Postoperative Delirium
Mr. A, a 50-year-old English-speaking Korean man, presented to the hospital with right scapular pain. CT of the chest showed two masses, and a CT-guided fine-needle aspiration biopsy study revealed poorly differentiated non-small-cell carcinoma of the lung. MRI of the brain, with and without gadolinium, showed neither a mass lesion nor abnormal enhancement suggestive of metastatic disease.
Mr. A’s medical history was significant for hepatitis C virus infection. He had a 40-pack-year history of cigarette smoking and a remote history of cocaine use. He acknowledged drinking three or four alcoholic beverages weekly but answered “no” to the four items of the CAGE questionnaire.
Mr. A underwent two cycles of etoposide and cisplatin 50/50 chemotherapy, irradiation of the upper lobe of the right lung, and excision of this lobe and the overlying chest wall. Postoperatively, he was aware of his hospitalization and experienced pain at the excision site, which was controlled with fentanyl and bupivacaine epidural infusion. On the second postoperative day, Mr. A became less communicative and more agitated. Epidural infusions were stopped. No focal neurological deficits were found. That evening, he began to cry out unintelligibly and attempted to get out of bed. Soft restraints were applied. Because he was thought to be in alcohol withdrawal, lorazepam was administered intravenously in 1 mg doses every 2 hours (total 4 mg), with no reduction in agitation. Haloperidol (5 mg i.v.) was then administered with slight effect, and psychiatric consultation was requested.
Mr. A’s temperature was 100°F, pulse 135 bpm, and blood pressure 160/90 mm Hg. He was awake and moved all limbs vigorously, trying to wrest himself from the restraints. Although he attempted to respond when addressed in his native Korean, he produced no intelligible speech. He did not appear to be responding to internal stimuli. Rigidity, tremor, myoclonus, and asterixis were absent.
ECG revealed sinus tachycardia with a corrected QT interval (QTc) of 413 msec. The patient’s WBC count was 5300/μl, hemoglobin concentration 6.6 g/dl, hematocrit 18.7%, and mean corpuscular volume (MCV) 94 fl. His transaminase and bilirubin levels were normal. His sodium concentration was 134 mmol/liter, potassium 3.8 mmol/liter, chloride 101 mmol/liter, bicarbonate 27.4 mmol/liter, BUN 27 mg/dl, creatinine 0.6 mg/dl, glucose 126 mg/dl, magnesium 1.4 meq/liter, and albumin 4.0 g/dl. Arterial blood analysis revealed a pH of 7.42, a P o2 of 185 mm Hg, and a P co2 of 44 mm Hg on oxygen delivered at 4 liters/minute by nasal prong. The patient’s blood alcohol level on admission was 0.
Potassium and magnesium were administered intravenously. Haloperidol was administered intravenously in two 5-mg boluses, separated by 30 minutes, and the patient fell asleep. When roused, he was calm but disoriented and unable to answer questions accurately. His heart rate fell to 90 bpm and his systolic blood pressure decreased to 130 mm Hg. The patient was judged not to be in alcohol withdrawal, and lorazepam was tapered gradually over the next 48 hours. Chest X-ray and urinalysis were normal, and blood cultures were sterile.
Over the next 24 hours, the patient received a total of 30 mg of haloperidol intravenously in 5-mg boluses, and his mental status markedly improved. He answered questions, followed instructions, and no longer attempted to climb out of bed or remove catheters and monitoring devices. In the subsequent days, he engaged in meaningful conversations. Sensorium, attention, memory, and thought process normalized by postoperative day 5. At discharge, his hematocrit was at 25%. He returned home on postoperative day 8 and has continued with cancer treatment.
The word “delirium” derives from the Latin delirare , which means “to be out of one’s furrow.” Delirium is an acute cerebral state characterized by disturbed consciousness and cognitive dysfunction occurring in the setting of a physiological derangement caused by a medical disorder. Delirium is common, affecting between 15% and 60% of medical and surgical inpatients and more than 2 million elderly patients each year in the United States (1) . It is correlated with increased morbidity and mortality, prolonged hospital stays, and cognitive deterioration (2 – 4) . The prevalence of delirium in postoperative patients ranges from 10% to 51% (5) . Cardiac surgery in general poses an even greater risk (6) . The incidence of delirium in elderly postoperative patients is 15% to 53% and as high as 80% in elderly patients who require intensive care (7) . One-quarter of delirious elderly patients die within 6 months (8) .
The pathophysiology of delirium is associated with a wide variety of etiologies. Determining the etiology, if possible, is always the first step in managing the condition, and treating the underlying cause may be curative. The postoperative state may itself lead to delirium as the ebb phase of anesthetic-induced vasodilation precipitates a hypermetabolic inflammatory state that is proportional to the degree of surgical injury. The timeline of this phasic response is relatively immutable and coincides with the clinical presentation and course of postoperative delirium. The treatment of choice for the agitated behavior of postoperative delirium is neuroleptic medication, in most cases intravenous haloperidol, especially when agitation is severe. In this article, we offer a review of the diagnosis, risk factors, etiology, pathophysiology, management, and treatment of delirium.
Differential Diagnosis
Delirium is a state of acute brain failure with disturbance of consciousness accompanied by cognitive deficits that cannot be accounted for by past or evolving dementia and is associated with evidence of physiological disturbance owing to a medical condition (9) . The DSM-IV-TR diagnostic criteria include:
A. Disturbance of consciousness (i.e., reduced clarity of awareness of the environment) with reduced ability to focus, sustain, or shift attention.
