Effects of Depression, Anxiety, Comorbidity, and Antidepressants on Resting-State Heart Rate and Its Variability: An ELSA-Brasil Cohort Baseline Study
Abstract
Objective:
Increases in resting-state heart rate and decreases in its variability are associated with substantial morbidity and mortality, yet contradictory findings have been reported for the effects of the mood and anxiety disorders and of antidepressants. The authors investigated heart rate and heart rate variability in a large cohort from Brazil, using propensity score weighting, a relatively novel method, to control for numerous potential confounders.
Method:
A total of 15,105 participants were recruited in the Brazilian Longitudinal Study of Adult Health. Mood and anxiety disorders were ascertained using the Portuguese version of the Clinical Interview Schedule–Revised. Heart rate and its variability were extracted from 10-minute resting-state electrocardiograms. Regressions weighted by propensity scores were carried out to compare participants with and without depressive or anxiety disorders, as well as users and non-users of antidepressants, on heart rate and heart rate variability.
Results:
Use of antidepressants was associated with increases in heart rate and decreases in its variability. Effects were most pronounced for the tricyclic antidepressants (Cohen’s d, 0.72–0.81), followed by serotonin and norepinephrine reuptake inhibitors (Cohen’s d, 0.42–0.95) and other antidepressants (Cohen’s d, 0.37–0.40), relative to participants not on antidepressants. Only participants with generalized anxiety disorder showed robust, though small, increases in heart rate and decreases in its variability after propensity score weighting.
Conclusions:
The findings may, in part, underpin epidemiological findings of increased risk for cardiovascular morbidity and mortality. Many factors that have an adverse impact on cardiac activity were controlled for in this study, highlighting the importance of cardiovascular risk reduction strategies. Further study is needed to examine whether, how, and when such effects contribute to morbidity and mortality.
Heart rate and its variability are determined by a variety of physiological factors, the most prominent of which is the autonomic system. In the resting state, the heart is under tonic inhibitory control by the parasympathetic (vagal) nervous system (1). Although the parasympathetic and sympathetic nervous systems are typically conceptualized as two opposing components, recent thinking suggests that vagal activity may be withdrawn without a corresponding increase in sympathetic nervous system activity (2). This metabolically conservative response during challenge may mirror autonomic dysregulation in psychiatric illness during the resting state. It is in this regard that we refer to resting-state heart rate and its variability as surrogate markers of vagally mediated cardiac activity. It is important to acknowledge, however, that measures of heart rate variability and the high-frequency component in particular are more pure indicators of vagal activity than heart rate (3), which may include sympathetic input. In this study, we examined the impact of depression, anxiety, comorbidity, and antidepressants on resting-state heart rate and its variability, measures we refer to here as “vagal activity” for brevity.
Vagal activity plays an important role in mental and physical well-being (see reference 4 for a review). Increases in vagal activity are associated with positive emotions, social connectedness, and longevity. By contrast, decreases in vagal activity are associated with depression, anxiety, cardiovascular disease, and mortality, findings that may be attributable to the downstream effects of a poorly functioning cholinergic anti-inflammatory reflex. Other mechanisms, including chronically elevated activity in the sympathetic nervous system, are also involved in the pathway from emotion to morbidity. However, we suggest that vagal activity may provide a unique structural link from day-to-day emotion experience to morbidity and mortality from a host of conditions. Within-subject phasic changes in vagal activity reflect a normal response to environmental challenge, while between-subject resting-state tonic differences in vagal activity predict future mortality. In this regard, an early seminal study on the effects of decreased heart rate variability following acute myocardial infarction (5), as measured by the standard deviation of all normal R-R intervals in 24-hour continuous ECG recordings from Holter monitors, reported a fivefold increase in relative risk of mortality.
