Circulating miRNA Biomarkers for Schizophrenia?
The Psychiatric Genomics Consortium recently derived a significant ensemble of common variants through its landmark mega genome-wide association study of schizophrenia (1). While this provides the foundations for variant profiling and the emergence of trait biomarkers, the predictive power of polygenic risk scoring defined by receiver-operating characteristic curve analysis is reported to be below the threshold for clinical utility (2). As an alternative, other nucleic acids, proteins, and various metabolites could support predictive function or serve as “state” biomarkers in the personalized management of schizophrenia (3).
In this issue, Wei et al. (4) explore the potential of circulating serum microRNA (miRNA) levels to inform schizophrenia diagnosis and antipsychotic drug-associated remission. The authors extracted RNA from plasma collected from a large group of subjects with psychiatric illness (N=726)—mostly confirmed cases of schizophrenia—and control subjects (N=400) matched for age, gender, smoking history, and education level. The analysis of these samples was divided into discovery, validation, and follow-up cohorts (Figure 1). In the discovery phase, plasma RNA from 150 case subjects and 150 control subjects were each pooled and subjected to both small RNA sequencing (sRNA-seq) and miRNA TaqMan Low Density Array (TLDA) analysis. The authors then selected a subset of differentially expressed miRNAs (schizophrenia/control pooled plasma) with more than a twofold difference by sRNA-seq and a 10-fold difference by TLDA that were consistent by direction on each platform. These criteria yielded eight miRNAs—miR-122, miR-130a, miR-130b, miR-193a-3p, miR-193b, miR-502-3p, miR-652, and miR-886-5p—which were then analyzed separately in the 300 individual samples of the discovery cohort by targeted quantitative polymerase chain reaction (qPCR) analysis.
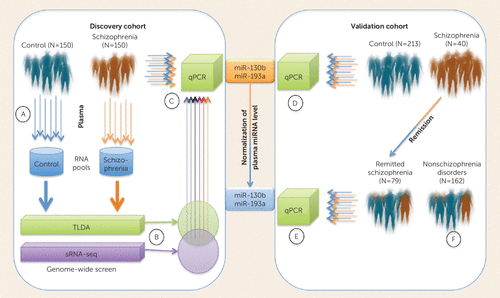
FIGURE 1. Scheme for Schizophrenia-Associated Plasma miRNA Discovery and Validation Workflow Employed by Wei et al.a
a qPCR=quantitative polymerase chain reaction; TLDA=TaqMan Low Density Array; sRNA-seq=small RNA sequencing. The plasma samples from the discovery group of 150 case subjects and 150 control subjects were pooled and extracted (A). RNA from each pool was then subjected to genome-wide miRNA analysis using TLDA and small RNA sequencing (B). miRNAs in common between the two techniques were then reanalyzed in individual qPCR reactions (C). The two miRNA molecules that remained significant through individual sample reactions were then validated in a large cohort using qPCR (D). Using the same qPCR, the authors then analyzed a subgroup of participants who were in remission (>6 months) after treatment with atypical antipsychotics (E). Samples designated nonschizophrenia psychiatric disorders were also analyzed and had levels similar to those of the control group (F).
Under these conditions, allowing the full heterogeneity of the discovery set to be accounted for, only two molecules, miR-130b and miR-193a-3p, remained significantly altered in the schizophrenia group compared with the control group. Fortunately, the disease-associated up-regulation of these molecules was robust and was supported in a large validation sample consisting of another 400 case subjects and 213 control subjects. The elevated levels of these two miRNAs were shown to be relatively specific to schizophrenia, as no significant difference was observed between 400 control subjects and 162 subjects with nonschizophrenia psychiatric disorders, including major depression, anxiety, bipolar disorder, and other atypical psychiatric diseases. The 400 participants in the schizophrenia group were followed up after 12 months of regular treatment with the atypical antipsychotics risperidone and aripiprazole. Interestingly, the plasma levels of miR-130b and miR-193a-3p appeared to normalize with respect to the control group in patients who were in remission (>6 months, N=79/107) according to the amelioration of their symptom severity on the Positive and Negative Syndrome Scale as defined by the Remission in Schizophrenia Working Group (5). In nonresponsive individuals (N=28) who exhibited acute-phase symptoms, plasma levels of these molecules remained relatively high, suggesting that they may be indicative of psychotic illness.
While this is not the first investigation of plasma miRNA levels in schizophrenia, the scale of this project is impressive, and the results provide some promise that circulating small RNA levels could be informative about the disorder and possibly other psychiatric syndromes. Ideally, it would have been desirable to have complete genome-wide screens of each individual sample rather than pooled samples. This would have been expensive and perhaps difficult if only small amounts of plasma were available; however, it may have revealed more molecules with the potential to establish complex molecular signatures with greater capacity for discrimination in heterogeneous populations. The authors concede that this is a limitation of the study and potentially responsible for some of the discrepancy between the two analytical approaches used in the primary screening of pooled sample groups. It may also be responsible for the high attrition rate between molecules selected from pooled analysis and the qPCR. To further validate the application of miR-130b and miR-193a-3p, follow-up studies should also endeavor to determine the sensitivity and specificity of this pattern of altered miRNA expression through receiver-operating characteristic curve analysis.