B. A change in cognition (such as memory deficit, disorientation, language disturbance) or the development of a perceptual disturbance that is not better accounted for by a preexisting, established, or evolving dementia.
C. The disturbance develops over a short period of time (usually hours to days) and tends to fluctuate during the course of the day.
Other common features of delirium include sleep disturbances (including reversal of the sleep-wake cycle), psychomotor activity changes, and neurobehavioral symptoms.
Hyperactive delirium is characterized by a state of hyperarousal, hypervigilance, and pronounced agitation. Patients with hypoactive delirium are hypoalert and lethargic; often their symptoms are not appreciated because of their nondisruptive nature. Occasionally, patients will have a delirium with features of both motoric types.
Labile or altered affective changes, such as anger and irritability as well as apathy and abulia, are frequently described. Patients may experience symptoms of psychosis, interweaving delusional and hallucinated events with real ones. Formal thought disorder may also be observed. When patients do not show autonomic derangements or perceptual changes, the moniker “acute confusional state” is sometimes preferred.
The differential diagnosis of delirium requires specific attention to symptom profile; temporal onset; physical, neurological, and mental status examinations; and results of radiological and laboratory testing (10) . Asterixis and multifocal myoclonus are physical signs. In most non-alcohol-withdrawal deliria, EEG shows generalized slowing and increased amplitude (11) . The presence of triphasic waves may be helpful in distinguishing certain specific metabolic deliria (e.g., hepatic encephalopathy). Abnormal clock drawing and dysgraphia are useful bedside findings.
Acute alcohol withdrawal is often seen in the inpatient setting, especially after surgery. Symptoms, generally due to adrenergic excess, include tremulousness, anxiety, diaphoresis, palpitations, and insomnia. When more pronounced, there may be hallucinosis, withdrawal seizures, and alcohol withdrawal syndrome or delirium tremens, which nowadays carries a mortality rate of 1% (12) . Classically, alcohol withdrawal syndrome presents with fever, tachycardia, hypertension, tremor, insomnia, hallucinations (predominantly visual, but also auditory and tactile), agitation, anxiety, and seizures, along with reduced attention, disorientation, cognitive dysfunction, and language disturbances. Distinguishing alcohol withdrawal syndrome from postoperative delirium is a common challenge because of symptom overlap.
Dementia can be difficult to distinguish from delirium. Late-stage dementia can lead to confusion, agitation, and psychosis, and any type of cognitive impairment may predispose to delirium. Delirium superimposed on dementia, or “beclouded dementia,” has a prevalence ranging from 23% to 89% in elderly patients with dementia (13) .
An accurate longitudinal history can help distinguish delirium from dementia. Abrupt onset of symptoms, with a waxing and waning course, is highly characteristic of delirium. Attention is significantly disturbed or fluctuates rapidly, whereas it remains relatively intact in uncomplicated dementia (14) . Hallucinations, delusions, and agitation levels are often prominent in delirium but usually are absent in dementia until the late stages.
Hypoactive delirium is often misdiagnosed as depression, as some overlap of symptoms occurs. These include psychomotor slowing, cognitive changes, sleep disturbances, irritability, and in cases of depression with psychotic features, perceptual changes and delusions. Attention and orientation are usually normal in depressive disorders, however.
Agitation in hyperactive delirium is often related to fear either caused or accompanied by symptoms of psychosis. This is often misdiagnosed as anxiety disorder and mistreated with benzodiazepines. Perceptual disturbances, along with thought abnormalities, can occur in both delirium and primary psychotic disorders. However, symptoms in delirium are more fluctuant and fragmentary and rarely as fixed or complex. Hallucinations in delirium tend to be visual rather than auditory. In addition, consciousness, attention, and cognition are generally less impaired in primary psychoses.
While the clinical evaluation remains paramount—and often it is the only approach available when encountering serious agitation—the history and examination can occasionally be supplemented by use of formal instruments. The Confusion Rating Scale (15) , the Mini-Mental State Examination (16) , the clock drawing test (17) , and the Glasgow Coma Scale (18) can be useful screens. The Delirium Symptom Interview, the Confusion Assessment Method (19) , and the Delirium Scale (20) can all aid in formal diagnosis. Instruments used to rate delirium severity include the Delirium Rating Scale (21) and the Memorial Delirium Assessment Scale (22) .
Our patient exhibited classic symptoms and signs of a hyperactive postoperative delirium with clear onset on postoperative day 2. An alcohol withdrawal syndrome was initially suspected, but a blood alcohol level of 0, a negative CAGE screen, normal MCV, and normal levels of liver enzymes, bilirubin, and albumin argued against it. The patient’s non-small-cell carcinoma of the lung suggested the remote possibility of paraneoplastic limbic encephalitis, but his MRI was unremarkable and his postoperative delirium responded briskly to haloperidol.
Risk Factors
Preoperative risk factors for postoperative delirium include advanced age, dementia, tobacco use, poor preoperative functional status, depression, abnormal serum levels of sodium, potassium, and glucose, and an albumin level ≤4.0 g/dl (23 – 26) . Intraoperative risk factors include blood loss and fluid infusion needs (27 , 28) . Vascular surgery (e.g., abdominal aortic aneurysm repair, coronary artery bypass grafting) is approximately twice as likely to cause delirium as elective orthopedic, abdominal, or head and neck operations (24) . A postoperative hematocrit of less than 30% and the need for multiple blood transfusions are risk factors, as are abnormal sodium, potassium, and glucose levels and an albumin concentration below 3.0 g/dl (27 , 29 , 30) .
