An fMRI Stroop Task Study of Ventromedial Prefrontal Cortical Function in Pathological Gamblers
Abstract
OBJECTIVE: Function of the ventromedial prefrontal cortex has been implicated in impulse control. The authors used the Stroop paradigm to test attention and response inhibition during the presentation of congruent and incongruent stimuli in male pathological gamblers and a group of comparison subjects. METHOD: Event-related functional magnetic resonance imaging was used to examine ventromedial prefrontal cortex function during Stroop performance. RESULTS: In response to infrequent incongruent stimuli, pathological gamblers demonstrated decreased activity in the left ventromedial prefrontal cortex relative to the comparison subjects. Both groups demonstrated similar activity changes in multiple brain regions, including activation of the dorsal anterior cingulate and dorsolateral frontal cortex. CONCLUSIONS: Pathological gamblers share many neural correlates of Stroop task performance with healthy subjects but differ in a brain region previously implicated in disorders characterized by poor impulse control.
Despite significant prevalence estimates and associations with adverse consequences (1), pathological gambling has received relatively little study, particularly in its neurobiological underpinnings (2, 3). Function of the ventromedial prefrontal cortex has been widely implicated in impulse regulation (4–9). Previously, we found that pathological gamblers exhibit decreased activity in the ventromedial prefrontal cortex during presentation of gambling cues (10). Our group has used an event-related Stroop paradigm (involving the infrequent presentation of mismatched color-word stimuli and frequent presentation of matched color-word stimuli) in functional magnetic resonance imaging (fMRI) studies to identify the neural correlates of the Stroop effect (11, 12). Since the incongruent stimuli presentations in the Stroop paradigm require response inhibition and pathological gambling involves impaired inhibitory control of gambling behaviors, we predicted that pathological gamblers would differ from comparison subjects in ventromedial prefrontal cortex activity during Stroop task performance.
Method
This research was approved by the Yale Human Investigations Committee, and all subjects provided written informed consent. All subjects were male, 18–65 years of age, native English speakers, and without past or present major neurological injury or illness. Two pathological gamblers were left-handed, and all other subjects were right-handed. Pathological gamblers had an average South Oaks Gambling Screen (13) score of 12.62 (SD=3.93). Pathological gamblers met DSM-IV-TR criteria for pathological gambling and were free of any other active axis I disorder except nicotine dependence. Comparison subjects were free of any active axis I disorder. Diagnostic information was determined by using the Structured Clinical Interview for DSM-IV (SCID) (14) for the pathological gamblers but not the comparison subjects. The Structured Clinical Interview for Pathological Gambling (unpublished, available from the first author upon request), a SCID-compatible module based on DSM-IV criteria, was used to confirm the diagnosis of pathological gambling.
Data were obtained from 13 pathological gamblers and 11 healthy comparison subjects. Pathological gamblers and comparison subjects were similar in age (mean=35.15 years [SD=7.97] and 29.00 years [SD=7.81], respectively; χ2=3.62, df=1, p=0.07). Six pathological gamblers were considered nicotine dependent (Fagerstrom Test for Nicotine Dependence scores ≥5); no comparison subjects reported smoking. All subjects were high school graduates. The racial/ethnic composition for the pathological gamblers was Caucasian (N=8), African American (N=4), and Hispanic (N=1); among the comparison subjects, nine were Caucasian, one was Hispanic, and one was of unknown ethnicity. All subjects denied psychoactive drug use with the exception of nicotine or caffeine for the 72 hours before the fMRI protocol.
Subjects performed the Stroop task as previously described (11, 12). Briefly, 9–10 runs of 102 stimuli were presented. Each stimulus was presented for 1300 msec, with an interstimulus interval of 350 msec. Incongruent stimuli were presented pseudorandomly every 13–16 congruent stimuli (i.e., 21.45–26.4 seconds apart). Subjects practiced aloud the task for one or two runs before scanning and silent naming was performed during imaging to minimize motion artifact. Performance was assessed following scanning to measure reaction time (difference in response times to incongruent versus congruent stimuli) and percentage of correct responses to incongruent stimuli (6, 11, 12).
