Prenatal and Neonatal Brain Structure and White Matter Maturation in Children at High Risk for Schizophrenia
Abstract
Objective:
Schizophrenia is a neurodevelopmental disorder associated with abnormalities of brain structure and white matter, although little is known about when these abnormalities arise. This study was conducted to identify structural brain abnormalities in the prenatal and neonatal periods associated with genetic risk for schizophrenia.
Method:
Prenatal ultrasound scans and neonatal structural magnetic resonance imaging (MRI) and diffusion tensor imaging were prospectively obtained in the offspring of mothers with schizophrenia or schizoaffective disorder (N=26) and matched comparison mothers without psychiatric illness (N=26). Comparisons were made for prenatal lateral ventricle width and head circumference, for neonatal intracranial, CSF, gray matter, white matter, and lateral ventricle volumes, and for neonatal diffusion properties of the genu and splenium of the corpus callosum and corticospinal tracts.
Results:
Relative to the matched comparison subjects, the offspring of mothers with schizophrenia did not differ in prenatal lateral ventricle width or head circumference. Overall, the high-risk neonates had nonsignificantly larger intracranial, CSF, and lateral ventricle volumes. Subgroup analysis revealed that male high-risk infants had significantly larger intracranial, CSF, total gray matter, and lateral ventricle volumes; the female high-risk neonates were similar to the female comparison subjects. There were no group differences in white matter diffusion tensor properties.
Conclusions:
Male neonates at genetic risk for schizophrenia had several larger than normal brain volumes, while females did not. To the authors' knowledge, this study provides the first evidence, in the context of its limitations, that early neonatal brain development may be abnormal in males at genetic risk for schizophrenia.
Schizophrenia has been considered a neurodevelopmental disorder for more than 20 years (1, 2). This conceptualization is based on observations that schizophrenia is associated with a variety of pre- and perinatal environmental insults to the developing brain, including infection and obstetric and birth complications (3). In addition, children who ultimately develop schizophrenia exhibit a variety of subtle developmental and neurocognitive abnormalities, including delayed motor milestones, speech problems, lower educational test scores (4), and deficits in verbal memory, attention, motor skills, and receptive language (5, 6). Finally, many of the genes that may increase risk of developing schizophrenia regulate multiple processes important in brain development (7, 8).
Schizophrenia is associated with subtle abnormalities of brain structure, including larger than normal lateral ventricles, lower cortical gray matter volumes, and lower hippocampal volumes (9). In general, these structural abnormalities are present at the first episode, suggesting that they may precede the onset of clinical symptoms (10). More recent diffusion tensor imaging studies also indicate that abnormalities of white matter are present in schizophrenia (11) and that they appear to be present at the first episode as well (12).
Structural brain abnormalities have also been observed in the unaffected relatives of individuals with schizophrenia. Unaffected co-twins exhibit cortical structure abnormalities that are similar to, but less severe than, those of the affected twins. These abnormalities include low brain volume, larger than normal lateral ventricles (13), and low gray matter volumes in the prefrontal cortex (14). Similar studies in unaffected siblings also suggest a structural endophenotype of low cortical gray matter volume associated with genetic risk (15–18), although this is not a consistent finding (19, 20). Adolescents and young adults at genetic risk for schizophrenia also have evidence of low prefrontal lobe volume (21) and low gray matter density in the cingulate and the temporal and right frontal lobes (22), as well as abnormalities of white matter as shown by diffusion tensor imaging (23).
There is evidence of subtle brain structure endophenotypes associated with genetic risk for schizophrenia in adults, although it is not clear when in the course of development these endophenotypes arise. Studies of the unaffected siblings of children with early-onset schizophrenia do show smaller than normal cortical gray matter that tends to normalize in adolescence (24), suggesting that endophenotypes of genetic risk are present in childhood. Understanding of the timing of structural abnormalities would allow a better identification of neurodevelopmental processes that contribute to risk for schizophrenia. To learn more about the timing in individuals with high genetic risk, we prospectively studied prenatal brain structure with ultrasound and neonatal brain structure with magnetic resonance imaging (MRI), including diffusion tensor imaging, in the children of mothers with schizophrenia and matched comparison subjects. We hypothesized that at-risk infants would have larger lateral ventricle volumes, lower cortical gray matter volumes, and evidence of dysmaturation of major white matter tracts in relation to the comparison subjects.
Method
Participants
This study was approved by the institutional review board of the University of North Carolina School of Medicine. Women with schizophrenia or schizoaffective disorder who were pregnant were recruited from inpatient psychiatric units and outpatient clinics in central North Carolina. Subjects were asked by their treating psychiatrist or social worker if they were interested in the study, and if they were, a study coordinator met with the patient and told her more about the study. Active substance abuse was an exclusion criterion for both the patients and comparison subjects. The matched comparison subjects were selected from a companion study of normal brain development; for this study, pregnant women without psychiatric illness, major medical illness, pregnancy complications, or abnormality shown by prenatal ultrasound were recruited by flyer from the Prenatal Diagnostic Clinic at University of North Carolina Hospitals. Mothers with schizophrenia underwent a Structured Clinical Interview for DSM-IV Axis I Disorders (SCID), and past psychiatric records were obtained; a final consensus diagnosis was assigned. Potential comparison mothers were screened for psychiatric illness by using a modified SCID. Comparison infants were matched on gender, age in terms of time since last menstrual period at birth, age since the last menstrual period at MRI, and maternal age.