Our patient’s anemia, respiratory compromise in the setting of thoracic surgery, history of heavy tobacco use, and metabolic derangements were risk factors predisposing him to a postoperative delirium.
Etiology and Pathophysiology
The pathophysiology of delirium is associated with a wide variety of etiologies ( Figure 1 ). Seven states (designated by the mnemonic WHHHIMP [31] ) require prompt intervention to avoid death or permanent CNS damage: Wernicke’s encephalopathy, hypoxia, hypoglycemia, hypertensive encephalopathy, intracerebral hemorrhage, meningitis/encephalitis, and poisoning, whether exogenous or iatrogenic. Other conditions requiring prompt intervention include subdural hematoma, septicemia, subacute bacterial endocarditis, hepatic or renal failure, thyrotoxicosis/myxedema, alcohol withdrawal syndrome, anticholinergic psychosis, and complex partial status epilepticus. Postoperative delirium, which may be complicated by these conditions, bears a temporal and etiological relationship to surgery, usually developing between postoperative days 2 and 7.
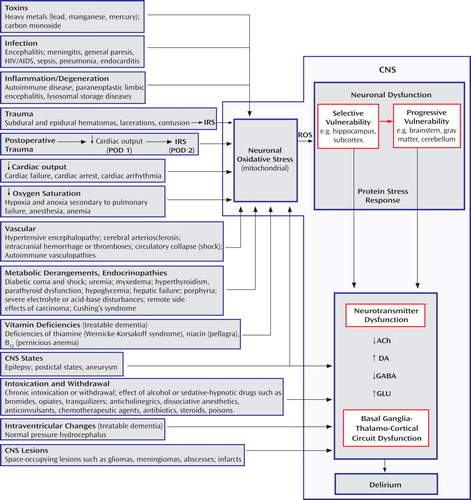
a Arrows point to major CNS sites of etiology impact. CNS=central nervous system; IRS=inflammatory response system; POD=postoperative day; ROS=reactive oxygen species; ACh=acetylcholine; DA=dopamine; GABA=γ-aminobutyric acid; GLU=glutamate.
Delirium is a potentially reversible dysregulation of neuronal functioning caused by oxidative stress initiated by a disturbance in brain structures most vulnerable to insult (32) . This “selective vulnerability” results in dysfunction of specific neurotransmitter systems. If the insult persists, less vulnerable brain structures also become dysfunctional in a process called “progressive vulnerability.” Hippocampal neurons are affected early in delirium, followed by neurons of the subcortex, brainstem, gray matter, and cerebellum (32) .
The inflammatory response syndrome resulting from anesthetized surgical or unanesthetized traumatic injury is a predictable phasic adaptation that optimizes the body’s healing capacity (33) . The “ebb” phase is akin to shock, affecting cardiac output, heart rate, oxygen consumption, gluconeogenesis, lipolysis, and cytokine and catecholamine levels. This roughly 24-hour period represents an attempt to survive in the face of acute injury by decreasing energy requirements. This first phase occurs during emergencies of hemorrhage, sepsis, and dehydration, as well as after elective surgery, when common anesthetic agents profoundly reduce cardiac output, thus activating the conservatory mechanisms described above.
When surgical interventions expand circulatory volume, other survival signals are activated, initiating the second phase of “flow” or hypermetabolism. The degree of initial injury proportionately propels the hypermetabolic response, yet the timeline of the response is relatively immutable. On postoperative day 2, almost all of the inflammatory and catabolic mediators peak, and they return to baseline levels by days 6 to 7. This course is closely correlated with the clinical course of an uncomplicated postoperative delirium, which suggests that the inflammatory response syndrome may be pathophysiologically involved.
For example, the inflammatory response syndrome may then affect circumventricular fenestrated endothelial zones, creating areas of inflammation leading to limbic disturbance and symptoms of delirium (34) . The transcription factor NF-kB is excited by the inflammatory response syndrome as well as by both oxidative and psychosocial stress, resulting in proinflammatory cytokine stimulation of delirium-potentiating neurotoxins (35) . An inflammatory response syndrome-stimulated macrophage enzyme called indoleamine 2,3-dioxygenase favors kynurenine production (36) . Excess kynurenine crossing the blood-brain barrier will potentiate a delirium through oxidative stress. In addition, the inflammatory response syndrome stimulates excessive cortisol release, which can exacerbate delirium.
Cholinergic, dopaminergic, histaminergic, noradrenergic, and serotonergic neurons are most susceptible to oxidative stress. Oxidative stress leads to neuronal calcium influx, mitochondrial strain, and a protein stress response. Enhanced release of some, but not all, neurotransmitters occurs, because while some enzymatic reactions require oxygen as a cofactor, others do not. For example, glutamate increases during hypoxia, which causes excitotoxicity, whereas oxidative stress decreases the synthesis of γ-aminobutyric acid (GABA), and even mild hypoxia interferes with a cholinergic cell’s ability to synthesize and release acetylcholine (32 , 37) .