Image Acquisition
Images were obtained with a 1.5-T GE Signa MRI system equipped with an echo-planar imaging system, a standard quadrature head coil, and a T2*-sensitive gradient-recalled, single-shot, echo-planar pulse sequence (6, 11, 12). Conventional T1-weighted spin-echo sagittal anatomic images (TE=11 msec, TR=667 msec, field of view=24 cm, slice thickness=5 mm, gap=1 mm, 256×128 data matrix) were obtained for slice localization. Ten T1-weighted oblique axial slices (TE=13 msec, TR=500 msec, field of view=40×40 cm, gap=1 mm, 256×192 data matrix) were obtained parallel to the plane transecting the anterior and posterior commissures (6, 11, 12). Functional images were obtained by using a single-shot, echo-planar gradient-echo sequence (TR=1.65 seconds; TE=60 msec; flip angle=60°; field of view=40×20 cm; slice thickness=7 mm, with skip varying between 0.5 and 2 mm to keep strict correspondence to Talairach space; in-plane resolution=3.12×3.12 mm; number of images per slice=102) at the same locations.
Data Analysis
Data were motion corrected for three translational directions and three possible rotations (15). Runs with motion in excess of 1.5 mm displacement and 2 degrees rotation were rejected. Similar numbers of runs per subject were used from pathological gamblers (mean=8.69, SD=1.65) and comparison subjects (mean=9.00, SD=0.89) (χ2=0.30, df=1, p=0.59). Corrected images were spatially filtered by using a Gaussian filter with a full width at half maximum of 6.25 mm.
T1-weighted axial anatomical images and corresponding functional images for each subject were transformed into a common stereotactic space by piece-wise linear warping. Pixel-based changes in fMRI signal associated with incongruent stimuli presentation were calculated as follows. The average signal of the six images preceding incongruent stimuli presentation was subtracted from each of the eight images following the incongruent stimuli at each pixel by using voxel-based statistical mapping methodologies to generate activation time courses as described previously (6, 10–12). Linear contrasts between conditions were used to calculate t values for each voxel and data randomizations using nonparametric statistics employed to create distributions with which to assign statistical significance to observed between-group differences at each voxel location (p-map generation). Time course p-maps (at p=0.05 with voxels in contiguous sets of 20 voxels each) were generated to identify between-group differences in ventromedial prefrontal cortex activity (12). The largest between-group differences were observed in the third and fourth images. Therefore, the average signal of the six images preceding incongruent stimuli presentation was subtracted from the average of the third and fourth images following the incongruent stimuli at each pixel at a significance threshold of p=0.01 for each subject group and between subject groups (11, 12). Significance was assigned by using voxel-based data randomizations as described previously (6, 10–12). Within-group correlation analyses were performed to investigate the potential effects of age and smoking status (Fagerstrom score) on observed between-group differences in signal activity. To ensure that signal arising at the lower part of the brain was not significantly impacted by susceptibility artifact, the signal from lower brain regions was evaluated in all cases to verify that it was >75% of the signal from cortical regions in more uniform areas. SUPER-ANOVA (Abacus Concepts, Inc.; Berkeley, Calif.) was used to perform t tests to analyze data from Stroop performance (reaction time and correct responses) (11, 12).