Where Do These Molecules Come From and What is Their Function?
miRNAs are an intriguing class of RNA molecule with enormous potential as biomarkers. Contrary to expectation, they seem to be able to survive in extracellular fluids largely owing to their association with RNA-binding proteins derived from the RNA-induced silencing complex (RISC), particularly Argonaute2 (Ago2) (6). They are also selectively enriched in membrane-encapsulated extracellular vesicles known as microparticles and exosomes (7). These are actively secreted from a variety of tissues and provide a protective microenvironment in fluids rich in RNA-hostile nucleases. As extracellular miRNAs can emerge from anywhere in the body, particularly immunocytes, neurons, and glia, they potentially report on the status of remote systems (8–10). The authors suggest that these plasma miRNAs could be derived from abnormally activated lymphocytes in schizophrenia as a consequence of disease-associated genetic variation at loci involved in the B-cell lineage development (1). In support of this hypothesis, my group observed a schizophrenia-associated reduction of miR-193b (altered in the discovery set of the Wei et al. study) and miR-130b-5p (passenger strand of miR-130b) (11), suggesting that intracellular levels of these miRNAs may be depleted in lymphocytes through secretion, which leads to elevated extracellular and circulating levels.
miRNAs have emerged as important regulators of brain development and function, and they appear to be involved in neurological and neuropsychiatric disorders (12). Several groups have observed neuropathological changes in the expression of miRNA in postmortem studies of schizophrenia (13). In accordance with both the active and remission levels of miR-193a-3p in the Wei et al. study, my laboratory observed this molecule’s up-regulation in the dorsolateral prefrontal cortex in schizophrenia (14) and down-regulation in the mouse brain after treatment with the atypical antipsychotic olanzapine (15). Regardless of their origin, these molecules have the potential to influence neural function and alter brain physiology, particularly if they are encapsulated in exosomes that can be taken up in remote cells and induce changes in gene expression (16). It is conceivable that systemic inflammatory processes may even induce or exacerbate neuroinflammation through the exosomal transfer of inflammatory molecules including miRNAs to the CNS (17). Wei et al. highlight several target genes of these miRNAs with potential significance to schizophrenia and brain function, suggesting that elevated levels may have relevant neurobehavioral implications.
Neuropsychiatric syndromes such as schizophrenia consist of complex clusters of behavioral phenotypes with relatively diffuse and inconsistent neuropathology. Diagnosis can therefore be a slow, iterative process requiring highly trained clinicians. Informative molecular biomarkers, including circulating miRNAs, could find a multiplicity of applications in both clinical and research environments by providing objective diagnostic and prognostic information. miRNAs have several advantages, including their proximity to the genetic basis of complex traits, in some cases directly linked, such as the genome-wide-associated variant MIR137 (1). They are also involved in epigenetic programming that arises through the culmination of environmental interaction. As functional entities with direct and immediate influence in cell biology, they may reflect the status of biological systems better than coding transcripts, which are subject to posttranscriptional and posttranslational regulation. Finally, miRNAs are surprisingly stable in extracellular fluids and are therefore relatively accessible molecules that can report on the prevailing “state” of deep tissue changes. These molecular signatures, including elevation of miR-130b and miR-193a-3p, may ultimately provide a basis for subtyping patients and predict therapeutic strategies that maximize efficacy and minimize adverse reactions.
1
2 : Projecting the performance of risk prediction based on polygenic analyses of genome-wide association studies. Nat Genet 2013; 45:400–405Crossref, Medline, Google Scholar
3 : Reliable biomarkers and predictors of schizophrenia and its treatment. Psychiatr Clin North Am 2012; 35:645–659Crossref, Medline, Google Scholar
4 : Detection of circulating miRNA levels in schizophrenia. Am J Psychiatry 2015; 172:1141–1147Link, Google Scholar
5 : Remission in schizophrenia: proposed criteria and rationale for consensus. Am J Psychiatry 2005; 162:441–449Link, Google Scholar
6 : Argonaute2 complexes carry a population of circulating microRNAs independent of vesicles in human plasma. Proc Natl Acad Sci USA 2011; 108:5003–5008Crossref, Medline, Google Scholar
7 : Classification, functions, and clinical relevance of extracellular vesicles. Pharmacol Rev 2012; 64:676–705Crossref, Medline, Google Scholar
8 : Activity-associated miRNA are packaged in Map1b-enriched exosomes released from depolarized neurons. Nucleic Acids Res 2014; 42:9195–9208Crossref, Medline, Google Scholar
9 : Exosome release by primary B cells. Crit Rev Immunol 2009; 29:203–217Crossref, Medline, Google Scholar
10 : Emerging roles of exosomes in neuron-glia communication. Front Physiol 2012; 3:119Crossref, Medline, Google Scholar
11 : Imprinted DLK1-DIO3 region of 14q32 defines a schizophrenia-associated miRNA signature in peripheral blood mononuclear cells. Mol Psychiatry 2012; 17:827–840Crossref, Medline, Google Scholar
12 : MicroRNA and posttranscriptional dysregulation in psychiatry. Biol Psychiatry 2015; 78:231–239Crossref, Medline, Google Scholar
13 : MicroRNA dysregulation in schizophrenia. Neurobiol Dis 2012; 46:263–271Crossref, Medline, Google Scholar
14 : Upregulation of dicer and microRNA expression in the dorsolateral prefrontal cortex Brodmann area 46 in schizophrenia. Biol Psychiatry 2011; 69:180–187Crossref, Medline, Google Scholar
15 : Gene-microRNA interactions associated with antipsychotic mechanisms and the metabolic side effects of olanzapine. Psychopharmacology (Berl) 2013; 227:67–78Crossref, Medline, Google Scholar
16 : Secreted monocytic miR-150 enhances targeted endothelial cell migration. Mol Cell 2010; 39:133–144Crossref, Medline, Google Scholar
17 : Exosomes as mediators of neuroinflammation. J Neuroinflammation 2014; 11:68Crossref, Medline, Google Scholar