Elevated Neuron Number in the Limbic Thalamus in Major Depression
Abstract
OBJECTIVE: The mediodorsal and anteroventral/anteromedial nuclei of the thalamus are brain regions of interest in the study of mood disorders because they connect subcortical limbic system structures such as the amygdala with the prefrontal, cingulate, and temporal cortices. Anatomical abnormalities have been observed both in the amygdala and in the aforementioned cortical regions in affective disorder patients. Neuroanatomical studies of the thalamus have rarely been conducted in patients with mood disorders. METHOD: Postmortem tissue from the Stanley Foundation Brain Bank was obtained from subjects diagnosed with major depressive disorder, bipolar disorder, and schizophrenia as well as a nonpsychiatric comparison group (N=10–13 per group). The optical disector stereological procedure was used to count neurons in the mediodorsal and anteroventral/anteromedial nuclei of the thalamus in each brain. RESULTS: There were significantly more neurons in the mediodorsal (37%) and anteroventral/anteromedial (26%) nuclei in subjects with major depressive disorder relative to the nonpsychiatric comparison subjects. Neuron numbers and volumes in these limbic thalamic nuclei were normal in the schizophrenia and bipolar subjects. CONCLUSIONS: The data indicate that there is an elevation in total neuron number in the limbic thalamus that is specific for major depressive disorder. This represents the first report of a neuropsychiatric disorder being associated with an increase in total regional neuron number. The present findings, along with recent data, indicate that significant anatomical and functional abnormalities are present in limbic circuits in major depressive disorder.
The anterior and mediodorsal thalamus are important components of the limbic system, which plays a role in the expression and experience of emotion. Reciprocal connections between the mediodorsal thalamus and the dorsolateral prefrontal, orbitofrontal, cingulate, and insular cortices influence the limbic system by integrating and redistributing emotionally relevant stimuli to the frontal lobes (1–3). The anteroventral and anteromedial thalamic nuclei also play a role in this circuit by connecting frontohippocampal structures with the anterior cingulate and entorhinal cortex (4). Furthermore, the thalamus, particularly the mediodorsal thalamus, is a major target for output from the amygdala (5), an important temporal lobe limbic structure mediating emotional responses (6). Together, the mediodorsal and anteroventral/anteromedial nuclei play important roles in connecting subcortical limbic structures to the limbic cortex.
Anatomical changes have been observed in several sites in the limbic system of patients with mood disorders, including the medial orbitofrontal cortex, hippocampus, amygdala, and prefrontal cortex (7–10). White matter abnormalities have also been identified in major depressive disorder and bipolar disorder in regions containing thalamocortical axons (11, 12). Microscopic anatomical studies of the mediodorsal and anteroventral/anteromedial nuclei have not been reported in mood disorders (13).
In the present study, we estimated neuronal cell numbers and volumes of mediodorsal and anteroventral/anteromedial thalamic nuclei in subjects from the Stanley Foundation Brain Bank. Four groups were studied: subjects with major depressive disorder, bipolar disorder, and schizophrenia, and an age-matched group of nonpsychiatric comparison subjects.
Method
Data were obtained from either the left or right thalamus of both male and female subjects who had been diagnosed with major depressive disorder, bipolar disorder, schizophrenia, or no psychopathology. The collection and diagnosis of subjects in the Stanley Foundation Brain Bank has been described in detail previously (14). Sixty specimens (15/group) were originally obtained. However, some of the specimens were not counted because either they could not be appropriately stained (N=3), they had missing tissue (anteroventral/anteromedial: N=5; mediodorsal: N=8), or they exhibited evidence of gross thalamic pathology (congenital defect in the region of the medial thalamus, plaque-filled lesion in the globus pallidus, loss of staining in anteroventral neurons, or neuronal hyperpigmentation in surrounding tissue). Because of the differing number of specimens affected by missing tissue, there are different numbers of subjects in the anteroventral/anteromedial (N=49) and mediodorsal (N=46) analyses. Data in Table 1 outline the postmortem characteristics of the four subject groups.
