Association of Choroid Plexus Enlargement With Cognitive, Inflammatory, and Structural Phenotypes Across the Psychosis Spectrum
Abstract
Objective:
The choroid plexus is an important physiological barrier and produces CSF and neurotrophic, angiogenic, and inflammatory factors involved in brain development. Choroid plexus abnormalities have been implicated in both schizophrenia and bipolar disorder. A previous choroid plexus transcriptomic analysis of schizophrenia identified an upregulation of immune and inflammatory genes that correlated with peripheral inflammatory markers. The purpose of this study was to examine choroid plexus volume in probands across the psychosis spectrum and in their first-degree and axis II cluster A relatives, as well as choroid plexus familiality and choroid plexus covariance with clinical, cognitive, brain, and peripheral marker measures.
Methods:
Choroid plexus volume was quantified (using FreeSurfer) in psychosis probands, their first-degree and axis II cluster A relatives, and healthy control subjects, organized by DSM-IV-TR diagnosis. Analyte, structural connectivity, and genotype data were collected from a subset of study subjects.
Results:
Choroid plexus volume was significantly larger in probands compared with first-degree relatives or healthy control subjects; first-degree relatives had intermediate enlargement compared with healthy control subjects; and total choroid plexus volume was significantly heritable. Larger volume was associated with worse cognition, smaller total gray matter and amygdala volume, larger lateral ventricle volume, and lower structural connectivity in probands. Associations between larger volume and higher levels of interleukin 6 in probands was also observed.
Conclusions:
These findings suggest the involvement of the choroid plexus across the psychosis spectrum with a potential pathophysiological mechanism involving the neuroimmune axis, which functions in maintaining brain homeostasis and interacting with the peripheral immune and inflammatory system. The choroid plexus may be an important target in future research.
The choroid plexus has been largely ignored in clinical neuroscience, but recent evidence suggests that it plays several important roles in brain homeostasis. The choroid plexus is located in the lateral, third, and fourth ventricles and produces CSF. It consists of an epithelial cell monolayer, which forms a physical barrier called the blood-CSF barrier, and a stromal compartment containing permeable fenestrated blood vessels as well as immune, pluripotent, hematopoietic, neuronal progenitor, and telocyte cells (1, 2). The choroid plexus epithelium limits the paracellular passage via tight junctions and allows intercellular communication through gap junctions (3). Transport across the blood-CSF barrier is tightly controlled, involving both active and passive transport, which plays an important role in cell division, migration, differentiation, and synaptogenesis during early brain development (4, 5). The blood-CSF barrier can function as an enzymatic barrier with the ability to conjugate drugs and other proteins, assisting in elimination and providing protection against oxidative stress (6). The blood-CSF barrier is also an immunological barrier with resident immune cells that can modulate leukocyte migration, produce proinflammatory cytokines, and express major histocompatibility complex molecules as well as molecules for leukocyte adhesion (6). Lastly, the lateral ventricle choroid plexus secretes chemokines, lymphokines, growth factors, carriers, and remodelers that promote the proliferation of neural stem cells (1, 7–9), and the choroid plexus epithelium transcriptome specifically functions in molecular transport, neurological disease processes, immunological functions, ciliary movement, hematopoiesis, and angiogenesis (10). While our understanding of the role of the choroid plexus in brain homeostasis is growing, much less is known about its role in psychotic disorders, despite the intersection between choroid plexus function and the neurodevelopmental, neurodegenerative, and neuroimmune hypotheses in psychosis pathophysiology.
Choroid plexus abnormalities have been discussed as a possible pathological feature in schizophrenia and bipolar disorder beginning with Morowoka and Kitabayashi in 1921, when morphological changes in choroid plexus epithelium (smaller and longer cells), vascular endothelium degeneration, large cystic formations, and cellular fat depositions in choroid plexus epithelium cells were identified in schizophrenia and evidence of choroid plexus epithelium hypersecretion and degeneration of vascular epithelium and connective tissue were found in individuals with bipolar mania compared with healthy control subjects (11, 12). However, despite these findings, the role of the choroid plexus in psychosis has been largely ignored. More recently, case reports have identified an association between worse cognition (memory difficulties and inattention), mood (depression, anhedonia, lethargy, isolation, and suicidal ideation), and psychosis (persecutory delusions, ideas of reference, auditory hallucinations, hostility, blunted affect, poverty of speech, emotional or social withdrawal, and motor retardation) with fourth-ventricle choroid plexus papilloma or third-ventricle tumor, with full symptom remission after tumor resection (13–15). Moreover, cross-sectional studies using CT brain imaging have identified an association between the presence of choroid plexus calcification and schizophrenia neurobiology (16–19); however, to our knowledge, no brain MRI studies examining choroid plexus volume in schizophrenia have been published. Previous imaging studies have identified an association between increased choroid plexus calcification size and greater hallucination intensity, worse formal thought disorder, regional brain volume reductions (frontal, temporal, parietal, hippocampal, and cerebellar), and larger ventricular brain ratio at the frontal horns, but less is known about negative symptoms or cognitive dysfunction (16, 18, 19). For schizophrenia, a transcriptomic analysis of the choroid plexus found that a coexpression module of immune and inflammation genes was upregulated, and this module was significantly associated with disease status and positively correlated with C-reactive protein (CRP), cortisol, and proinflammatory cytokines in the serum and frontal cortex in the same individuals (20). These findings suggest that the increased size of the choroid plexus may be involved in psychosis phenomenology (positive, negative, and cognitive symptoms), neurobiology (regional volumetric reductions), and neuroimmune axis connection, but more research is needed to further our understanding of the role of the choroid plexus across the psychosis spectrum.