We and others have demonstrated that vagal activity is reduced in the mood and anxiety disorders (see reference 4 for a review). In one of the first studies on this topic more than two decades ago (6), depressed patients with coronary artery disease were found to have a higher heart rate relative to nondepressed patients with coronary artery disease, independent of age, smoking, and beta-blocker medication. Depressed patients also had reduced heart rate variability, although this association fell short of statistical significance. This study was also based on data extracted from 24-hour ambulatory ECG recordings. Reliable and valid measures of heart rate and its variability may also be obtained from shorter recordings under standardized and controlled conditions (7). Using short-term ECG recordings in another early study (8), we reported that patients with generalized anxiety disorder displayed lower high-frequency heart rate variability. High-frequency heart rate variability, in particular, is mediated solely by changing levels of vagal activity (3). We concluded that generalized anxiety disorder and its cardinal feature (worry) are associated with reduced vagal activity. More recently, we demonstrated that patients with comorbid major depressive disorder (which we refer to here as “depression” for brevity) and generalized anxiety disorder (9; see reference 4 for review) display the most robust reductions in resting-state vagal activity. Our explanation for these findings is that chronic worry and hypervigilance to threat may underpin chronic withdrawal of vagal activity that may subsequently lead to increased morbidity and mortality.
Recent debate has focused on whether mental disorders such as depression and anxiety are associated with reductions in vagal activity (see reference 4 for review) or whether these findings are driven by antidepressant medications (10, 11). However, studies have seldom used appropriate measures to adequately control for the effects of these variables on vagal activity. Inappropriate control of confounding factors may lead to a phenomenon known as the “reversal paradox,” in which “the association between two variables may be reversed, diminished, or enhanced when another variable is statistically controlled” (12). Here we control for confounding factors through propensity score weighting using a generalized boosted modeling technique (13).
This relatively novel method has several advantages over traditional regression-based techniques, including 1) application of flexible machine-learning algorithms yielding well-calibrated estimates; 2) capture of complex and nonlinear relationships between groups and covariates without overfitting; 3) reduced bias by estimating the propensity score without reference to the outcome variable; and 4) the opportunity to analyze observational data to mimic the advantages of a randomized controlled trial design. This methodological approach provides an alternative to multiple regression that is more interpretable and less prone to violation of model assumptions (14). We apply this technique on a large sample of participants recruited for the Brazilian Longitudinal Study of Adult Health (ELSA-Brasil) (15).
ELSA-Brasil is the first large multicenter cohort study of adult health conducted in Brazil. It is an ongoing cohort study designed to investigate the relationship between cardiovascular diseases and diabetes, as well as their social determinants and risk factors. In this regard, vagal activity is an important consideration (4), and cross-sectional analysis of the ELSA-Brasil cohort at baseline will provide an important foundation for future longitudinal analyses of data from this cohort. Consistent with our previous studies with smaller samples (9, 16, 17), we hypothesized that individuals diagnosed with mood and anxiety disorders would exhibit reductions in vagal activity, and that use of antidepressants would be associated with further reductions, a finding that would be most apparent in users of tricyclic antidepressants.
Method
Participants
A total of 15,105 civil servants ages 35 to 74 were recruited for the first wave of ELSA-Brasil (15) between August 2008 and December 2010 at six sites in Brazil. Exclusion criteria were current or recent pregnancy (within 4 months of first interview), intention to quit working at the institution in the near future, severe cognitive or communication impairment, and if retired, residence outside of a study center’s metropolitan area. The study design and sampling procedures of ELSA-Brasil have been reported previously (15; see also Revista de Saúde Pública, volume 47, supplement 2, which is dedicated to methodological aspects of the ELSA-Brasil study and includes articles in English).
Procedures
Data were collected from participants in two phases: the first, lasting approximately 1 hour, included collection of informed consent and an initial interview at the participant’s job site; the second, lasting approximately 6 hours, was conducted in a study clinic and involved additional interviews, clinical examinations, collection of blood and urine samples, and a comprehensive set of examinations and measurements, including ECG. Participants were asked to abstain from caffeine, alcohol, and physical activity 12 hours prior to ECG assessment. These procedures are described in more detail below (see also reference 15).
Psychiatric Evaluation
Mental disorders were ascertained by trained interviewers using the Portuguese version (18) of the Clinical Interview Schedule–Revised (19). This is a structured interview used for diagnosis of current common nonpsychotic psychiatric conditions in the community. The following categories were determined according to ICD-10: depression (F32.xx), agoraphobia (F40.0), social phobia (F40.1), specific phobia (F40.2), generalized anxiety disorder (F41.1), panic disorder (F41.0), and social anxiety disorder (F42). These diagnostic groupings were then reduced to five categories: depression, generalized anxiety disorder, all phobias, panic disorder, and a comorbid group comprising participants with depression and any anxiety disorder.