In delirium, there is dysfunction of several brain nodal points, including subcortical structures such as the amygdala and hippocampus, the brainstem, the thalamus, the nondominant parietal lobe, and the prefrontal cortices, all connected by basal ganglia-thalamo-cortical loops. Damage at these nodal points will enhance vulnerability to delirium. An important branch of these circuits is the medial forebrain bundle, which carries axons from the ventral tegmentum (dopamine), the locus ceruleus (norepinephrine), and the nucleus raphe (serotonin). Corticostriatal glutamatergic, distributed cholinergic, and GABA-ergic neuronal tracts are also key variables in basal ganglia-thalamo-cortical functioning.
In the striatum, dopamine is required for glutamate to precipitate hypoxic neuroinjury. Dopamine blockade may protect neurons against subsequent hypoxic stress and injury (22) . Preclinical studies suggest that haloperidol may protect against brain ischemic oxidative stress through its sigma-1 antagonistic butyrophenone effect (38) .
Clinically, intravenous haloperidol can rapidly improve hippocampal function (e.g., short-term memory) and reverse frontal disinhibition. Dopamine excess may cause agitation and delusions. Acetylcholine insufficiency may lead to disorientation, hallucinations, and memory impairment (39) . In animals, stress increases mesocorticolimbic dopamine release (40) . Dopamine, a weak inhibitor of acetylcholine release, in excess may exacerbate the effects of a relative acetylcholine deficiency (41) .
Psychotic fear in delirium may originate in the central extended amygdala, which normally integrates internal stimuli from the hypothalamus and brainstem and external stimuli from the basolateral amygdala (42) . In delirium, abnormal signals from the hippocampus, temporal cortex, and medial orbitofrontal cortex may converge on the basolateral amygdala, which subsequently sends disordered inputs to the central extended amygdala. The medial central extended amygdala may then abnormally excite dopamine subpopulations that in turn project to limbic areas and to cognitive regions of the cortex and striatum. This may be why the fear in delirium requires dopamine-blocking neuroleptics rather than benzodiazepines to be managed effectively.
Management and Treatment
Because postoperative delirium is a diagnosis of exclusion, evaluation of other etiologies is the first step in management and treatment ( Figure 2 ). Eliminating underlying causes can be corrective, and pharmacological agents are among the most common iatrogenic causes of delirium.
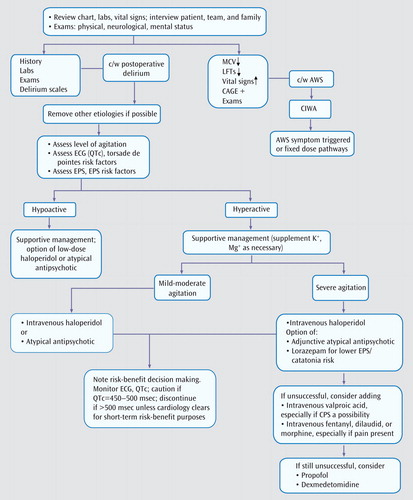
a C/W=consistent with; QTc=corrected QT interval; EPS=extrapyramidal symptoms; MCV=mean corpuscular volume; LFTs=liver function tests; CIWA=Clinical Institute Withdrawal Assessment for Alcohol; AWS=alcohol withdrawal syndrome; CPS=complex partial seizures.
Review of current and past medications is essential, since delirium may occur when CNS-active drugs reach toxic levels or when certain drugs and substances associated with physiological dependence are withdrawn abruptly. Drug-drug interactions can further complicate the issue. Management entails discontinuing or reducing the dose of the offending drug(s) when feasible.
If removal of deliriogenic factors fails to lead to improvement and if the patient’s behavior dictates it, the treatment of choice is neuroleptic medication. Haloperidol administered intravenously is the mainstay of the management of delirious agitation, as underscored in APA guidelines (43 , 44) , despite the fact that this route of administration has not been approved by the U.S. Food and Drug Administration (FDA) and that haloperidol now carries a black box warning about the potential for lethal ventricular arrhythmias, including torsade de pointes (45) . At least 28 case reports of QT prolongation and torsade de pointes, some with fatal outcome, have been reported with haloperidol. It may be of anecdotal interest in this regard that with over 90 career years of haloperidol use among the authors, none of us can recall seeing a case of torsade de pointes after initiating intravenous haloperidol, although we have run across other ventricular arrhythmias. When prescribing intravenous haloperidol, one should monitor the ECG and clarify why the risk-benefit ratio favors its use. Haloperidol may be especially effective against agitation because of its strong dopamine D 2 receptor binding affinity, which atypical antipsychotics lack. It has few anticholinergic side effects and few active metabolites. The effects of haloperidol on blood pressure, pulmonary artery pressure, heart rate, and respiration are milder than those of intravenous benzodiazepines and other neuroleptics, and it is less sedating (43 , 44 , 46) .
Intravenous administration of haloperidol is preferable to intramuscular administration. Drug absorption may be poor in distal muscles if there is hemodynamic instability. In addition, painful intramuscular injections may exacerbate paranoia. Interpretations of muscle enzyme values may be erroneous with frequent intramuscular injections. Moreover, haloperidol administered intravenously is less likely to produce extrapyramidal symptoms than intramuscular or even oral haloperidol (47) . Intravenous haloperidol has a mean distribution time of 11 minutes, although in critically ill and elderly patients this interval may be prolonged, and thus tranquilizing effects may take 15–20 minutes. The mean half-life of haloperidol when given intravenously is about 24 hours. Intravenous haloperidol is twice as potent as the oral form (46) .
We often prescribe 2–2.5 mg i.v. of haloperidol for mild agitation, 5 mg i.v. for moderate agitation, and 7.5–10 mg i.v. for severe agitation. In the elderly, doses should be approximately one-third of what is usually prescribed. The APA guidelines recommend 0.25–0.5 mg every 4 hours as needed for elderly delirious patients (43 , 44) .