In this study, we sought to investigate 1) choroid plexus volume in probands across the spectrum of psychosis, their first-degree and axis II cluster A relatives, and healthy control subjects, 2) choroid plexus familiality between probands and their first-degree relatives, and 3) choroid plexus covariance with clinical, brain, and peripheral marker measures using data from the cross-sectional Bipolar-Schizophrenia Network on Intermediate Phenotypes (B-SNIP) consortium. We hypothesized that choroid plexus volume would be increased in psychosis and be heritable and that increased volume would be associated with worse psychosis symptoms and cognition, correlated with regional brain atrophy, and related to increased levels of peripheral inflammatory markers.
Methods
Participants
A subset of participants for whom FreeSurfer analyses could be completed were included from the B-SNIP multisite study, which included Harvard University, Wayne State University, the Maryland Psychiatric Research Center, the University of Chicago, the University of Texas Southwestern Medical Center at Dallas, and the Institute of Living at Yale University. The study protocol was approved by the institutional review board at each site. Recruited individuals were probands with a diagnosis of schizophrenia, schizoaffective disorder, or psychotic bipolar I disorder, at least one first-degree relative of each proband, and healthy control subjects (21). (For details on inclusion criteria, see the online supplement.) Written informed consent was obtained from all participants.
The Structured Clinical Interview for DSM-IV was administered to all participants, and a consensus diagnosis was made using clinical interviews, medical records, and psychiatric histories. Relatives were assessed with the Structured Interview for DSM-IV Personality Disorders to evaluate personality traits specifically relevant to the psychosis spectrum, represented by cluster A personality disorders (21). Antipsychotic drugs were categorized into first-generation and second-generation antipsychotic classes, and average daily dosages were converted to chlorpromazine equivalents (Table 1). Probands were assessed with the Positive and Negative Syndrome Scale (PANSS) and the Montgomery-Åsberg Depression Rating Scale (MADRS). Cognition was evaluated using the Brief Assessment of Cognition in Schizophrenia battery (22), and all scores were adjusted for age and sex and z-transformed to a range of ±4.0. The Birchwood Social Functioning Scale was used to capture social functioning in all groups. For group sample size by clinical measure, see Table S1 in the online supplement.
Characteristic | Healthy Control Subjects (N=333) | Psychosis Probands (N=554) | First-Degree Relatives (N=522) | Analysis | |||||
---|---|---|---|---|---|---|---|---|---|
N | % | N | % | N | % | χ2 | df | p | |
Female | 184 | 55.3 | 287 | 51.8 | 364 | 69.7 | 38.7 | 2 | <0.001* |
Race | 20.8 | 4 | <0.001* | ||||||
African American | 88 | 26.4 | 206 | 37.2 | 146 | 28 | |||
Caucasian | 215 | 64.6 | 313 | 56.5 | 350 | 67 | |||
Other | 30 | 9 | 35 | 6.3 | 26 | 5 | |||
Antipsychotic | |||||||||
First-generation | 48 | 8.6 | |||||||
Second-generation | 406 | 73.2 | |||||||
None | 90 | 16.2 | |||||||
Mean | SD | Mean | SD | Mean | SD | F | df | p | |
Antipsychotic daily dose (chlorpromazine equivalents) | — | — | 446.9 | 390.5 | — | — | — | — | |
Age (years) | 37.0 | 12.4 | 35.5 | 12.5 | 40.8 | 15.6 | 20.6 | 2, 1406 | <0.001* |
Duration of illness (years) | — | — | 17.9 | 12.2 | — | — | — | — | |
Age at onset (years) | — | — | 17.6 | 7.7 | — | — | — | — | |
PANSS total score | — | — | 62.9 | 16.9 | — | — | — | — | |
Intracranial volume (mm3) | 1,431,124.5 | 191,440.9 | 143,5747.3 | 184,991.4 | 1,406,2519 | 179,218.8 | 3.79 | 2, 1406 | 0.023* |
TABLE 1. Demographic and clinical characteristics of psychosis probands, first-degree relatives, and healthy control subjectsa
Structural MRI
Participants were scanned at all six sites, and T1-MPRAGE images were processed using FreeSurfer 6.0 (for details on the imaging protocol, see the online supplement). Forty-seven participants were removed as a result of scanner inhomogeneity and motion that interfered with segmentation. Participants with more than four standard deviations (N=6) and between the third and fourth standard deviation (N=19) were removed and winsorized to the third standard deviation, respectively. To examine the relationship between choroid plexus volume and the dysconnectivity hypothesis in psychosis, we conducted an exploratory analysis of diffusion-weighted images to extract the average tissue fractional anisotropy in a subset of study subjects (probands, N=131; first-degree relatives, N=140 [see also the online supplement]). Healthy control subjects were not included in this analysis given the small size of this subsample (N=42).