ECG Assessment
A 10-minute resting-state ECG recording was performed for each participant during spontaneous breathing without task demands, in the supine position. The ECG was always performed in the morning (between 8:00 a.m. and noon) in a temperature-controlled room (21–24°C) and was sampled at 250 Hz with a digital electrocardiograph (Micromed, Brazil), consistent with international standards for the measurement of heart rate variability (7). The WinCardio software program, version 4.4a, was used to generate the R-R interval series from a selected lead (D2), associated with higher R-wave amplitude. The artifact detection and spectral analytic techniques have been described previously (20). Briefly, the R-R series was automatically preprocessed to remove ectopic beats and artifact, and linear interpolation was employed to replace any removed beats. Power spectral analysis was carried out by autoregressive modeling, estimated by the Yule Walker method, using the recursive algorithm of Levinson-Durbin. Measures of heart rate and its variability, including the root mean square of successive squared differences, as well as the high-frequency components, were then extracted. The high-frequency component (0.15–0.40 Hz) was estimated and expressed in absolute units. Measures of heart rate variability were then log-transformed as a normalization strategy.
Covariates
A host of covariates that are considered to have an impact on vagal activity were used in propensity score matching. Sociodemographic information included age and sex. Behavioral and lifestyle characteristics included smoking status (past or current versus never), physical activity (measured with the International Physical Activity Questionnaire and categorized according to low activity versus moderate or high activity, as determined using scoring guidelines), and body mass index. Data on health included information on hypertension, diabetes mellitus, dyslipidemia, myocardial infarction, and coronary revascularization. Hypertension was defined as a systolic blood pressure ≥140 mmHg, a diastolic blood pressure ≥90 mmHg, or use of antihypertensive medications. Diabetes was defined as a self-reported or fasting blood glucose level ≥126 mg/dL, a 2-hour oral glucose tolerance test glucose level ≥200 mg/dL, or a glycated hemoglobin level ≥6.5%. Dyslipidemia was defined as an LDL cholesterol level ≥130 mg/dL or use of lipid-lowering medication. Blood samples were collected after a 12-hour overnight fast. Medication use for hypertension, diabetes, and lipid reduction, as well as antidepressants, was identified on the basis of pill bottle review. Analysis of the mood and anxiety disorders also included total score on the Clinical Interview Schedule–Revised to control for disorder severity.
Statistical Analysis
Statistical analysis was conducted with SPSS, version 21 (IBM, Armonk, N.Y.) and R-statistics, version 3.0.1 (www.r-project.org/). Regressions weighted by propensity scores were carried out using the “twang” and “survey” packages in the R statistical environment. Both R and the two packages are freely available, and details on how to implement procedures have been described previously (13). Two main analyses were conducted. The first involved comparison of participants with depression, phobias, panic disorder, or generalized anxiety disorder, participants with comorbid depression and any anxiety disorder, and participants without any depressive or anxiety disorders (comparison participants) on measures of vagal activity (heart rate, root mean square of successive differences, and high-frequency components). The second compared users of selective serotonin reuptake inhibitors (SSRIs), serotonin and norepinephrine reuptake inhibitors (SNRIs), tricyclic antidepressants, and other antidepressants on these same measures. Effective sample sizes were obtained after propensity score weighting; these reflect numbers of participants with similar features on covariates in each of the groups. Effective sample sizes capture the adverse impact of increased variance on precision and power (13). Sensitivity analyses were conducted using propensity score matching. While weighting involves covariance-adjusted, regression-based modeling in which propensity scores are used as a covariate, matching involves selecting comparison participants (without depressive or anxiety disorders) to match the other groups on propensity scores. Here, propensity score matching was carried out using the MatchIt package in the R statistical environment using one-to-one exact matching, which paired each participant of each disorder group (the depression, anxiety, and comorbid groups) with a participant from the comparison group based on the propensity score. SPSS was then used to conduct between-group t tests on matched samples.