Histology
For each brain, three coronal blocks containing the entire thalamus were embedded in paraffin by the Stanley Foundation. No sections were taken from the paraffin blocks. In order to save all of the tissue sections and use the tissue for stereological analysis, the thalamic blocks were subsequently deparaffinized. Prior to cutting, the blocks were immersed in cryoprotectant (20% sucrose/10% formalin solution for 2–3 weeks) and serially sectioned on a freezing microtome at 60 μm. After Nissl staining every 20th section with cresyl violet, the regional boundaries of the two thalamic nuclei were delineated at low magnification according to neuroanatomical boundaries defined by Jones (15). Darkfield examination of unstained sections aided in the identification of the neuroanatomical borders of the two nuclei. Figure 1 illustrates the borders of the anteroventral/anteromedial nuclei in unstained sections from two representative brains. The neuroanatomical boundaries of the anteroventral/anteromedial and mediodorsal nuclei have been described previously (16). The deparaffinization and re-embedding process resulted in a final section thickness in all specimens ranging from 9 to 14 μm. There was no difference in section thickness for any of the four groups according to analysis of variance (ANOVA) (F=0.56, df=3, 45, p<0.67).
Stereology
Cell counting was performed blind with respect to the identification of the brains. In each thalamic region, 6–11 sections were analyzed. Using more than 100 counting frames (50×75 μm systematically placed throughout the entire rostral-caudal extent of each nucleus, the average density of neurons in the counting frames was determined using a 100× oil immersion objective (numerical aperture=1.4). A 2-μm upper guard zone, a 2-μm lower guard zone, and a 7-μm thick counting frame was used for cell counting. One specimen had a thickness of 9 microns, and in this brain there was no lower guard zone (removing this specimen had no effect on mediodorsal or anteroventral/anteromedial neuron number statistics). Two hundred to 500 neurons containing a nucleolus clearly in focus within the counting frame were counted in each specimen. Figure 2 depicts a Nissl-stained section of the anteroventral thalamus, illustrating high and low power images. StereoInvestigator software (MicroBrightfield Inc., Williston, Vt.) was used to calculate thalamic nuclear volumes from planimetric data using the outlined borders of the nuclei and to estimate total neuron numbers using a grid-ratio fractionator approach. The coefficient of error was lower than 0.07 for each of the nuclei counted (mediodorsal: mean=0.061, range=0.04–0.08; anteroventral/anteromedial: mean=0.068, range=0.04–0.10). In addition, the overall volume of the thalamus for nonpsychiatric comparison subjects and subjects with major depressive disorder was estimated using the reticular nucleus as the lateral border but excluding the lateral geniculate nucleus.
Statistics
ANOVA followed by post hoc Dunnett’s tests for group comparisons with the nonpsychiatric comparison group was used to test for differences in cell number and volume. Chi-square analysis was performed for categorical data (e.g., right versus left hemisphere, male versus female). In addition, analysis of covariance (ANCOVA) with age, postmortem interval, gender, and laterality was performed with neuron number as the dependent variable.
Results
The four subject groups were matched for several clinical variables. According to ANOVA, the groups did not differ in terms of age, postmortem interval, time in formalin, or brain weight. Chi square analysis indicated that the groups did not differ in terms of gender or laterality (i.e., side of the brain analyzed) (Table 1).
There were more neurons in the mediodorsal and anteroventral/anteromedial nuclei in subjects with major depressive disorder compared with the nonpsychiatric comparison group. Mediodorsal neuron number was significantly different among the four groups of subjects (F=3.42, df=3, 43, p<0.03) (Table 2). Dunnett’s test indicated that only the major depressive disorder group was significantly different (37% higher) from the nonpsychiatric comparison group. With ANCOVA, the main effect of diagnosis remained significant after accounting for age, postmortem interval, gender, and laterality covariates (F=3.43, df=7, 38, p<0.03), further validating the ANOVA finding of elevated neuron number in the mediodorsal nucleus in major depressive disorder. For anteroventral/anteromedial neuron number data, there was not a significant difference among the four subject groups according to the ANOVA (F=1.60, df=3, 45, p<0.20). However, ANCOVA with covariates of age, postmortem interval, gender, and laterality revealed a significant effect of diagnosis on anteroventral/anteromedial neuron number (F=3.15, df=7, 41, p<0.04). The ANCOVA post hoc test (least squares means Students contrast t test) indicated that only the major depressive disorder group had significantly more anteroventral/anteromedial neurons (26%) than the nonpsychiatric comparison group. The ANCOVA improvement was due in part to correcting the data for laterality and gender effects (right hemisphere structures are larger than left, and females structures are smaller). Figure 3 illustrates the distribution of mediodorsal and anteroventral/anteromedial cell counts for the four subject groups.