Because few studies have examined choroid plexus volume using FreeSurfer, two raters (P.L. and O.L.) established reliability using manual ratings for the scans. The choroid plexus for 24 raw scans (four per site) was randomly and blindly manually rated for small, medium, and large volumes. The raters had a high intraclass correlation coefficient for their ratings (0.79) and moderate Spearman correlations with FreeSurfer volume (r=0.44, p<0.01).
Plasma Analyte Assays
Blood samples from the Chicago site were obtained from 107 probands with psychosis (psychotic bipolar I disorder, N=48; schizoaffective disorder, N=23; schizophrenia, N=36) and 58 healthy control subjects at baseline and were batched, spun at 4°C, divided into 300 μL aliquots, and stored at −80°C. The plasma concentrations of inflammatory and neurotrophic (IL-1β, IL-2, IL-4, IL-6, IL-8, IL-10, IL-12, IL-12p70, IFNγ, TNFα, CRP, sFlt-1, VEGF, TGFβ) markers were determined using Meso Scale Discovery MULTI-ARRAY technology. These analytes were selected on the basis of their implication in schizophrenia and bipolar disorder (23–29). For details regarding analyte quality control, see the methods section in the online supplement. For a breakdown of the number of study subjects assayed by analyte and by group, see Table S1 in the online supplement.
Statistical Analysis
Descriptive statistics were computed for demographic variables across groups, and differences were examined using the chi-square test or analysis of variance. Pairwise contrasts using analysis of covariance to assess choroid plexus volume were run on probands, first-degree relatives, axis II cluster A family members, and healthy control subjects. An exploratory analysis of the diagnostic groups (schizophrenia, schizoaffective disorder, and psychotic bipolar I disorder) compared with healthy control subjects was performed to determine whether a particular group was driving the effect.
Effect sizes were calculated using Cohen’s d. Partial correlations for clinical, cognitive, analyte, and neuroimaging measures were analyzed using the Spearman correlation (nonnormal distribution for several variables). Calculated p values were false discovery rate-corrected for multiple comparisons (q value), and this was performed for each hypothesis tested, as well as for exploratory analyses. All data were adjusted for age, sex, race, and site. Imaging data were additionally adjusted for total intracranial volume. To examine whether choroid plexus volume was determined by total lateral ventricle volume or total gray matter volume, we performed choroid plexus group comparisons using total lateral ventricle volume and total lateral ventricle volume plus total gray matter volume instead of intracranial volume as covariates to regress out the effects of cortical atrophy and ventricular enlargement. Analyte data were additionally adjusted for storage days. All statistical analyses were performed using R (version 3.4.3).
Familiality for total choroid plexus volume was quantified in 340 probands and 464 of their first-degree relatives using Sequential Oligogenic Linkage Analysis Routines, version 8.1.4, with a maximum likelihood method. Significance was determined by comparing a model explaining phenotypic variation by family membership with a model assuming that family membership has no variation. Familiality estimates were calculated using a polygenic model, and choroid plexus volume was covaried for age, sex, race, site, and intracranial volume.
Results
Demographic Characteristics
Our final sample included 554 probands (diagnostic groups: schizophrenia, N=223; schizoaffective disorder, N=141; psychotic bipolar I disorder, N=190), 522 first-degree relatives of probands, 42 axis II cluster A relatives, and 333 healthy control subjects (Table 1; see also Table S5 in the online supplement). The probands, family members, and healthy control subjects differed significantly on age, sex, race, and intracranial volume (Table 1). The three diagnostic groups showed no differences on age but were significantly (p<0.001) different on sex, race, duration of illness, age at illness onset, and intracranial volume (for further details, see Table S2 in the online supplement). PANSS total scores were in the moderate range (>58 and ≤75) for the proband group (Table 1), in the mild (≤58) and moderate ranges for the three diagnostic groups, and significantly different between the diagnostic groups (for further details, see Table S2 in the online supplement) (30). Daily chlorpromazine equivalents were not significantly different between the diagnostic groups (for further details, see Table S2 in the online supplement). Many of the probands were taking second-generation antipsychotic drugs (N=406), few were taking first-generation antipsychotics (N=48), none were taking both first- and second-generation antipsychotics, and the remaining were not taking any antipsychotic medications (N=90) (Table 1).