Results
Participant Characteristics
Participant characteristics before and after propensity score weighting are presented in the data supplement that accompanies the online edition of this article: Table S1 presents characteristics for the mood and anxiety disorder groupings, and Table S2 does so for the antidepressant groupings.
Impact of Disorder on Vagal Activity
After propensity score weighting, effective sample sizes were as follows: comparison participants with no depressive or anxiety disorders, N=10,346; participants with depression, N=98; participants with phobias, N=121; participants with panic disorder, N=43; participants with generalized anxiety disorder, N=1,183; and participants with comorbid disorders, N=311. Average heart rate was slightly higher in the generalized anxiety disorder group relative to the comparison group (67.30 bpm compared with 66.79 bpm), although the association fell short of statistical significance and was associated with a small effect size (p=0.086, d=0.053). No other groups differed significantly from the comparison group. Sensitivity analysis using propensity score matching revealed similar nonsignificant findings for the generalized anxiety disorder group (N=1,249) relative to the comparison group (N=1,236) (67.23 bpm compared with 67.90 bpm; p=0.065, d=0.074).
Analysis of heart rate variability revealed significant effects for both measures after propensity score weighting; root mean square of successive differences was lower in the generalized anxiety disorder group relative to the comparison group (3.17 compared with 3.21; p=0.015, d=0.075), as was the high-frequency component (5.23 compared with 5.32; p=0.033, d=0.066). No other groups differed significantly from the comparison group. Again, sensitivity analysis using propensity score matching revealed similar findings for the generalized anxiety disorder group relative to the comparison group (root mean square of successive differences: 3.18 compared with 3.25; p=0.004, d=0.116; high-frequency component: 5.29 compared with 5.42; p=0.005, d=0.113).
Impact of Antidepressants on Vagal Activity
After propensity score weighting, effective sample sizes were as follows: participants not on antidepressants, N=14,069; those on SSRIs, N=356; those on SNRIs, N=52; those on tricyclic antidepressants, N=84; and those on other antidepressants, N=75. As shown in Figure 1, average heart rate relative to those not on antidepressants (mean=66.87 bpm) was higher in those on tricyclics (mean=73.94 bpm, p<0.001, d=0.721), those on other antidepressants (mean=71.00 bpm, p=0.001, d=0.380), and those on SNRIs (mean=70.55 bpm, p=0.003, d=0.420) and slightly lower among those on SSRIs (mean=65.40 bpm, p=0.003, d=0.161). In addition, all antidepressants were associated with a lower heart rate variability (root mean square of successive differences, tricyclics: mean=2.71, p<0.001, d=0.810; SNRIs: mean=2.79, p<0.001, d=0.952; other antidepressants: mean=2.95, p<0.001, d=0.402; SSRIs: mean=3.08, p<0.001, d=0.280; high-frequency component, tricyclics: mean=4.26, p<0.001, d=0.730; SNRIs: mean=4.48, p<0.001, d=0.789; other antidepressants: mean=4.82, p=0.001, d=0.367; SSRIs: mean=5.05, p<0.001, d=0.254) relative to those not on antidepressants (root mean square of successive differences: mean=3.23; high-frequency component: mean=5.36). These findings were all replicated in sensitivity analysis using propensity score matching (results not shown).
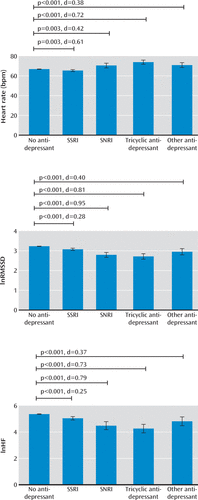
FIGURE 1. Key Findings Associated With the Impact of Antidepressants on Heart Rate and Its Variability After Propensity Score Weightinga
a lnRMSSD=log-transformed root mean square of successive difference; lnHF=log-transformed high-frequency component; SSRI=selective serotonin reuptake inhibitor; SNRI=serotonin and norepinephrine reuptake inhibitor. Error bars indicate 95% confidence intervals corresponding to the propensity score weighted regression. Antidepressant group sizes are follows: no antidepressant, N=14,069; SSRI, N=356; SNRI, N=52; tricyclic antidepressant, N=84; other antidepressant, N=75.