Doses can be repeated intravenously every 30 minutes until the patient is calm yet arousable to normal voice. When serious agitation persists, the previous dose can be doubled 30 minutes later; this approach of successive doubling can be repeated. The goal is to ameliorate the agitation rapidly, as partial control can sometimes prolong the delirious state. For reemergent agitation, the last effective dose can be administered. For unremitting agitation, a continuous infusion of 5–10 mg/hour can be instituted, although we have very rarely needed this approach.
Careful, painstaking clinical evaluation should guide any necessary medication adjustments. If sensorium and behavior improve, lower doses of intravenous haloperidol or an orally administered atypical antipsychotic may suffice.
Maximum daily doses have not been established, although over 1000 mg of intravenous haloperidol has been used safely (48) . Hypotension can occur as a result of a low-volume state; if this occurs, monitored volume replacement can precede administration of any further doses. Haloperidol may lower seizure threshold, although it is one of the safest neuroleptics in this respect. Haloperidol is rarely associated with respiratory depression and is well tolerated by patients with chronic obstructive pulmonary disease. In the ICU setting it can often help reduce fear and agitation associated with ventilator weaning.
Haloperidol infrequently causes ventricular arrhythmia, occasionally in a torsade de pointes configuration, so ECG monitoring is important, especially in susceptible populations (49 , 50) . Drug-induced QTc prolongation is relatively common, whereas QTc greater than 500 msec is uncommon and torsade de pointes is rare. Risk factors include the myocytic drug effect of blocking the delayed rectifier potassium channel (I Kr ) resulting in decreased K + outflux, which is how neuroleptic medications primarily offend. Secondary risk factors amplify a congenital or acquired reduced “repolarization reserve.” Individuals with reduced repolarization reserve cannot compensate for drugs that block I Kr and threaten depolarization. Secondary risk factors include high doses or rapid administration, inhibition of cytochrome P450 liver enzymes (for example, paroxetine and ketoconazole are inhibitors of haloperidol oxidative metabolism), impaired drug elimination, bradycardia, hypokalemia, hypomagnesemia, left ventricular hypertrophy, heart failure, recent conversion of atrial fibrillation, hypothyroidism, older age, female gender, concomitant use of another I Kr blocker, undetected ion channel polymorphisms, and congenital long QT syndrome. The vast majority of patients who develop drug-related torsade de pointes have at least one risk factor.
Pharmacodynamic interactions with other QTc-prolonging medications, such as fluoroquinolones, macrolides, class I antiarrhythmics, tricyclic antidepressants, carbamazepine, and methadone, may further contribute to prolonged QTc. Discontinuation or a switch to non-QTc-prolonging medications may be required.
In healthy male subjects, orally administered haloperidol causes a mean increase in QTc of 4.7 msec. Atypical antipsychotics can also increase the QTc, as reported in a Pfizer study for the FDA (50 , 51) : olanzapine causes a mean increase of 6.8 msec, risperidone 11.6 msec, quetiapine 14.5 msec, and ziprasidone 20.3 msec. Aripiprazole may offer a better QTc risk profile and has been used in cases of mild to moderate delirium, as have other atypical antipsychotics (52 , 53) .
In a meta-analysis of two randomized controlled trials—haloperidol versus risperidone and haloperidol versus olanzapine—no evidence of differential effect on symptoms and side effects was noted when low-dose haloperidol was used (54 – 56) . With haloperidol doses greater than 4.5 mg/day, more extrapyramidal side effects occurred. In neither study was haloperidol given intravenously, nor were possible ethnopsychopharmacological confounders addressed. Nevertheless, the authors of the meta-analysis suggest using atypical antipsychotics instead of orally administered haloperidol in high doses or when there is a high risk of extrapyramidal side effects or cardiotoxicity.
Unfortunately, this is not always a pragmatic suggestion. While atypical antipsychotics given orally or intramuscularly are useful for mild to moderate delirious agitation and sometimes as a supplement for severe and dangerous agitation, the treatment of choice in the latter case, in our view, is intravenous haloperidol in high doses if needed because it offers the safest and quickest way to calm the patient, in whom the risk of insufficiently treated severe agitation often outweighs the risk of side effects. After reviewing the QTc risk factor profile, we make corrections as needed (e.g., supplementation of potassium to 4 mmol/liter and magnesium to 2 meq/liter) before giving intravenous haloperidol. When the QTc increases to 450–500 msec, there is cause for concern and caution; when it exceeds 500 msec, there is an increased risk of torsade de pointes (49) . We adhere to APA guidelines suggesting that a QTc interval greater than 450 msec or more than 25% over baseline may warrant a cardiology consultation and reduction or discontinuation of the neuroleptic medication. Occasionally, however, when the QTc is greater than 500 msec and a risk-benefit analysis made in concert with the cardiology and medical teams indicates that it is clinically necessary to continue acute, short-term treatment, we continue cautiously with the aid of cardiac telemetry, close monitoring of electrolytes, and elimination of any reversible pharmacokinetic risks and potentially adverse pharmacodynamic interactions.