The history of psychiatric drug usage during the subjects’ lifetime was obtained from medical chart review (14). In order to determine whether antidepressant drug usage influenced the number of neurons in the two thalamic nuclei, we examined mediodorsal thalamic cell number in patients with schizophrenia who were treated (six of 11) versus not treated, and in bipolar cases that were treated (nine of 12) versus not treated with antidepressant medication. Antidepressant treatment did not influence mediodorsal thalamic neuron number in schizophrenia subjects (mean=4.25 million cells [SD=0.55] and 4.32 million cells [SD=0.33], respectively) or bipolar subjects (mean=4.46 [SD=0.86] and 4.23 [SD=0.46]) and did not influence anteroventral/anteromedial thalamic neuron number in the schizophrenia subjects (mean=0.91 million [SD=0.05] and 0.84 million [SD=0.20]) or bipolar subjects (mean=0.88 million [SD=0.32] and 1.0 million [SD=0.32]). Cumulative lifetime antipsychotic drug treatment in fluphenazine equivalents computed from medical records was not significantly correlated with mediodorsal or anteroventral neuron number or volume according to Pearson’s correlation analysis for the schizophrenia (N=11/11), bipolar (N=9/12), or combined (N=20) groups. For the entire cohort, utilization of neuroleptics at any point during the subjects’ lifetime did not have a significant effect on either neuron number or nucleus volume for either thalamic region.
The density of neurons in mediodorsal nuclei did not differ among the four groups of subjects according to ANOVA (nonpsychiatric comparison group: mean=24,654 neurons/mm3 [SD=4880]; major depressive disorder group: mean=27,324 neurons/mm3 [SD=2555]; bipolar group: mean=25,200 neurons/mm3 [SD=6409]; schizophrenia group: mean=25,522 neurons/mm3 [SD=2142]). Similarly, there was not a significant difference in the density of anteroventral/anteromedial neurons among the four groups (nonpsychiatric comparison group: mean=35,408 neurons/mm3 [SD=6232], major depressive disorder group: mean=37,542 neurons/mm3 [SD=8597]; bipolar group: mean=33,619 neurons/mm3 [SD=5165]; schizophrenia group: mean=35,348 neurons/mm3 [SD=4800]).
There was a tendency for the volume of the mediodorsal nucleus to be different among the four groups (ANOVA F=2.67, df=3, 43, p<0.06). Post hoc testing (Dunnett’s) indicated a significant difference (22%) between the major depressive disorder and nonpsychiatric comparison groups (p<0.05). However, the ANCOVA analyses with covariates of age, gender, laterality, and postmortem interval did not reveal volume differences among the four groups (F=0.76, df=7, 38, p<0.54). The anteroventral/anteromedial nucleus was not statistically different among the four groups (ANOVA F=1.66, df=3, 45, p<0.19; ANCOVA F=1.95, df=7, 41, p<0.14). We also examined the volume of the total thalamus in major depressive disorder and nonpsychiatric comparison subjects to see if the entire thalamus was larger in major depressive disorder cases. The mean total thalamus volume was 16% greater in the major depressive disorder group versus the comparison subjects, although the result was not significant at the p<0.05 level (comparison group: mean=878 mm3 [SD=105]; major depressive disorder group: mean=1020 mm3 [SD=186]; t=1.78, df=22, p<0.09).
Discussion
In the present study, the mediodorsal and the anteroventral/anteromedial nuclei of the thalamus had significantly more neurons in major depressive disorder cases relative to nonpsychiatric comparison subjects. This is the first study of neuron number in the thalamus of major depressive disorder subjects, and our findings are unique because increases in total neuron number have not previously been observed in psychiatric conditions. Contrary to previous studies, the number of neurons in the mediodorsal and anteroventral/anteromedial nuclei were normal in schizophrenia subjects.
The present data are consistent with other reports of abnormalities in subcortical limbic structures in major depressive disorder (13). In a structural magnetic resonance imaging study of first-episode depression patients, Frodl et al. (17) found a bilateral enlargement of the amygdala, and two additional studies report that the amygdala is enlarged in subsets of patients with depressive symptoms (18, 19). The pituitary gland is also enlarged in major depressive disorder (20). In the hypothalamus, there are several reports of increased numbers of immunoreactive cells (e.g., arginine vasopressin, vasopressin, corticotropin-releasing hormone) in major depressive disorder (21–23). These immunohistochemical studies differ from the present study because they report changes in subsets of immunoreactive cells rather than the total number of neurons. Both the hypothalamus and amygdala are highly interconnected with the mediodorsal and anteroventral/anteromedial thalamus, prefrontal cortex, and cingulate cortex (5, 24, 25). On the other hand, the caudate nucleus, putamen, and other basal ganglia structures are not increased in volume in major depressive disorder subjects (26), suggesting that anatomical changes are selective for subcortical structures with limbic connections. Finally, metabolism is increased in subcortical limbic structures in major depressive disorder, including the amygdala and medial thalamus (27, 28). Increased output from the amygdala to the thalamus, suggested by these previous findings, is particularly interesting since an increase in amygdala metabolism occurs both during episodes of severe major depressive disorder symptoms and after depressive symptoms have resolved (27). These observations suggest the presence of both structural and functional abnormalities in subcortical limbic structures in major depressive disorder.