Results
The pathological gamblers and comparison subjects performed similarly on the Stroop task in terms of incorrect response percentage (mean=12.09% [SD=16.34%] and 7.14% [SD=10.10%], respectively; χ2=0.70, df=1, p=0.41) and reaction times to incongruent stimuli (mean=215.77 msec [SD=126.20] and 197.55 msec [SD=66.77]; χ2=0.14, df=1, p=0.71). Following presentation of incongruent stimuli, pathological gamblers and comparison subjects demonstrated activity changes in many of the same brain regions (Figure 1, Table 1). Activations observed for both groups involved the dorsal anterior cingulate, right middle and inferior frontal gyri, bilateral inferior frontal gyri, right insula, and right thalamus. Both groups demonstrated decreased activity in the ventral anterior cingulate. Pathological gamblers and comparison subjects were distinguished by differences in signal change in the left ventromedial prefrontal cortex (Figure 1, Table 1). This region of the ventromedial prefrontal cortex involves the left middle and superior frontal gyri and borders the superior frontal sulcus laterally and the orbitofrontal cortex ventrally. The difference in signal change was attributable largely to decreased activity in pathological gamblers, with a lesser contribution from increased activity in healthy subjects (observed in comparison maps of less stringent significance thresholds than p<0.01 [not shown]). Correlation analyses suggest that the between-group difference in the left ventromedial prefrontal cortex was not attributable to between-group differences in age or smoking status (data not shown).
Discussion
Past investigations have reported cognitive biases (16) and attentional deficits (17) in pathological gamblers, and our group has found decreased activity in the ventromedial prefrontal cortex in pathological gamblers during gambling cue presentation (10). The Stroop paradigm was selected to investigate aspects of attention and response inhibition and ventromedial prefrontal cortex function in pathological gamblers and healthy comparison subjects. Behavioral performance measures of the Stroop task were similar across groups, although sensitivity is decreased by the sizable variance. Task-related brain activities were largely similar across groups, suggesting that brain functioning and its behavioral consequences were similar in pathological gamblers and healthy subjects. In particular, both groups demonstrated activation of the dorsolateral prefrontal cortex and dorsal anterior cingulate, brain regions previously implicated in conflict monitoring and cognitive control and identified as activated in the Stroop paradigm (6, 11, 12, 18–20).
The most robust between-group difference was observed in the left ventromedial prefrontal cortex, a region involving the superior aspect of the orbitofrontal cortex. The ventromedial prefrontal cortex has been implicated in decision making (4), and its orbitofrontal component is thought to participate in the processing of rewards during the expectancy and experiencing of monetary gains or losses (21). The orbitofrontal cortex also appears to become activated when there is insufficient information to determine the appropriate course of action or when decision-making action requires suppression of previously rewarded responses (22). Individuals with impaired impulse control have demonstrated orbitofrontal cortex/ventromedial prefrontal cortex dysfunction (5–8), including during Stroop task performance (23). The finding of decreased orbitofrontal cortex/ventromedial prefrontal cortex activation in pathological gamblers during incongruent stimulus presentation suggests dysfunction in this brain region. Further investigations are needed to examine directly the extent to which ventromedial prefrontal cortex dysfunction underlies specific aspects of the pathology of pathological gambling, including poor decision making and rapid temporal discounting of rewards (24). Future investigations should address limitations of the present study by using a larger, more diverse sample (with women); matching precisely for age and race/ethnicity; examining the potential effect of tobacco use; obtaining structured clinical interview data on all subjects; and correlating on-line Stroop task performance with measures of brain activity.
