization of brain uptake in nonhuman primates. Synapse 1993; 13:295–309Crossref, Medline, Google Scholar
10. Scheffel U, Dannals RF, Cline EJ, Ricaurte GA, Carroll FI, Abraham P, Lewin AH, Kuhar MJ: [123/125I]RTI-55, an in vivo label for the serotonin transporter. Synapse 1992; 11:134–139Crossref, Medline, Google Scholar
11. First MB, Spitzer RL, Gibbon M, Williams JBW: Structured Clinical Interview for DSM-IV Axis I Disorders (SCID). New York, New York State Psychiatric Institute, Biometrics Research, 1997Google Scholar
12. Gelernter J, Kranzler H, Cubells JF: Serotonin transporter protein (SLC6A4) allele and haplotype frequencies and linkage disequilibria in African- and European-American and Japanese populations and in alcohol dependent subjects. Hum Genet 1997; 101:243–246Crossref, Medline, Google Scholar
13. Laruelle M, Wallace E, Seibyl JP, Baldwin RM, Zea-Ponce Y, Zoghbi SS, Neumeyer JL, Charney DS, Hoffer PB, Innis RB: Graphical, kinetic and equilibrium analyses of in vivo [123I]β-CIT binding to dopamine transporters in healthy human subjects. J Cereb Blood Flow Metab 1994; 14:982–994Crossref, Medline, Google Scholar
14. Rajeevan N, Zubal IG, Ramsby SQ, Zoghbi SS, Seibyl J, Innis RB, Zoghbi S: Significance of nonuniform attenuation correction in quantitative brain SPECT imaging. J Nucl Med 1998; 39:1719–1726Google Scholar
15. Fleiss JL: The Design and Analysis of Clinical Experiments. New York, John Wiley & Sons, 1986Google Scholar
16. Giedd JN, Snell JW, Lange N, Rajapakse JC, Casey BJ, Kozuch PL, Vaituzis AC, Vauss YC, Hamburger SD, Kaysen D, Rapoport JL: Quantitative magnetic resonance imaging of human brain development: ages 4–18. Cereb Cortex 1996; 6:551–560Crossref, Medline, Google Scholar
17. Min Kang A, Palmatier MA, Kidd KK: Global variation of a 40-bp VNTR in the 3′-untranslated region of the dopamine transporter gene (SLC6A3). Biol Psychiatry 1999; 46:151–160Crossref, Medline, Google Scholar
18. Daly G, Hawi Z, Fitzgerald M, Gill M: Mapping susceptibility loci in attention deficit hyperactivity disorder: preferential transmission of parental alleles at DAT1, DBH and DRD5 to affected children. Mol Psychiatry 1999; 4:192–196Crossref, Medline, Google Scholar
19. Gill M, Daly G, Heron S, Hawi Z, Fitzgerald M: Confirmation of association between attention deficit hyperactivity disorder and a dopamine transporter polymorphism. Mol Psychiatry 1997; 2:311–313Crossref, Medline, Google Scholar
20. Waldman ID, Rowe DC, Abramowitz A, Kozel ST, Mohr JH, Sherman SL, Cleveland HH, Sanders ML, Gard JMC, Stever C: Association and linkage of the dopamine transporter gene and attention-deficit hyperactivity disorder in children: heterogeneity owing to diagnostic subtype and severity. Am J Hum Genet 1998; 63:1767–1776Google Scholar
21. Giros B, Jaber M, Jones SR, Wightman RM, Caron MG: Hyperlocomotion and indifference to cocaine and amphetamine in mice lacking the dopamine transporter. Nature 1996; 379:606–612Crossref, Medline, Google Scholar