All but one of the major depressive disorder subjects in the present study received antidepressant drugs. In animals, treatment with antidepressants increases the rate of neurogenesis, migration, and differentiation in the subgranular zone of the hippocampus and in the marginal ventricular zone (29). These adult-born neurons can form functional connections (30). However, the thalamus has not been observed to be a site of antidepressant-induced neurogenesis. Furthermore, approximately 50% of the schizophrenia subjects in the present study had a history of antidepressant treatment, and neither mediodorsal nor anteroventral/anteromedial neuron numbers were elevated in these patients. These data suggest that it is unlikely that antidepressant-induced neurogenesis is responsible for the increase in neuron number in the limbic thalamus in major depressive disorder.
The increase in limbic thalamic neuron numbers in major depressive disorder may be caused by an abnormal neurodevelopmental process. Elevations in mediodorsal and anteroventral/anteromedial neuron numbers could reflect a developmental problem such as an accentuated neuronal birth rate or, alternatively, the survival of excess numbers of neurons. Our data provide no information about which thalamic neuron population (projection neuron or interneuron) is abnormal in major depressive disorder. An elevated number of projection neurons could result in an excess of excitatory glutamatergic output from the thalamus to the cortex in major depressive disorder. Conversely, if numbers of inhibitory interneurons in the limbic thalamus were elevated in major depressive disorder, greater than normal inhibition of thalamic output to the cortex might be present. In humans, many thalamic interneurons are generated in the ganglionic eminence of the telencephalon. Some of these neurons subsequently migrate into the thalamus to become GABAergic interneurons (31, 32). Further studies are needed to determine whether the “projection” or “interneuron” population is abnormal in the thalamus of major depressive disorder patients in order to better understand the developmental implications and potential pathophysiological effects of thalamic abnormalities in this disorder.
Previous studies have documented abnormalities in the limbic cortex in major depressive disorder, including decreased volume of the medial orbitofrontal cortex (33), hippocampus (9, 10), and prefrontal cortex (7). Also, there is a decreased density of glial cells in the dorsolateral prefrontal cortex (34) and a decrease in glial cell numbers in subgenual cingulate cortex area 24 (35). These anatomical abnormalities in the cortical target areas of the mediodorsal and anteroventral/anteromedial nuclei may be the result of a decreased cortical input from the thalamus caused by excessive GABA interneuron inhibition of thalamocortical neurotransmission. Decreased input to the cortex could minimize the need for glial support of cortical neurotransmission, affecting cortical glial populations. Alternatively, cortical pathology may be the result of an elevation of excitatory input to the cortex from the thalamus caused by excess numbers of glutamatergic projection neurons. Excess input to the cortex could promote excitotoxicity to cortical cells, including glia. Determination of which populations of thalamic neurons are abnormal in major depressive disorder will be an important goal for future studies.
It has been proposed that a lack of coordinated activity within the limbic system is responsible for some of the symptoms of major depressive disorder (27, 28, 36). Because the thalamus is a critical connection between the amygdala and the prefrontal cortex, it is well positioned for involvement in major depressive disorder pathophysiology. In particular, GABAergic neurotransmission in the thalamus has a substantial effect on prefrontal cortical metabolism (37). Excess GABAergic inhibition in the medial thalamus results in decreased frontal cortical metabolism and behavioral deficits such as attention neglect and learning deficits, behaviors consistent with the cognitive symptoms of severe depression. The present data support the hypothesis that thalamic anatomical abnormalities are involved in limbic system dysfunction in major depressive disorder.