Discussion
The most striking effects from our study relate to the use of antidepressant medications; tricyclic antidepressants, SNRIs, and other antidepressants were associated with moderate to large adverse effects. With respect to the impact of mood and anxiety disorders—an issue that has attracted the most debate—the findings demonstrate that only generalized anxiety disorder is associated with a reduction in vagal activity after controlling for a host of clinical covariates, including biomarkers of metabolic and cardiovascular risk. The effects of generalized anxiety disorder on vagal activity were statistically significant and robust, although effect sizes were small. This is an important finding, as other researchers have recently argued that it is the antidepressant medication, rather than the disorder per se, that has an adverse impact on vagal activity (10, 11). While it is notable that participants with comorbid depression and anxiety disorders did not display reductions in vagal activity, it is important to bear in mind that many of the factors that adversely affect vagal activity were controlled for in this study.
Mood and anxiety disorders raise metabolic and cardiovascular risk (21), emphasizing the need for comprehensive cardiovascular risk reduction strategies in all patients with these disorders to minimize subsequent morbidity and mortality. In this study, we showed that only generalized anxiety disorder without comorbidity is associated with reductions in vagal activity. These findings highlight the independent adverse effects of generalized anxiety disorder without comorbidity on vagal activity. It is important to note, however, that generalized anxiety disorder and depression are linked sequentially such that each disorder increases the likelihood of developing the other over time (22). Longitudinal follow-up of our participants will provide important data to further examine the relationship between depression and generalized anxiety disorder over time and the disorders’ effects on vagal activity. In line with our previous studies on generalized anxiety disorder (8, 9), we suggest that the cardinal features of this disorder, including chronic worry and hypervigilance to threat, independently contribute to long-term reductions in vagal activity and subsequent increases in morbidity and mortality.
Previous studies of mood disorders and vagal activity have reported contradictory findings, leading to significant debate and discussion. In 2007, Rottenberg (23) reported findings from a meta-analysis indicating that depression had an adverse impact on vagal activity, a finding associated with a small to medium effect size. He also suggested that low heart rate variability may be a consequence of comorbid anxiety, rather than of depression per se. Licht et al. reported that although heart rate variability is reduced in patients with depression (24) and those with anxiety disorders (25), these reductions are driven by antidepressant medications. These findings contrasted with smaller studies that had reported adverse effects of the mood and anxiety disorders, leading to the publication of our meta-analysis in 2010 (16). We reported that heart rate variability was lower in unmedicated depressed patients relative to healthy comparison subjects, a finding associated with a large effect size when focusing on nonlinear measures of heart rate variability. Recent years have witnessed increasing calls for independent replication of published findings in science (26, 27). Consistent with this goal, we have examined the impact of mental disorders and antidepressants on vagal activity using a variety of approaches. In addition to meta-analysis, we have replicated reductions in vagal activity in mood and anxiety disorders in multiple independent cohorts of patients (9, 17, 28). In the largest independent cohort to date, we now report that after controlling for multiple confounding variables, we find that generalized anxiety disorder is robustly associated with reductions in vagal activity, although these reductions are small. It is possible that the lack of findings for depression are related to disorder heterogeneity. For example, specific depression characteristics (e.g., melancholia) may be associated with more robust effects on vagal activity.
We suggest here that the effects we observed for the tricyclics and SNRIs in particular may underpin previously reported increases in risk for cardiovascular morbidity and mortality (29, 30). There is strong evidence for a continuous increase in risk for cardiovascular and all-cause mortality in men and women with a resting heart rate above 60 bpm, regardless of history of cardiovascular disease (31). In the present study, we showed that tricyclic antidepressants were associated with an average resting heart rate of ∼74 bpm, while SNRIs and other antidepressants were associated with an average resting heart rate of approximately ∼71 bpm, compared with ∼67 bpm for those not taking antidepressants. In light of increasing concerns about the impact of antidepressant medications (10, 29, 30), we recently investigated the moderating effects of physical activity on the impact of the SSRI escitalopram on cardiac stress responses (32). We found that participants who engaged in exercise at least three times a week exhibited a more resilient vagal response to stress relative to irregular exercisers, a finding associated with a moderate effect size. We also observed that acute treatment with escitalopram had a similar impact on irregular exercisers during stress. The moderating effects of regular exercise in long-term users of antidepressants need to be examined in future research. In the present study, the SSRIs were associated with a decrease in heart rate and its variability. It is particularly interesting that these findings have been reported previously for this class of medication (10)—findings that were interpreted as both a decrease in sympathetic activity and parallel decreases in net cardiac vagal effects.