Choroid Plexus Volumetric Differences
Probands had significantly larger bilateral choroid plexus volume compared with healthy control subjects (q<0.001, Cohen’s d=0.335 and 0.301 for the left and right, respectively) (Figure 1A; see also Tables S3 and S4 in the online supplement), and these results remained significant after covarying for lateral ventricle volume (q<0.05, Cohen’s d=0.193 and 0.162 for the left and right, respectively) and lateral ventricle volume plus total gray matter volume (q<0.05, Cohen’s d=0.188 and 0.181 for the left and right, respectively). Probands had significantly greater bilateral choroid plexus volume compared with first-degree relatives (left choroid plexus: q≤0.001, Cohen’s d=0.226; right choroid plexus: q<0.001, Cohen’s d=0.205) (Figure 1A); however, these results were no longer significant after covarying for lateral ventricle volume with or without total gray matter volume (see Table S4 in the online supplement). There were no significant choroid plexus volume differences between first-degree relatives and healthy control subjects (Figure 1A). Probands showed a nonsignificant increase in the left choroid plexus volume (Cohen’s d=0.254) compared with axis II cluster A relatives, and axis II cluster A relatives demonstrated a nonsignificant bilateral enlargement (Cohen’s d=0.09–0.25) compared with healthy control subjects (see Table S5 in the online supplement).
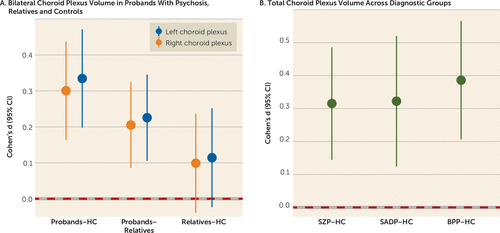
FIGURE 1. Effect sizes for choroid plexus volume comparisons in psychosis probands, relatives, and healthy control subjectsa
a Panel A shows pairwise contrasts of bilateral choroid plexus volume between psychosis probands, first-degree relatives, and healthy control (HC) subjects. Panel B shows comparisons of total choroid plexus volume in probands across diagnostic groups (schizophrenia [SZP], schizoaffective disorder [SADP], and psychotic bipolar I disorder [BPP]) compared with healthy control subjects. Solid circles indicate Cohen’s d, and solid lines indicate confidence intervals. Cohen’s d estimates were adjusted for age, sex, race, site, and intracranial volume.
Because greater choroid plexus volume was observed bilaterally in probands, subsequent analyses assessed total choroid plexus volume by summating the corresponding left and right choroid plexus volumes. All three diagnostic groups (schizophrenia, schizoaffective disorder, and psychotic bipolar I disorder) showed greater total volume compared with the healthy control group (diagnostic groups: q≤0.001, Cohen’s d=0.315–0.386) (Figure 1B; see also Table S4 in the online supplement). When including lateral ventricle volume as a covariate instead of intracranial volume, volumes in the schizophrenia and psychotic bipolar I disorder groups (Cohen’s d=0.192 and 0.252, respectively; see also Table S4 in the online supplement) remained significantly larger than volumes in the healthy control group (q<0.05), while volumes in the schizoaffective disorder group did not. A similar pattern was observed when including lateral ventricle and total gray mater volumes as covariates instead of intracranial volume (schizoaffective disorder: Cohen’s d=0.198; psychotic bipolar I disorder: Cohen’s d=0.259; see also Table S4 in the online supplement), which was significantly (q<0.05) different from volumes in healthy control subjects.
Total choroid plexus volume was found to be moderately heritable (log likelihood=−5,039.68, h2R=0.297, standard error=0.087, p<0.001, kurtosis 0.570).
Choroid Plexus Volume Correlations With Clinical Variables
The PANSS positive score and negative score, age at illness onset, and daily chlorpromazine equivalents showed no significant relationships in the proband group (for further details, see Table S6 in the online supplement). To address the dopamine and serotonin link to choroid plexus (31, 32), we explored the relationship of antipsychotic drugs that are predominantly dopamine antagonists (first-generation antipsychotics) compared with serotonin-dopamine antagonists (second-generation antipsychotics) with choroid plexus volume. There was no significant relationship (F=1.38, df=2, 531, p=0.25) between first-generation antipsychotic use (choroid plexus volume: mean=1,089.4, SD=304.2), second-generation antipsychotic use (choroid plexus volume, mean=1,141.9, SD=327.6), and no antipsychotic use (choroid plexus volume, mean=1,097.4, SD=329.5) in our study sample. The Birchwood Social Functioning Scale total score was not significantly associated with choroid plexus volume in probands, first-degree relatives of probands, or healthy control subjects (Figure 2A; see also Table S7 in the online supplement), as well as in axis II cluster A relatives (for further details, see Table S5 in the online supplement).