In the schizophrenia group, mediodorsal neuron number was normal. These data differ from previous studies in which mediodorsal neuron number was reduced in schizophrenia subjects (16, 38–40). The number of neurons in the comparison subjects in the present study is similar to our previous study (16), and similar to estimates reported by others (39–42). This observation indicates that the present nonpsychiatric comparison group is not different from other comparison groups with respect to mediodorsal neuron number. In the four previous studies (16, 38–40) in which mediodorsal cell numbers were reduced in schizophrenia brains, the average age of the subjects was over 60 years. In the present study, the mean age of subjects was 46 years. Because the present data indicate a normal number of limbic thalamic neurons in schizophrenia, additional studies will be needed to investigate the effects of age on thalamic neuron numbers in schizophrenia.
The number of neurons and the volume in the mediodorsal and anteroventral/anteromedial nuclei were normal in subjects with bipolar disorder. Exclusion of four subjects diagnosed with bipolar II disorder, or exclusion of subjects without psychotic features, did not change the overall results. However, it is notable that several bipolar subjects had highly elevated mediodorsal and anteroventral/anteromedial neuron numbers similar to major depressive disorder subjects. Additional studies may identify clinical subgroups of bipolar disorder patients that share some of the anatomical characteristics of major depressive disorder.
The present results should be interpreted with certain limitations in mind. The thalamic tissue blocks were processed in a unique manner consisting of deparaffinization before cryoprotection and sectioning, which may have contributed to a z-axis shrinkage. However, we did not observe significant differences in z-axis thickness between the four subject groups, and our conclusions are unlikely to have been biased by this variable. All anatomical findings, including those of the present study, need to be replicated to verify the uniformity of experimental findings. In particular, volume measurements of the mediodorsal and anteroventral/anteromedial nuclei and of the total thalamus need to be performed with an increased sample size to investigate the tendencies observed in the present study. In the present study, a strength was the inclusion of different neuropsychiatric groups to allow investigation of disease-specific effects. Although the present study represents one of the largest neuropsychiatric cohorts analyzed by rigorous stereological techniques (N=45), the sample size was still not large enough to provide sufficient statistical power to fully characterize potentially important variables such as age, gender, and laterality. The Stanley Foundation brain collection provides a history of drug treatment for all subjects, allowing modest, but still limited, insight into whether the experimental findings of the present study are related to disease or drug treatment. Additional studies will be needed to fully characterize the influences of drug and other therapies. It is thus critical that the present findings be replicated in other cohorts of depressed patients to determine whether the current major depressive disorder population is representative of the disease.
In conclusion, the present study provides evidence for an elevation in neuron number in the limbic thalamus in major depressive disorder, and a normal number of neurons in schizophrenia and bipolar cases. These findings add to the evolving consensus that there is substantial brain pathology in major depressive disorder (43). The causes and implications of this novel neuroanatomical finding remain to be elucidated.
![]() |
![]() |
Received March 7, 2003; revisions received June 11 and Oct. 28, 2003; accepted Nov. 7, 2003. From the Central Texas Veterans Health Care System; the Department of Psychiatry and Behavioral Science Neuropsychiatry Research Program, Scott & White Hospital, Temple, Tex.; and the Department of Psychiatry, University of Texas Southwestern Medical School, Dallas. Address reprint requests to Dr. Young, Central Texas Veterans Health Care System, Neuropsychiatry Research Program (151N), 1901 S. First St., Temple, TX 76504; [email protected] (e-mail).Supported by NIMH grant MH-59145 and funding from the Veterans Administration; the Scott, Sherwood and Brindley Foundation; and the Theodore and Vada Stanley Foundation.The authors thank the Stanley Foundation Brain Bank and Drs. M. Knable, E.F. Torrey, M. Webster, and R. Yolken for providing tissue used in this experiment as well as Debbie Stitt and Jeana Ulses for their technical assistance.
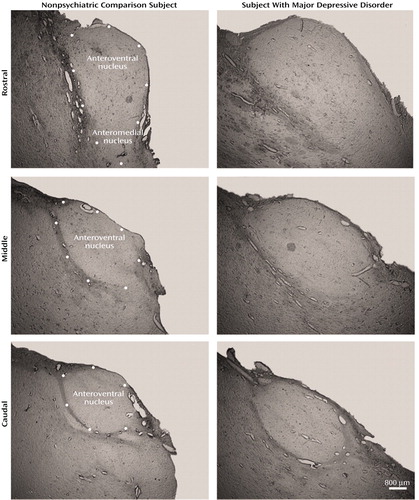
Figure 1. Darkfield Photomicrographs of Anteroventral/Anteromedial Thalamic Nuclei From Representative Brain Specimens of a Subject With No Psychopathology and a Subject With Major Depressive Disordera
aDots represent the neuroanatomical boundaries of the nucleus.