![]() |
Received Oct. 29, 2002; revision received Jan. 13, 2003; accepted Jan. 17, 2003. From the Department of Psychiatry and the Department of Diagnostic Radiology, Yale University School of Medicine; the Connecticut Mental Health Center; and the West Haven VA Hospital, West Haven, Conn. Address reprint requests to Dr. Potenza, Yale University School of Medicine, Connecticut Mental Health Center, Room S-104, 34 Park St., New Haven, CT 06519; [email protected] (e-mail). Supported by Young Investigator Awards from the National Alliance for Research in Schizophrenia and Depression (Drs. Potenza and Blumberg); a Drug Abuse Research Scholar Program in Psychiatry Award from APA (Dr. Potenza); National Institute on Drug Abuse grant DA-00366 (Dr. Potenza); funding from the National Center for Responsible Gaming (Dr. Potenza), the Stanley Foundation (Dr. Blumberg), the Charles A. Dana Foundation (Dr. Peterson), and the Suzanne Crosby Murphy Endowment at Columbia University College of Physicians and Surgeons (Dr. Peterson); VA grants for Research Career Development (Dr. Blumberg) and Merit Review (Dr. Blumberg) and the Research Enhancement Award Program (Drs. Leung and Blumberg); and NIMH grants MH-01232 and MH-59139 (Dr. Peterson). The authors thank Hedy Sarofin, Cheryl McMurray, Terry Hickey, Mary Wilber, Erin Reutenauer, and Kathleen Colonese for technical support.
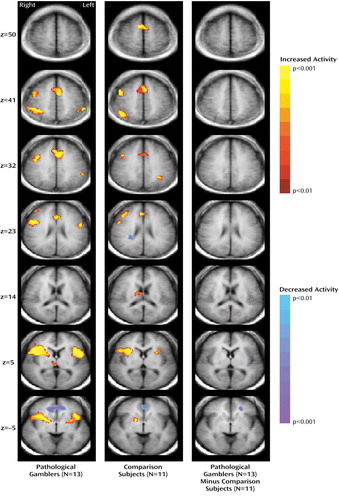
Figure 1. Activation Differences in Pathological Gamblers and Comparison Subjects Following Presentation of Incongruent Stimuli (Stroop task)
1. Potenza MN, Kosten TR, Rounsaville BJ: Pathological gambling. JAMA 2001; 286:141–144Crossref, Medline, Google Scholar
2. Eber GB, Shaffer HJ: Trends in bio-behavioral gambling studies research: quantifying citations. J Gambl Stud 2000; 16:461–467Crossref, Medline, Google Scholar
3. Potenza MN: The neurobiology of pathological gambling. Semin Clin Neuropsychiatry 2001; 6:217–226Crossref, Medline, Google Scholar
4. Bechara A: Neurobiology of decision-making: risk and reward. Semin Clin Neuropsychiatry 2001; 6:205–216Crossref, Medline, Google Scholar
5. Siever LJ, Buchsbaum MS, New AS, Spiegel-Cohen J, Wei T, Hazlett EA, Sevin E, Nunn M, Mitropoulou V: d,l-Fenfluramine response in impulsive personality disorder assessed with [18F]fluorodeoxyglucose positron emission tomography. Neuropsychopharmacology 1999; 20:413–423Crossref, Medline, Google Scholar
6. Blumberg HP, Stern E, Ricketts S, Martinez D, de Asis J, White T, Epstein J, Isenberg N, McBride PA, Kemperman I, Emmerich S, Dhawan V, Eidelberg D, Kocsis JH, Silbersweig DA: Rostral and orbital prefrontal cortex dysfunction in the manic state of bipolar disorder. Am J Psychiatry 1999; 156:1986–1988Abstract, Google Scholar
7. Pietrini P, Guazzelli M, Basso G, Jaffe K, Grafman J: Neural correlates of imaginal aggressive behavior assessed by positron emission tomography in healthy subjects. Am J Psychiatry 2000; 157:1772–1781Link, Google Scholar
8. New AS, Hazlett EA, Buchsbaum MS, Goodman M, Reynolds D, Mitropoulou V, Sprung L, Shaw RB Jr, Konigsberg H, Platholi J, Silverman J, Siever LJ: Blunted prefrontal cortical 18-fluorodeoxyglucose positron emission tomography response to meta-chlorophenylpiperazine in impulsive aggression. Arch Gen Psychiatry 2002; 59:621–629Crossref, Medline, Google Scholar