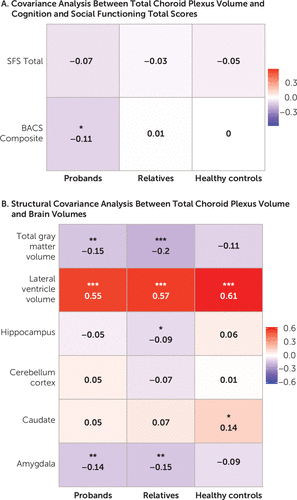
FIGURE 2. Choroid plexus volume correlations with clinical and brain structural measures in psychosis probands, first-degree relatives, and healthy control subjectsa
a Panel A shows partial correlations between choroid plexus volume and cognition (Brief Assessment of Cognition in Schizophrenia [BACS] composite score) and functioning (Birchwood Social Functioning Scale [SFS] total score). Panel B shows partial correlations between choroid plexus volume and total gray matter, lateral ventricle, hippocampus, cerebellum cortex, caudate, and amygdala volume. Partial correlations were adjusted for age, sex, race, and site. Imaging data were additionally adjusted for total intracranial volume. False discovery rate-corrected q-values are presented.
Choroid Plexus Volume Correlations With Cognition
Larger choroid plexus volume was significantly (r=−0.11, q<0.05) associated with worse composite scores on the Brief Assessment of Cognition in Schizophrenia among probands but not among family members or healthy control subjects (Figure 2A; see also Table S7 in the online supplement). For subcategories of the Brief Assessment of Cognition in Schizophrenia, among probands, significant associations between greater volume and lower performance in verbal fluency (r=−0.132, q<0.05) and symbol coding (r=−0.109, q<0.05) were observed but not for other subdomains or among healthy control subjects, first-degree relatives of probands (for further details, see Figure S1 and Table S7 in the online supplement), and axis II cluster A relatives (for further details, see Table S5 in the online supplement).
Choroid Plexus Volume Correlations With Brain Structure
Larger choroid plexus volume significantly (q<0.001) correlated with larger lateral ventricle volume in probands, first-degree relatives, and healthy control subjects (Figure 2B; see also Table S8 in the online supplement). Lower total gray matter volume showed a significant (q<0.01) association with larger choroid plexus volume in probands and first-degree relatives of probands. In addition, a significant association (q<0.01) was observed between smaller amygdala and greater choroid plexus volume in probands and first-degree relatives. A relationship between larger choroid plexus volume and smaller hippocampal volume (q<0.05) was observed in first-degree relatives. A significant correlation (q<0.05) between higher choroid plexus volume and higher caudate volume was observed in healthy control subjects (for further details, see Table S8 in the online supplement). A significant (q<0.05) positive relationship was observed between larger choroid plexus volume and larger lateral ventricle and caudate volume in axis II cluster A relatives (for further details, see Table S5 in the online supplement). No significant relationships were observed between choroid plexus volume and cerebellum cortex volume in any of the groups.
In examining the relationship between choroid plexus volume and brain structural connectivity, in a subset of our neuroimaging study sample, we observed that increased volume was significantly negatively associated with global fractional anisotropy (p=0.012) (Figure 3) in probands, but this relationship was not seen in first-degree relatives.
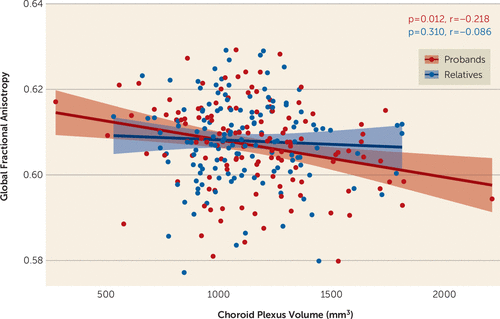
FIGURE 3. Choroid plexus volume correlations with free water-corrected global fractional anisotropy in psychosis probands and first-degree relativesa
a Partial correlations were adjusted for age, sex, race, and site. Choroid plexus volume was additionally adjusted for total intracranial volume.
Choroid Plexus Volume Correlations With Analytes
Elevations in interleukin-6 (IL-6) were associated (r=0.23, p=0.02, uncorrected) with larger choroid plexus volume in probands but not in healthy control subjects (Figure 4A and 4B; see also Table S9 in the online supplement). To test the effect of clinical condition, we examined diagnostic group differences and found that greater levels of IL-6 (r=0.31, p=0.04) and IL-10 (r=0.36, p=0.01) were associated with larger choroid plexus volume in the bipolar I disorder group, while greater IL-4 levels were associated with smaller choroid plexus volume (r=−0.30, p=0.04) in the healthy control group (see also Table S9 in the online supplement). No other significant correlations were observed between choroid plexus volume and remaining analytes in the proband, diagnostic, or healthy control group. To address whether IL-6 levels were associated with symptom severity, we computed Spearman correlations between IL-6 levels and either PANSS total score or MADRS total score, and no relationship was observed (r=0.059, p=0.542 and r=0.021, p=0.833, respectively).