Intravenous droperidol, which carries a black box warning, can also reduce delirious agitation; however, this agent is a more potent α 1 -adrenergic receptor antagonist and thus is more likely to cause hypotension. It can also be more of a respiratory depressant. Other parenteral neuroleptic drugs occasionally used for treatment of agitation include perphenazine and chlorpromazine, which are effective but unfortunately can cause hypotension and occasionally a decline in cardiac output because of their potent α 1 -adrenergic antagonism. These phenothiazines are relatively anticholinergic, can lower the seizure threshold, and prolong the QTc. However, in low doses they can be both safe and effective.
Adding lorazepam (1–2 mg i.v. every 2–4 hours) to haloperidol in the management of severely agitated patients can be helpful because lorazepam may reduce the extrapyramidal side effects of haloperidol, especially akathisia (57 , 58) . Lorazepam given intravenously has a half-life of about 12–14 hours; therefore, its sedating effect may accumulate, and tapering before ventilator weaning may be needed. Nevertheless, lorazepam is one of the least likely benzodiazepines to cause respiratory depression. Along with oxazepam and temazepam, it is safest for patients with hepatic insufficiency because it does not require oxidative metabolism.
The addition of intravenous valproate may be considered to manage severe agitation when conventional therapy is inadequate or when problematic side effects emerge (59) . Fentanyl or hydromorphone can be helpful for severely agitated patients on mechanical ventilation. If these medications are not sufficiently calming, sedation with ventilator support can be achieved with propofol or dexmedetomidine. Propofol is deeply sedating and is short acting. It has a titratable progression from anxiolysis to sedation to hypnosis to anesthesia (60) . However, when used over a prolonged period (≥2 weeks), tachyphylaxis can emerge, making weaning from propofol difficult (61) . In these situations, we have successfully used dexmedetomidine, a very selective α 2 -adrenergic receptor agonist, with caution regarding low-output cardiac side effects. Dexmedetomidine warrants further study in postoperative delirium (62) .
Pain can exacerbate agitation in postoperative delirium. Morphine, hydromorphone, or fentanyl can be used. Respiratory depression and hypotension are potential side effects. In patients being managed with fentanyl, it is occasionally difficult to achieve steady-state tranquilization because of this agent’s short-acting nature. Meperidine is to be avoided because of deliriogenesis related to its normeperidine metabolite. Patient-controlled analgesia and patient-controlled epidural analgesia using an opioid and a local anesthetic are important strategies for controlling pain after surgery. In one randomized study of elderly patients who had undergone major abdominal surgery, both of these methods were effective, but patient-controlled epidural analgesia was actually associated with better pain, mental status, and bowel outcomes over the 5-day postoperative study period (63) .
Specific nonpharmacological management strategies for delirium have been advocated (5 , 10) (see Table 1 ). At present, evidence on delirium prevention is sparse, but more research is on the way (64) .
Conclusion
Postoperative delirium is an important condition with significant associated morbidity and mortality, especially in the elderly. Neuroleptics are helpful in the treatment of delirium, and haloperidol given intravenously remains the treatment of choice for delirium with severe agitation, which is the most challenging type of delirium that psychiatric consultants confront in the general hospital.
1. Ely EW, Siegel MD, Inouye SK: Delirium in the intensive care unit: an underrecognized syndrome of organ dysfunction. Semin Resp Crit Care Med 2001; 22:115–126Google Scholar
2. McCusker J, Cole M, Abrahamowicz M, Primeau F, Belzile E: Delirium predicts 12-month mortality. Arch Intern Med 2002; 162:457–463Google Scholar
3. McCusker J, Cole MG, Dendukuri N, Belzile E: Does delirium increase hospital stay? J Am Geriatr Soc 2004; 51:1539–1546Google Scholar
4. McCusker J, Cole M, Dendukuri N, Belzile E, Primeau F: Delirium in older medical inpatients and subsequent cognitive and functional status: a prospective study. CMAJ 2001; 165:575–583Google Scholar
5. Wise MG, Hilty DM, Cerda GM, Trzepacz PT: Delirium (confusional states), in Textbook of Consultation-Liaison Psychiatry: Psychiatry in the Medically Ill. Edited by Wise MG, Rundell JR. Washington, DC, American Psychiatric Publishing, Inc, 2002, pp 257–272Google Scholar
6. van der Mast RC, Roest FHJ: Delirium after cardiac surgery: a critical review. J Psychosom Res 1996; 41:13–30Google Scholar
7. Demeure MJ, Fain MJ: The elderly surgical patient and postoperative delirium. J Am Coll Surg 2006; 203:752–757Google Scholar
8. Fann JR: The epidemiology of delirium: a review of studies and methodological issues. Semin Clin Neuropsychiatry 2000; 5:64–74Google Scholar