While our study has important strengths—particularly the application of propensity score weighting on a large, independent cohort—a number of limitations should also be noted. First, the reliability of short-term measurements of heart rate variability has been questioned (33). However, we have demonstrated that the protocol on which this study is based shows good reproducibility (34). Second, we acknowledge that respiration rate may influence estimates of heart rate and its variability. While we did not collect data on respiration rate, the question of whether or not respiration rate should be controlled for remains a divisive one in the field of psychophysiology. Researchers have even cautioned against experimentally controlling for respiration, as this may confound the visceral-medullary feedback system and shift respiratory parameters (2). Also, the root mean square of successive differences, unlike frequency-based measures, may actually be more robust to changes in breathing patterns (35). In the present study, key findings were obtained across both measures of heart rate variability, highlighting the robustness of findings. Finally, it should be noted that although we controlled for a variety of important covariates, there are likely other factors that we did not measure or include in our models that may have affected our findings. Thus, there remains the potential for residual confounding factors in our study.
In summary, this study provides much-needed evidence for the impact of mood and anxiety disorders, as well as antidepressants, on resting-state vagal activity after controlling for a large number of confounding factors. Future studies employing a randomized controlled design will provide further important evidence for the impact of specific antidepressants on vagal activity. Further research is also needed to determine whether certain clinical characteristics and their amelioration moderate observed findings. It is possible, for instance, that specific subtypes of depression (e.g., melancholia) or specific symptoms of a depressive episode or an anxiety disorder (e.g., somatic symptoms) have more robust effects on vagal activity. There is also a need for research to determine how and when reduced vagal activity leads to morbidity and mortality from a host of conditions.
1 : Heart rate variability, prefrontal neural function, and cognitive performance: the neurovisceral integration perspective on self-regulation, adaptation, and health. Ann Behav Med 2009; 37:141–153Crossref, Medline, Google Scholar
2 : The Polyvagal Theory: Neurophysiological Foundations of Emotions, Attachment, Communication, and Self-Regulation. New York, WW Norton, 2011Google Scholar
3 : Beat-to-beat variations of heart rate reflect modulation of cardiac autonomic outflow. Physiology (Bethesda) 1990; 5:32–37Google Scholar
4 : The relationship between mental and physical health: insights from the study of heart rate variability. Int J Psychophysiol 2013; 89:288–296Crossref, Medline, Google Scholar
5 : Decreased heart rate variability and its association with increased mortality after acute myocardial infarction. Am J Cardiol 1987; 59:256–262Crossref, Medline, Google Scholar
6 : The relationship between heart rate, heart rate variability, and depression in patients with coronary artery disease. J Psychosom Res 1988; 32:159–164Crossref, Medline, Google Scholar
7
8 : Autonomic characteristics of generalized anxiety disorder and worry. Biol Psychiatry 1996; 39:255–266Crossref, Medline, Google Scholar
9 : Depression, comorbid anxiety disorders, and heart rate variability in physically healthy, unmedicated patients: implications for cardiovascular risk. PLoS One 2012; 7:e30777Crossref, Medline, Google Scholar
10 : Longitudinal evidence for unfavorable effects of antidepressants on heart rate variability. Biol Psychiatry 2010; 68:861–868Crossref, Medline, Google Scholar
11 : Effects of antidepressants, but not psychopathology, on cardiac sympathetic control: a longitudinal study. Neuropschopharmacology, 2012, 37:2487–2495Crossref, Medline, Google Scholar