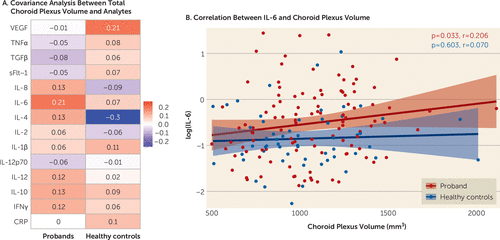
FIGURE 4. Choroid plexus volume partial correlations with analyte measures in psychosis probands and healthy control subjectsa
a Panel A shows partial correlations between choroid plexus volume and analytes. Panel B shows partial correlation between choroid plexus volume and log(IL-6) in probands and healthy control subjects. Partial correlations were adjusted for age, sex, and race, and p values were false discovery rate-corrected for multiple comparisons. Imaging data were additionally adjusted for total intracranial volume, and analyte data were additionally adjusted for sample storage days.
Discussion
We found that bilateral choroid plexus volume was significantly larger in probands across the psychosis spectrum (schizophrenia, schizoaffective disorder, and psychotic bipolar I disorder) compared with healthy control subjects and was independent of diagnostic category, that first-degree relatives had intermediate bilateral choroid plexus enlargement compared with healthy control subjects, and that total choroid plexus volume was significantly heritable. We also observed associations between larger choroid plexus volume and worse composite cognitive score, which was primarily driven by poorer performance on verbal fluency and digit symbol coding among probands. We did not observe any associations between choroid plexus size and positive or negative symptoms of psychosis or social functioning. Similarly, we established that greater choroid plexus volume was related to smaller total gray matter and amygdala volume, larger lateral ventricle volume, and lower structural connectivity in probands. Additionally, we uncovered an important association between larger choroid plexus volume and higher levels of IL-6 in probands. Our results support our hypothesis that choroid plexus enlargement exists across psychotic disorders and that it is related to clinical and neuroimaging phenotypes observed in psychosis, which include cognitive impairment, brain atrophy, brain structural dysconnectivity, and increased inflammation.
Our finding of greater choroid plexus volume in psychosis suggests that it may be a common trait shared across the psychosis spectrum. Because this is the first brain structural MRI study examining choroid plexus volume in psychotic disorders, estimates of choroid plexus structural alterations do not exist. However, postmortem studies of schizophrenia and bipolar disorder have described morphological changes in the choroid plexus, such as smaller and longer epithelial cells, large cystic formations, epithelial cellular fat depositions, and epithelial hypersecretion, which would suggest that choroid plexus enlargement is a pathological feature of psychosis (11). Additionally, evidence supporting this hypothesis has come from observations of enlargement as a result of tumor formation or calcifications, which have shown that increased size is associated with worse symptoms of cognition, mood, and psychosis, as well as a pattern of regional brain atrophy similar to that found in schizophrenia (13–19).
While we observed an increase in choroid plexus volume in psychosis, this information was not sufficient to enhance our understanding of the pathophysiological role of enlargement in psychosis, which could be hypothesized to include a neurodevelopmental, neurodegenerative, or neuroimmune variable or the effect of antipsychotic medications. In this study, we ruled out the effect of comorbidity of medication, such as antipsychotic dosage or antipsychotic class, on choroid plexus volume, and our finding was consistent with preclinical studies showing that infusion with dopamine or serotonin increases choroid plexus blood flow and that dopamine receptor blockade reduces flow but does not necessarily change the volume (31, 32). From a neurodevelopmental and neurodegenerative standpoint, we were not able to determine whether our volume finding was due to hypersecretion, decreased absorption, or other morphological changes because of limitations in structural brain imaging, which could explain the well-known finding of ventricular enlargement or cortical atrophy. However, we were able to show that choroid plexus volume was both independently and significantly increased in probands compared with healthy control subjects after controlling for lateral ventricle volume or lateral ventricle volume plus total gray matter volume. Lateral ventricle enlargement and cortical atrophy are well-established findings in schizophrenia and bipolar disorder, and our findings suggest that the choroid plexus is a more specific determinant of psychosis proneness and that it may contribute to lateral ventricle enlargement through a currently unknown mechanism. It remains to be determined whether choroid plexus enlargement is a cause or a consequence of psychosis, and this question could not be fully ascertained in our cross-sectional study. Thus, our findings suggest that choroid plexus alterations may be involved in psychosis, and it would be important for future studies to identify whether the choroid plexus plays a pathogenic role, is governed by ventricular enlargement via cortical atrophy, progressively increases over the course of illness, and is specific to psychosis by examining nonpsychotic bipolar I disorder or major depressive disorder groups.