9. Best M, Williams JM, Coccaro EF: Evidence for a dysfunctional prefrontal circuit in patients with an impulsive aggressive disorder. Proc Natl Acad Sci USA 2002; 99:8448–8453Crossref, Medline, Google Scholar
10. Potenza MN, Steinberg MA, Skudlarski P, Fulbright RK, Lacadie CM, Wilber MK, Rounsaville BJ, Gore JC, Wexler BE: Gambling urges in pathological gambling: a functional magnetic resonance imaging study. Arch Gen Psychiatry 2003; 60:828–836Crossref, Medline, Google Scholar
11. Leung H-C, Skudlarski P, Gatenby JC, Peterson BS, Gore JC: An event-related functional MRI study of the Stroop color word interference task. Cereb Cortex 2000; 10:552–560Crossref, Medline, Google Scholar
12. Peterson BS, Kane MJ, Alexander GM, Lacadie C, Skudlarski P, Leung H-C, May J, Gore JC: An event-related functional MRI study comparing interference effects in the Simon and Stroop task. Cogn Brain Res 2002; 13:427–440Crossref, Medline, Google Scholar
13. Lesieur HR, Blume SB: The South Oaks Gambling Screen (SOGS): a new instrument for the identification of pathological gamblers. Am J Psychiatry 1987; 144:1184–1188Link, Google Scholar
14. First MB, Spitzer RL, Gibbon M, Williams JBW: Structured Clinical Interview for DSM-IV Disorders, Patient Edition (SCID-P). New York, New York State Psychiatric Institute, Biometrics Research, 1995Google Scholar
15. Friston KJ, Holmes AP, Worsley KJ, Pooline J-B, Frith CD, Frackowiak RSJ: Statistical parametric maps in functional imaging: a general linear approach. Hum Brain Mapp 1995; 2:184–210Google Scholar
16. McCusker CG, Gettings B: Automaticity of cognitive biases in addictive behaviours: further evidence with gamblers. Br J Clin Psychol 1997; 36(part 4):543–554Google Scholar
17. Rugle L, Melamed L: Neuropsychological assessment of attention problems in pathological gamblers. J Nerv Ment Dis 1993; 181:107–112Crossref, Medline, Google Scholar
18. Bush G, Luu P, Posner MI: Cognitive and emotional influences in anterior cingulate cortex. Trends Cogn Sci 2000; 4:215–222Crossref, Medline, Google Scholar
19. Peterson BS, Skudlarski P, Gatenby JC, Zhang H, Anderson AW, Gore JC: An fMRI study of Stroop word-color interference: evidence for cingulate subregions subserving multiple distributed attentional systems. Biol Psychiatry 1999; 45:1237–1258Crossref, Medline, Google Scholar
20. van Veen V, Cohen JD, Botvinick MM, Stenger VA, Carter CS: Anterior cingulate cortex, conflict monitoring, and levels of processing. Neuroimage 2001; 14:1302–1308Crossref, Medline, Google Scholar
21. Breiter HC, Aharon I, Kahneman D, Dale A, Shizgal P: Functional imaging of neural responses to expectancy and experience of monetary gains and losses. Neuron 2001; 30:619–639Crossref, Medline, Google Scholar
22. Elliott R, Dolan RJ, Frith CD: Dissociable functions in the medial and lateral orbitofrontal cortex: evidence from human neuroimaging studies. Cereb Cortex 2000; 10:308–317Crossref, Medline, Google Scholar
23. Blumberg HP, Leung HC, Skudlarski P, Lacadie CM, Fredericks CA, Harris BC, Charney DS, Gore JC, Krystal JH, Peterson BS: A functional magnetic resonance imaging study of bipolar disorder: state- and trait-related dysfunction in ventral prefrontal cortices. Arch Gen Psychiatry 2003; 60:601–609Crossref, Medline, Google Scholar
24. Bechara A: Risky business: emotion, decision-making, and addiction. J Gambl Stud 2003; 19:23–51Crossref, Medline, Google Scholar