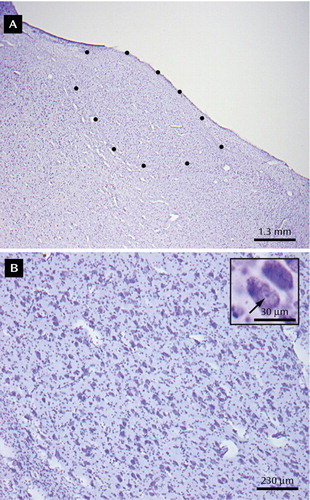
Figure 2. Anteroventral Thalamic Tissue Nissl-Stained With Cresyl Violeta
aThe low-power photomicrograph (image A) illustrates the neuroanatomical boundaries of the anteroventral nucleus in a nonpsychiatric comparison brain. The border of the nucleus is outlined with dots. The higher-power photomicrograph (image B) illustrates the quality of the tissue and Nissl-staining and the morphology of neurons. Neurons with a nucleolus were counted when the nucleolus was in focus within the confines of a three-dimensional counting box. The insert within image B illustrates a neuron with a nucleolus (arrow) in focus.
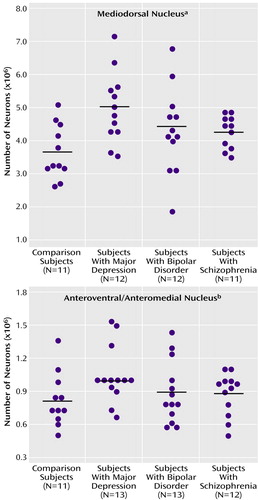
Figure 3. Thalamic Nuclei Neuron Numbers From Brain Specimens of Subjects With Major Depressive Disorder, Bipolar Disorder, Schizophrenia, and No Psychopathologya
aAnalysis of variance (ANOVA) indicated that neuron number differed among the four groups, but post hoc Dunnett’s tests showed that only the major depressive disorder group significantly differed from the nonpsychiatric comparison group. Analysis of covariance (ANCOVA) showed that the main effect of diagnosis remained after age, gender, laterality, and postmortem interval were entered as covariates.
bANOVA indicated that neuron number did not differ among the four groups. However, ANCOVA (with age, gender, laterality, and postmortem interval as covariates) followed by post hoc contrast tests revealed a significant difference between the major depressive disorder group and the nonpsychiatric comparison subjects.
1. Mufson EJ, Mesulam MM: Thalamic connections of the insula in the rhesus monkey and comments on the paralimbic connectivity of the medial pulvinar nucleus. J Comp Neurol 1984; 227:109–120Crossref, Medline, Google Scholar
2. Giguere M, Goldman-Rakic PS: Mediodorsal nucleus: areal, laminar, and tangential distribution of afferents and efferents in the frontal lobe of rhesus monkeys. J Comp Neurol 1988; 277:195–213Crossref, Medline, Google Scholar
3. Price JL: Prefrontal cortical networks related to visceral function and mood. Ann NY Acad Sci 1999; 877:383–396Crossref, Medline, Google Scholar
4. Vogt BA, Pandya DN, Rosene DL: Cingulate cortex of the rhesus monkey, I: cytoarchitecture and thalamic afferents. J Comp Neurol 1987; 262:256–270Crossref, Medline, Google Scholar
5. Porrino LJ, Crane AM, Goldman-Rakic PS: Direct and indirect pathways from the amygdala to the frontal lobe in rhesus monkeys. J Comp Neurol 1981; 198:121–136Crossref, Medline, Google Scholar
6. LeDoux JE: Emotion circuits in the brain. Annu Rev Neurosci 2000; 23:155–184Crossref, Medline, Google Scholar
7. Botterton KN, Raichle ME, Drevets WC, Heath AC, Todd RD: Volumetric reduction in left subgenual prefrontal cortex in early onset depression. Biol Psychiatry 2002; 51:342–344Crossref, Medline, Google Scholar
8. Rajkowska G, Halaris A, Selemon LD: Reductions in neuronal and glial density characterize the dorsolateral prefrontal cortex in bipolar disorder. Biol Psychiatry 2002; 49:741–752Crossref, Google Scholar
9. Mervaala E, Fohr J, Kononen M, Valkonen-Korhonen M, Vainio P, Partanen K, Partanen J, Tiihonen J, Viinamaki H, Karjalainen AK, Lehtonen J: Quantitative MRI of the hippocampus and amygdala in severe depression. Psychol Med 2000; 30:117–125Crossref, Medline, Google Scholar
10. Bremner JD, Narayan M, Anderson ER, Staib LH, Miller HL, Charney DS: Hippocampal volume reduction in major depression. Am J Psychiatry 2000; 157:115–117Link, Google Scholar