Another important pathophysiologic mechanism involves the neuroimmune connection and its role in brain homeostasis. Thus, we examined the relationship between serum markers and choroid plexus volume, because it links peripheral signaling (inflammatory, angiogenic, and neurotrophic) to CNS molecular processes, which may contribute to the development and progression of psychotic disorders (20, 26, 33, 34). We found that increased IL-6 levels were significantly associated with larger volume. This finding is of interest, because the choroid plexus expresses the receptor for IL-6 (35) and, in the blood, IL-6 has pleiotropic activity, which induces the synthesis of acute-phase proteins (e.g., CRP), activates the acquired immune response by stimulating antibody production and effector T-cell development, and can promote the differentiation or proliferation of nonimmune cells (36). It is noteworthy that IL-6 elevations are strongly implicated in schizophrenia and bipolar pathophysiology (25) and that cortical gray matter reductions (assessed in postmortem samples) in schizophrenia are exaggerated in individuals with high expression of inflammatory cytokines (37). In further support of this hypothesis, a schizophrenia postmortem choroid plexus transcriptome study identified an immune-inflammation module that significantly and positively correlated with serum levels of CRP, which is downstream from IL-6 (20). Lastly, a preliminary study conducted by Zhou et al. (38) showed that choroid plexus volume was increased in patients with chronic regional pain syndrome compared with healthy control subjects, and the authors postulated that this finding was a result of inflammatory processes. While this novel finding may suggest that choroid plexus enlargement is a result of inflammatory pathways in any disorder rather than being specific to psychosis, we believe that the small sample size in the Zhou et al. study and the lateralization of their findings suggest that more work is needed before concluding that enlargement is specific to inflammatory activation in any disorder. Thus, IL-6 and CRP may play a role in stress-immune-related pathways leading to immune activation and vascular dysfunction that can have detrimental CNS effects (25). The positive relationship between choroid plexus volume and IL-6 provides further support for the choroid plexus linking the peripheral and CNS environments. However, additional work is needed to better understand the significance of peripheral immune and inflammatory markers in choroid plexus structural alterations.
The association between the choroid plexus neuroimmune axis and our brain structural and cognitive findings is further supported by our covariance analysis. Previous studies have shown that increased levels of inflammation are associated with reductions in cortical gray matter volume (37). Additionally, neuroinflammation has been associated with white matter pathology, and it may contribute to structural and functional dysconnectivity in psychosis (39). Lastly, animal studies have demonstrated that CD4+ T cells in the choroid plexus are responsible for cognitive functions, such as spatial learning and memory, and that these CD4+ T cells accumulate during mental stress (34). Taken together, the association between higher levels of IL-6 and increased choroid plexus volume could explain the association between greater choroid plexus volume and total gray matter thinning, amygdala atrophy, ventricular enlargement, and lower structural connectivity, and these changes may culminate in worse cognition. This axis may be triggered by mental stress, which would activate a neuroimmune cascade with significant downstream effects on cognition and brain structure.
Discussion of choroid plexus familiality findings is presented in the online supplement.
To our knowledge, this is the first study of alterations in choroid plexus volumes across the psychosis spectrum. A major strength of this study is an automated parcellation approach with high intraclass correlations between raters and moderate correlations with FreeSurfer choroid plexus volume. Another strength is the large, clinically well-characterized sample of patients across the psychosis spectrum. However, the subsample was not large enough to detect robust relationships with peripheral markers. Nevertheless, our results can inform future studies.
1 : The path from the choroid plexus to the subventricular zone: go with the flow! Front Cell Neurosci 2012; 6:34Crossref, Medline, Google Scholar
2 : Regulation of neural stem cells by choroid plexus cells population. Neurosci Lett 2016; 626:35–41Crossref, Medline, Google Scholar
3 : A new angle on blood-CNS interfaces: a role for connexins? FEBS Lett 2014; 588:1259–1270Crossref, Medline, Google Scholar
4 : Transporters of the blood-brain and blood-CSF interfaces in development and in the adult. Mol Aspects Med 2013; 34:742–752Crossref, Medline, Google Scholar
5 : A new look at cerebrospinal fluid circulation. Fluids Barriers CNS 2014; 11:10Crossref, Medline, Google Scholar
6 : The choroid plexus: a comprehensive review of its history, anatomy, function, histology, embryology, and surgical considerations. Childs Nerv Syst 2014; 30:205–214Crossref, Medline, Google Scholar