12 : Simpson’s paradox, Lord’s paradox, and suppression effects are the same phenomenon: the reversal paradox. Emerg Themes Epidemiol 2008; 5:2Crossref, Medline, Google Scholar
13 : A tutorial on propensity score estimation for multiple treatments using generalized boosted models. Stat Med 2013; 32:3388–3414Crossref, Medline, Google Scholar
14 : Assessing the effect of race bias in post-traffic stop outcomes using propensity scores. J Quant Criminol 2006; 22:1–29Crossref, Google Scholar
15 : Cohort profile: Longitudinal Study of Adult Health (ELSA-Brasil). Int J Epidemiol (Epub ahead of print, Feb 27, 2014)Google Scholar
16 : Impact of depression and antidepressant treatment on heart rate variability: a review and meta-analysis. Biol Psychiatry 2010; 67:1067–1074Crossref, Medline, Google Scholar
17 : Heart rate variability is a trait marker of major depressive disorder: evidence from the Sertraline vs Electric Current Therapy to Treat Depression Clinical Study. Int J Neuropsychopharmacol 2013; 16:1937–1949Crossref, Medline, Google Scholar
18 : Adaptação transcultural do CIS-R (Clinical Interview Schedule–Revised Version) para o português no Estudo Longitudinal de Saúde do Adulto (ELSA). Revista HCPA 2012; 31:487–490Google Scholar
19 : Measuring psychiatric disorder in the community: a standardized assessment for use by lay interviewers. Psychol Med 1992; 22:465–486Crossref, Medline, Google Scholar
20 : Spectral analysis of heart rate variability with the autoregressive method: what model order to choose? Comput Biol Med 2012; 42:164–170Crossref, Medline, Google Scholar
21 : Heartache and heartbreak: the link between depression and cardiovascular disease. Nat Rev Cardiol 2012; 9:526–539Crossref, Medline, Google Scholar
22 : Depression and generalized anxiety disorder: cumulative and sequential comorbidity in a birth cohort followed prospectively to age 32 years. Arch Gen Psychiatry 2007; 64:651–660Crossref, Medline, Google Scholar
23 : Cardiac vagal control in depression: a critical analysis. Biol Psychol 2007; 74:200–211Crossref, Medline, Google Scholar
24 : Association between major depressive disorder and heart rate variability in the Netherlands Study of Depression and Anxiety (NESDA). Arch Gen Psychiatry 2008; 65:1358–1367Crossref, Medline, Google Scholar
25 : Association between anxiety disorders and heart rate variability in the Netherlands Study of Depression and Anxiety (NESDA). Psychosom Med 2009; 71:508–518Crossref, Medline, Google Scholar
26 : Why most discovered true associations are inflated. Epidemiology 2008; 19:640–648Crossref, Medline, Google Scholar
27 : Rewarding replications: a sure and simple way to improve psychological science. Perspect Psychol Sci 2012; 7:608–614Crossref, Medline, Google Scholar
28 : Reduced heart rate variability in social anxiety disorder: associations with gender and symptom severity. PLoS ONE 2013; 8:e70468Crossref, Medline, Google Scholar
29 : Depression and risk of sudden cardiac death and coronary heart disease in women: results from the Nurses’ Health Study. J Am Coll Cardiol 2009; 53:950–958Crossref, Medline, Google Scholar
30 : Antidepressant medication use and future risk of cardiovascular disease: the Scottish Health Survey. Eur Heart J 2011; 32:437–442Crossref, Medline, Google Scholar
31 : Resting heart rate in cardiovascular disease. J Am Coll Cardiol 2007; 50:823–830Crossref, Medline, Google Scholar
32 : The impact of escitalopram on vagally mediated cardiovascular function to stress and the moderating effects of vigorous physical activity: a randomized controlled treatment study in healthy participants. Front Physiol 2013; 4:259Crossref, Medline, Google Scholar
33 : The reliability of short-term measurements of heart rate variability. Int J Cardiol 2005; 103:238–247Crossref, Medline, Google Scholar
34 : Reproducibility of heart rate variability parameters measured in healthy subjects at rest and after a postural change maneuver. Braz J Med Biol Res 2010; 43:982–988Crossref, Medline, Google Scholar
35 : Time domain, geometrical and frequency domain analysis of cardiac vagal outflow: effects of various respiratory patterns. Clin Physiol 2001; 21:365–376Crossref, Medline, Google Scholar