9. American Psychiatric Association: Diagnostic and Statistical Manual of Mental Disorders, 4th ed, Text Revision (DSM-IV-TR). Washington, DC, American Psychiatric Association, 2000Google Scholar
10. Marcantonio E: The management of delirium, in Delirium in Old Age. Edited by Lindsay J, Rockwood R, Macdonald A. Oxford, UK, Oxford University Press, 2002, pp 123–151Google Scholar
11. Engel GL, Romano J: Delirium: a syndrome of cerebral insufficiency. J Chronic Dis 1959; 9:260–277Google Scholar
12. Mayo-Smith MF, Beecher LH, Fischer TL, Gorelick DA, Guillaume JL, Hill A, Jara G, Kasser C, Melbourne J; Working Group on the Management of Alcohol Withdrawal Delirium, Practice Guidelines Committee, American Society of Addiction Medicine: Management of alcohol withdrawal delirium: an evidence-based practice guideline. Arch Intern Med 2004; 164:1405–1412Google Scholar
13. Fick DM, Agostini JV, Inouye SK: Delirium superimposed on dementia: a systematic review. J Am Geriatr Soc 2002; 50:1723–1732Google Scholar
14. Trzepacz PT, Mulsant BH, Dew MA, Pasternak R, Sweet RA, Zubenko GS: Is delirium different when it occurs in dementia? a study using the delirium rating scale. J Neuropsychiatry Clin Neurosci 1998; 10:199–204Google Scholar
15. Williams MA, Ward SE, Campbell EB: Confusion: testing versus observation. J Gerontol Nurs 1988; 14:25–30Google Scholar
16. Folstein MF, Folstein SE, McHugh PR: “Mini-Mental State”: a practical method for grading the cognitive state of patients for the clinician. J Psychiatr Res 1975; 12:189–198Google Scholar
17. Shulman KI, Shedletsky R, Silver I: The challenge of time: clock drawing and cognitive function in the elderly. Int J Geriatr Psychiatry 1986; 1:135–140Google Scholar
18. Teasdale G, Jennett B: Assessment of coma and impaired consciousness: a practical scale. Lancet 1974; 2:81–84Google Scholar
19. Inouye SK, van Dyck CH, Alessi CA, Balkin S, Siegal AP, Horwitz RI: Clarifying confusion: the confusion assessment method: a new method for the detection of delirium. Ann Intern Med 1990; 113:941–948Google Scholar
20. Lowy F, Engelsmann F, Lipowski Z: Study of cognitive functioning in a medical population. Compr Psychiatry 1973; 14:331–338Google Scholar
21. Trzepacz P, Baker R, Greenhouse J: A symptom rating scale for delirium. Psychiatry Res 1998; 23:89–97Google Scholar
22. Breitbart W, Rosenfeld B, Roth F, Smith MJ, Cohen K, Passik S: The Memorial Delirium Assessment Scale. J Pain Symptom Manage 1997; 13:128–137Google Scholar
23. Marcantonio ER, Goldman L, Mangione CM, et al: A clinical prediction rule for delirium after elective noncardiac surgery. JAMA 1994; 271:134–139Google Scholar
24. Rudolph JL, Jones RN, Rasmussen LS, Silverstein JH, Inouye SK, Marcantonio ER: Independent vascular and cognitive risk factors for postoperative delirium. Am J Med 2007; 120:807–813Google Scholar
25. van der Mast RC, van den Broek WW, Fekkes D, Pepplinkhuizen L, Habbema JD: Incidence of and preoperative predictors for delirium after cardiac surgery. J Psychosom Res 1999; 46:479–483Google Scholar
26. Galanakis P, Bickel H, Gradinger R, Von Gumppenberg S, Förstl H: Acute confusional state in the elderly following hip surgery: incidence, risk factors, and complications. Int J Geriatr Psychiatry 2001; 16:349–355Google Scholar
27. Marcantonio ER, Goldman L, Orav EJ, Cook EF, Lee TH: The association of intraoperative factors with the development of postoperative delirium. Am J Med 1998; 105:380–384Google Scholar
28. Böhner H, Hummel TC, Habel U, Miller C, Reinbott S, Yang Q, Gabriel A, Friedrichs R, Müller EE, Ohmann C, Sandmann W, Schneider F: Predicting delirium after vascular surgery: a model based on pre- and intraoperative data. Ann Surg 2003; 238:149–156Google Scholar
29. Yildizeli B, Ozyurtkan MO, Batirel HF, Kuşcu K, Bekiroglu N, Yüksel M: Factors associated with postoperative delirium after thoracic surgery. Ann Thorac Surg 2005; 79:1004–1009Google Scholar
30. Inouye SK, Charpentier PA: Precipitating factors for delirium in hospitalized elderly persons: predictive model and interrelationship with baseline vulnerability. JAMA 1996; 275:852–857Google Scholar
31. Cassem NH, Murray GB, Lafayette JM, Stern TA: Delirious patients, in The MGH Handbook of General Hospital Psychiatry, 5th ed. Edited by Stern TA, Fricchione GL, Cassem NH, Jellinek MS, Rosenbaum JF. St Louis, Mosby, 2004, pp 119–134Google Scholar
32. Brown TM: Basic mechanisms in the pathogenesis of delirium, in Psychiatric Care of the Medical Patient, 2nd ed. Edited by Stoudemire A, Fogel BS, Greenberg DB. New York, Oxford University Press, 2000, pp 571–580Google Scholar
33. Kohl BA, Deutschman CS: The inflammatory response to surgery and trauma. Curr Opin Crit Care 2006; 12:325–332Google Scholar
34. Saper CB: Neurobiological basis of fever. Ann NY Acad Sci 1998; 856:90–94Google Scholar
35. Bierhaus A, Humpert PM, Nawroth PP: NF-kB as a molecular link between psychosocial stress and organ dysfunction. Pediatr Nephrol 2004; 19:1189–1191Google Scholar