7 : Age-dependent niche signals from the choroid plexus regulate adult neural stem cells. Cell Stem Cell 2016; 19:643–652Crossref, Medline, Google Scholar
8 : Nerve growth factor and doublecortin expression correlates with improved outcome in children with severe traumatic brain injury. J Trauma 2008; 65:80–85Crossref, Medline, Google Scholar
9 : Distinct sites of insulin-like growth factor (IGF)-II expression and localization in lesioned rat brain: possible roles of IGF binding proteins (IGFBPs) in the mediation of IGF-II activity. Endocrinology 1999; 140:520–532Crossref, Medline, Google Scholar
10 : Gene expression-based comparison of the human secretory neuroepithelia of the brain choroid plexus and the ocular ciliary body: potential implications for glaucoma. Fluids Barriers CNS 2014; 11:2Crossref, Medline, Google Scholar
11 : A note on the pathology of the choroid plexus in general paralysis. Arch NeurPsych 1922; 7:177–182Crossref, Google Scholar
12 : The choroid plexus in organic diseases of the brain and of schizophrenia. J Nerv Ment Dis 1921; 56:21–26Google Scholar
13 : Choroid plexus papilloma presenting as schizophrenia: a case report. J Neuropsychiatry Clin Neurosci 2013; 25:E26–E27Crossref, Medline, Google Scholar
14 : Third ventricular choroid plexus papilloma with psychosis: case report. J Neurosurg 1997; 87:103–105Crossref, Medline, Google Scholar
15 : Fourth ventricle choroid plexus papilloma (CPP) presenting as apathy and depression. Delhi Psychiatry J 2013; 16:1–3Google Scholar
16 : Choroid plexus calcification: clinical, neuroimaging and histopathological correlations in schizophrenia. Rom J Morphol Embryol 2013; 54:365–369Medline, Google Scholar
17 : Choroid plexus calcification: a biological marker of suicidality. Int J Neurosci 1991; 57:95–97Crossref, Medline, Google Scholar
18 : Choroid plexus calcification as a possible marker of hallucinations in schizophrenia. Int J Neurosci 1993; 71:87–92Crossref, Medline, Google Scholar
19 : Choroid plexus calcification as a possible clue of serotonin implication in schizophrenia. Neurosci Lett 1999; 259:169–172Crossref, Medline, Google Scholar
20 : Transcriptome sequencing of the choroid plexus in schizophrenia. Transl Psychiatry 2016; 6:e964–e4Crossref, Medline, Google Scholar
21 : Clinical phenotypes of psychosis in the Bipolar-Schizophrenia Network on Intermediate Phenotypes (B-SNIP). Am J Psychiatry 2013; 170:1263–1274Link, Google Scholar
22 : Neuropsychological impairments in schizophrenia and psychotic bipolar disorder: findings from the Bipolar-Schizophrenia Network on Intermediate Phenotypes (B-SNIP) study. Am J Psychiatry 2013; 170:1275–1284Link, Google Scholar
23 : Neurotrophins and schizophrenia. Schizophr Res 2007; 94:1–11Crossref, Medline, Google Scholar
24 : Angiogenesis in the pathophysiology of schizophrenia: a comprehensive review and a conceptual hypothesis. Life Sci 2015; 128:79–93Crossref, Medline, Google Scholar
25 : A meta-analysis of blood cytokine network alterations in psychiatric patients: comparisons between schizophrenia, bipolar disorder and depression. Mol Psychiatry 2016; 21:1696–1709Crossref, Medline, Google Scholar
26 : Meningeal and choroid plexus cells--novel drug targets for CNS disorders. Brain Res 2013; 1501:32–55Crossref, Medline, Google Scholar
27 : Angiogenic and immune signatures in plasma of young relatives at familial high-risk for psychosis and first-episode patients: a preliminary study. Schizophr Res 2016; 170:115–122Crossref, Medline, Google Scholar
28 : C-reactive protein concentrations across the mood spectrum in bipolar disorder: a systematic review and meta-analysis. Lancet Psychiatry 2016; 3:1147–1156Crossref, Medline, Google Scholar
29 : C-reactive protein is increased in schizophrenia but is not altered by antipsychotics: meta-analysis and implications. Mol Psychiatry 2016; 21:554–564Crossref, Medline, Google Scholar
30 : What does the PANSS mean? Schizophr Res 2005; 79:231–238Crossref, Medline, Google Scholar
31 : Choroid plexus blood flow: evidence for dopaminergic influence. Brain Res 1984; 290:165–169Crossref, Medline, Google Scholar
32 : Effect of serotonin on blood flow to the choroid plexus. Brain Res 1989; 478:121–126Crossref, Medline, Google Scholar
33 : Type I/II interferon balance in the regulation of brain physiology and pathology. Trends Immunol 2016; 37:181–192Crossref, Medline, Google Scholar
34 : Therapeutic implications of the choroid plexus-cerebrospinal fluid interface in neuropsychiatric disorders. Brain Behav Immun 2015; 50:1–13Crossref, Medline, Google Scholar
35 : Lipopolysaccharide up-regulates IL-6R alpha expression in cultured leptomeningeal cells via activation of ERK1/2 pathway. Neurochem Res 2008; 33:1901–1910Crossref, Medline, Google Scholar
36 : IL-6 in inflammation, immunity, and disease. Cold Spring Harb Perspect Biol 2014; 6:a016295–a5Crossref, Medline, Google Scholar
37 : Cortical grey matter volume reduction in people with schizophrenia is associated with neuro-inflammation. Transl Psychiatry 2016; 6:e982–e2Crossref, Medline, Google Scholar
38 : Enlargement of choroid plexus in complex regional pain syndrome. Sci Rep 2015; 5:14329Crossref, Medline, Google Scholar
39 : Neuroinflammation and white matter pathology in schizophrenia: systematic review. Schizophr Res 2015; 161:102–112Crossref, Medline, Google Scholar