11. Kumar A, Thomas A, Lavretsky H, Yue K, Huda A, Curran J, Venkatraman T, Estanol L, Mintz J, Mega M, Toga A: Frontal white matter biochemical abnormalities in late-life major depression detected with proton magnetic resonance spectroscopy. Am J Psychiatry 2002; 159:630–636Link, Google Scholar
12. Pillai JJ, Friedman L, Stuve TA, Trinidad S, Jesberger JA, Lewin JS, Findling RL, Swales TP, Schulz SC: Increased presence of white matter hyperintensities in adolescent patients with bipolar disorder. Psychiatry Res 2002; 114:51–56Crossref, Medline, Google Scholar
13. Harrison PJ: The neuropathology of primary mood disorder. Brain 2002; 125:1428–1449Crossref, Medline, Google Scholar
14. Torrey EF, Webster M, Knable M, Johnston N, Yolken RH: The Stanley Foundation brain collection and Neuropathology Consortium. Schizophr Res 2000; 44:151–155Crossref, Medline, Google Scholar
15. Jones EG: A description of the human thalamus, in Thalamus, vol II. Edited by Steriade M, Jones EG, McCormick DA. New York, Elsevier Science, 1997, pp 425–499Google Scholar
16. Young KA, Manaye KF, Liang C-L, Hicks PB, German DC: Reduced number of mediodorsal and anterior thalamic neurons in schizophrenics. Biol Psychiatry 2000; 47:944–953Crossref, Medline, Google Scholar
17. Frodl T, Meisenzahl E, Zetzsche T, Bottlender R, Born C, Groll C, Jager M, Leinsinger G, Hahn K, Moller HJ: Enlargement of the amygdala in patients with a first episode of major depression. Biol Psychiatry 2002; 51:708–714Crossref, Medline, Google Scholar
18. Tebartz van Elst L, Woermann F, Lemieux L, Trimble MR: Increased amygdala volumes in female and depressed humans: a quantitative magnetic resonance imaging study. Neurosci Lett 2000; 281:103–106Crossref, Medline, Google Scholar
19. Altshuler L, Bartzokis G, Grieder T, Curran J, Jimenez T, Leight K, Wilkins J, Gerner R, Mintz J: An MRI study of temporal lobe structures in men with bipolar disorder or schizophrenia. Biol Psychiatry 2000; 48:147–162Crossref, Medline, Google Scholar
20. Krishnan KR, Doraiswamy PM, Lurie SN, Figiel GS, Husain MM, Boyko OB, Ellinwood EH Jr, Nemeroff CB: Pituitary size in depression. J Clin Endocrinol Metab 1991; 72:256–259Crossref, Medline, Google Scholar
21. Raadsheer FC, Hoogendijk WJ, Stam FC, Tilders FJ, Swaab DF: Increased numbers of corticotropin-releasing hormone expressing neurons in the hypothalamic paraventricular nucleus of depressed patients. Neuroendocrinology 1994; 60:436–444Crossref, Medline, Google Scholar
22. Purba JS, Hoogendijk WJ, Hofman MA, Swaab DF: Increased number of vasopressin- and oxytocin-expressing neurons in the paraventricular nucleus of the hypothalamus in depression. Arch Gen Psychiatry 1996; 53:137–143Crossref, Medline, Google Scholar
23. Zhou JN, Riemersma RF, Unmehopa UA, Hoogendijk WJ, van Heerikhuize JJ, Hofman MA, Swaab DF: Alterations in arginine vasopressin neurons in the suprachiasmatic nucleus in depression. Arch Gen Psychiatry 2001; 58:655–662Crossref, Medline, Google Scholar
24. Ono K, Niimi K: Direct projections of the hypothalamic nuclei to the thalamic mediodorsal nucleus in the cat. Neurosci Lett 1985; 57:283–287Crossref, Medline, Google Scholar
25. Cornwall J, Phillipson OT: Afferent projections to the dorsal thalamus of the rat as shown by retrograde lectin transport, I: the mediodorsal nucleus. Neuroscience 1988; 24:1035–1049Crossref, Medline, Google Scholar
26. Baumann B, Danos P, Krell D, Diekmann S, Leschinger A, Stauch R, Wurthmann C, Bernstein HG, Bogerts B: Reduced volume of limbic system-affiliated basal ganglia in mood disorders: preliminary data from a postmortem study. J Neuropsychiatry Clin Neurosci 1999; 11:71–78Crossref, Medline, Google Scholar