36. Wichers MC, Maes M: The role of indoleamine 2,3-dioxygenase (IDO) in the pathophysiology of interferon-a-induced depression. J Psychiatry Neurosci 2004; 29:11–17Google Scholar
37. Gibson GE: Hypoxia, in Cerebral Energy Metabolism and Metabolic Encephalopathy. Edited by McCandless DW. New York, Plenum, 1985, pp 43–78Google Scholar
38. Schetz JA, Perez E, Liu R, Chen S, Lee I, Simpkins JW: A prototypical sigma-1 receptor antagonist protects against brain ischemia. Brain Res 2007; 1181:1–9Google Scholar
39. Murray GB: Excess endogenous dopamine and decreased endogenous acetylcholine responsible for acute delirium: an hypothesis (abstract). J Neuropsychiatry Clin Neurosci 1993; 5:456Google Scholar
40. Thierry AM, Tassin JP, Blanc G, Glowinski J: Selective activation of mesocortical DA system by stress. Nature 1976; 263:242–244Google Scholar
41. Coffman JA, Dilsaver SC: Cholinergic mechanisms in delirium. Am J Psychiatry 1988; 145:382–383Google Scholar
42. Fudge JL, Emiliano AB: The extended amygdala and the dopamine system: another piece of the dopamine puzzle. J Neuropsychiatry Clin Neurosci 2003; 15:306–316Google Scholar
43. American Psychiatric Association: Practice guideline for the treatment of patients with delirium. Am J Psychiatry 1999; 156(May suppl)Google Scholar
44. Cook IA: Guideline Watch: Practice Guideline for the Treatment of Patients With Delirium. Arlington, Va, American Psychiatric Association, 2004Google Scholar
45. Shapiro BA, Warren J, Egol AB, Greenbaum DM, Jacobi J, Nasraway SA, Schein RM, Spevetz A, Stone JR: Practice parameters for intravenous analgesia and sedation for adult patients in the intensive care unit: an executive summary (Society of Critical Care Medicine). Crit Care Med 1995; 23:1596–1600Google Scholar
46. Sos J, Cassem NH: Managing postoperative agitation. Drug Ther 1980; 10:103–106Google Scholar
47. Menza MA, Murray GB, Holmes VF, Rafuls WA: Decreased extrapyramidal symptoms with intravenous haloperidol. J Clin Psychiatry 1987; 48:278–280Google Scholar
48. Tesar GE, Murray GB, Cassem NH: Use of high-dose intravenous haloperidol in the treatment of agitated cardiac patients. J Clin Psychopharmacol 1985; 5:344–347Google Scholar
49. Heist EK, Ruskin JN: Drug-induced proarrhythmia and use of QTc-prolonging agents: clues for clinicians. Heart Rhythm 2005; 2:S1–S8Google Scholar
50. Glassman AH, Bigger JT Jr: Antipsychotic drugs: prolonged QTc interval, torsade de pointes, and sudden death. Am J Psychiatry 2001; 158:1774–1782Google Scholar
51. US Food and Drug Administration Advisory Committee: Zeldox Capsules (ziprasidone): Summary of Efficacy and Safety and Overall Benefit Risk Relationship. Bethesda, Md, US Food and Drug Administration, July 19, 2000Google Scholar
52. Straker DA, Shapiro PA, Muskin PR: Aripiprazole in the treatment of delirium. Psychosomatics 2006; 47:385–391Google Scholar
53. Boettger S, Breitbart W: Atypical antipsychotics in the management of delirium: a review of the empirical literature. Palliat Support Care 2005; 3:227–237Google Scholar
54. Han CS, Kim YK: A double-blind trial of risperidone and haloperidol for the treatment of delirium. Psychosomatics 2004; 45:297–301Google Scholar
55. Hu H, Deng W, Yang H: A prospective random control study comparison of olanzapine and haloperidol in senile delirium. Chongging Med J 2004; 8:1234–1237Google Scholar
56. Lonergan E, Britton AM, Luxenberg J, Wyller T: Antipsychotics for delirium. Cochrane Database Syst Rev 2007; 2:CD005594Google Scholar
57. Menza MA, Murray GB, Holmes VF, Rafuls WA: Controlled study of extrapyramidal reactions in the management of delirious, medically ill patients: intravenous haloperidol versus intravenous haloperidol plus benzodiazepines. Heart Lung 1988; 17:238–241Google Scholar
58. Adams F, Fernandez F, Andersson BS: Emergency pharmacotherapy of delirium in the critically ill cancer patient. Psychosomatics 1986; 27(Jan suppl):33–38Google Scholar
59. Bourgeois JA, Koike AK, Simmons JE, Telles S, Eggleston C: Adjunctive valproic acid for delirium and/or agitation on a consultation-liaison service: a report of six cases. J Neuropsychiatry Clin Neurosci 2005; 17:232–238Google Scholar
60. Smith I, White PF, Nathanson M, Gouldson R: Propofol: an update on its clinical use. Anesthesiology 1994; 81:1005–1043Google Scholar
61. Currier DS, Bevacqua BK: Acute tachyphylaxis to propofol sedation during ethanol withdrawal. J Clin Anesth 1997; 9:420–423Google Scholar
62. Szumita PM, Baroletti SA, Anger KE, Wechsler ME: Sedation and analgesia in the intensive care unit: evaluating the role of dexmedetomidine. Am J Health Syst Pharm 2007; 64:37–44Google Scholar
63. Mann C, Pouzeratte Y, Boccara G, Peccoux C, Vergne C, Brunat G, Domergue J, Millat B, Colson P: Comparison of intravenous or epidural patient-controlled analgesia after major abdominal surgery. Anesthesiology 2000; 92:433–441Google Scholar
64. Siddiqi N, Stockdale R, Britton AM, Holmes J: Interventions for preventing delirium in hospitalised patients. Cochrane Database Syst Rev 2007; 2:CD005563Google Scholar