27. Drevets WC: Neuroimaging studies of mood disorders. Biol Psychiatry 2000; 48:813–829Crossref, Medline, Google Scholar
28. Drevets WC, Price JL, Bardgett ME, Reich T, Todd RD, Raichle ME: Glucose metabolism in the amygdala in depression: relationship to diagnostic subtype and plasma cortisol levels. Pharmacol Biochem Behav 2002; 71:431–447Crossref, Medline, Google Scholar
29. Duman RS, Nakagawa S, Malberg J: Regulation of adult neurogenesis by antidepressant treatment. Neuropsychopharmacology 2001; 25:836–844Crossref, Medline, Google Scholar
30. Shors TJ, Miesegaes G, Beylin A, Zhao M, Rydel T, Gould E: Neurogenesis in the adult is involved in the formation of trace memories. Nature 2001; 410:372–376Crossref, Medline, Google Scholar
31. Letinic K, Kostovic I: Transient fetal structure, the gangliothalamic body, connects telencephalic germinal zone with all thalamic regions in the developing human brain. J Comp Neurol 1997; 384:373–395Crossref, Medline, Google Scholar
32. Letinic K, Rakic P: Telencephalic origin of human thalamic GABAergic neurons. Nat Neurosci 2001; 4:931–936Crossref, Medline, Google Scholar
33. Bremner JD, Vythilingam M, Vermetten E, Nazeer A, Adil J, Khan S, Staib LH, Charney DS: Reduced volume of orbitofrontal cortex in major depression. Biol Psychiatry 2002; 51:273–279Crossref, Medline, Google Scholar
34. Cotter D, Mackay D, Chana G, Beasley C, Landau S, Everall IP: Reduced neuronal size and glial cell density in area 9 of the dorsolateral prefrontal cortex in subjects with major depressive disorder. Cereb Cortex 2002; 12:386–394Crossref, Medline, Google Scholar
35. Ongur D, Drevets WC, Price L: Glial reduction in subgenual prefrontal cortex in mood disorders. Proc Natl Acad Sci USA 1998; 95:13290–13295Crossref, Medline, Google Scholar
36. Mayberg HS, Liotti M, Brannan SK, McGinnis S, Mahurin RK, Jerabek PA, Silva JA, Tekell JL, Martin CC, Lancaster JL, Fox PT: Reciprocal limbic-cortical function and negative mood: converging PET findings in depression and normal sadness. Am J Psychiatry 1999; 156:675–682Abstract, Google Scholar
37. Young KA, Hicks PB, Randall PK, Wilcox RE: Behavioral and frontal cortical metabolic effects of apomorphine and muscimol microinjections into the mediodorsal thalamic nucleus. J Neural Transm (Gen Sect) 1994; 98:119–132Crossref, Medline, Google Scholar
38. Pakkenberg B: Pronounced reduction of total neuron number in mediodorsal thalamic nucleus and nucleus accumbens in schizophrenics. Arch Gen Psychiatry 1990; 47:1023–1028Crossref, Medline, Google Scholar
39. Popken GJ, Bunney WE Jr, Potkin SG, Jones EG: Subnucleus-specific loss of neurons in medial thalamus of schizophrenics. Proc Natl Acad Sci USA 2000; 97:9276–9280Crossref, Medline, Google Scholar
40. Byne W, Buchsbaum MS, Mattiace LA, Hazlett EA, Kemether E, Elhakem SL, Purohit DP, Haroutunian V, Jones L: Postmortem assessment of thalamic nuclear volumes in subjects with schizophrenia. Am J Psychiatry 2002; 159:59–65Link, Google Scholar
41. Xuereb JH, Perry RH, Candy JM, Perry EK, Marshall E, Bonham JR: Nerve cell loss in the thalamus in Alzheimer’s disease and Parkinson’s disease. Brain 1991; 114:1363–1379Crossref, Medline, Google Scholar
42. Harding AJ, Halliday GM, Cullen K: Practical considerations for the use of the optical disector in estimating neuronal number. J Neurosci Methods 1994; 51:83–89Crossref, Medline, Google Scholar
43. Vastag B: Decade of work shows depression is physical. JAMA 2002; 287:1787–1788Crossref, Medline, Google Scholar