Prenatal Ultrasound
Ultrasound scans obtained at approximately 22 and 32 weeks after the last menstrual period were performed by trained study ultrasonographers on an ATL 5000 (Philips Medical Systems, Andover, Mass.) or Voluson Expert (GE Healthcare, Waukesha, Wis.). Head circumference was measured in a horizontal plane by using standard landmarks; the ventricle width was determined at the atrium of the lateral ventricle at the tail of the choroid, with measurement from inner wall to inner wall (25). The width of the ventricle farthest from the transducer was measured twice and averaged for each visit.
MR Image Acquisition
Images were acquired on a Siemens head-only 3-T scanner (Allegra, Siemens Medical Solutions, Erlangen, Germany). Neonates were scanned unsedated during natural sleep; they were fed prior to scanning, swaddled, and fitted with ear protection, and their heads were secured in a vacuum-fixation device. A physician or nurse was present during each scan; a pulse oximeter was used to monitor heart rate and oxygen saturation. In the initial phase of the study, T1-weighted structural pulse sequences were obtained for seven infants (four in the high-risk group, three in the comparison group) with a three-dimensional T1-weighted fast low-angle shot sequence (TR=15 msec, TE=7 msec, flip angle=25°); then a three-dimensional magnetization prepared rapid gradient echo sequence was used for 57 neonates (TR=1,820 msec, TI=400 msec, TE=4.38 msec, flip angle=7°). Proton density and T2-weighted images were obtained with a turbo spin echo sequence (TR=6,200 msec, TE1=20 msec, TE2=119 msec, flip angle=150°). The spatial resolution that was used provided a 1×1×1-mm voxel for the T1-weighted images and a 1.25×1.25×1.50-mm voxel with a 0.5-mm interslice gap for the proton density and T2-weighted images. For one neonate in the high-risk group early in the study, a lower-resolution T2 sequence was used (turbo spin echo sequence: TR=7,000 msec, TE1=18 msec, TE2=108 msec, flip angle=150°) with a 1×1×3-mm voxel size and a 0.9-mm interslice gap.
A single shot echo planar spin echo diffusion tensor imaging sequence was used with the following variables: TR=5,200 msec, TE=73 msec, slice thickness=2 mm, in-plane resolution=2×2 mm2, and 45 slices. Seven images were acquired for each slice, one without diffusion gradient (b=0) and the remaining six with b=1,000 sec/mm2 and diffusion gradients along {1/√2, 0, 1/√2}, {−1/√2, 0, 1/√2}, {0, 1/√2, 1/√2}, {0, 1/√2, −1/√2}, {1/√2, 1/√2, 0}, {−1/√2, 1/√2, 0}, separately. In order to improve the signal-to-noise ratio for the diffusion tensor images, five separate sets of images with two averages in each set were acquired. This approach shortens data acquisition time (1.18 minutes/set) and minimizes motion artifacts.
Segmentation and Lobe Parcellation Analysis
Brain tissue was automatically classified as gray matter, unmye-linated white matter, myelinated white matter, and CSF by using an atlas-moderated iterative expectation maximization segmentation algorithm based on both T1 and T2 scans as previously described (26) (Figure 1). Five subjects in the high-risk group had a T2 scan only; these segmentations were compared to the results derived from the T2 scan of the matched comparison subjects. Overall results were similar for all analyses when the T2-only subjects were excluded. Parcellation of the cortex into anatomical regions (frontal, prefrontal, parietal, and occipital) was achieved by nonlinear warping of a parcellation atlas template as previously described (26). Left and right hemispheres were subdivided into four regions along the anterior-posterior axis (prefrontal, frontal, parietal, occipital) and into infra- and supratentorial regions (Figure 1). The cerebellum, brainstem, and combined sets of subcortical structures are represented separately.
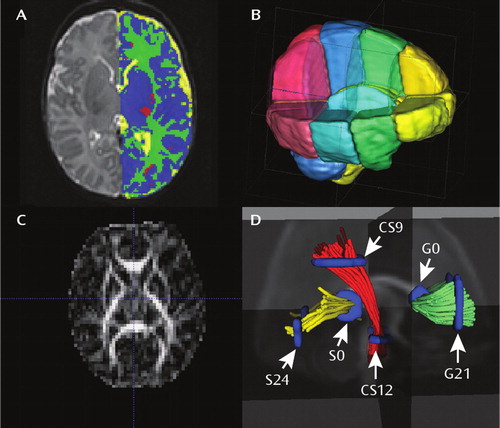
FIGURE 1. Representative MRI Images and Image Analysis of Brain Structure in Infants at High Genetic Risk for Schizophreniaa
a Part A is a T2-weighted image with tissue segmented into CSF (yellow), gray matter (blue), unmyelinated white matter (green), and myelinated white matter (red). Part B displays the regional parcellation; see text for details. Part C is a fractional anisotropy image. Part D displays the white matter tractography involving the genu of the corpus callosum (G), splenium of the corpus callosum (S), and corticospinal tract (CS).
The volume of the lateral ventricles was segmented with a user-supervised, highly automated level-set evolution tool, itkSNAP (27). Left and right lateral ventricles were separated by a three-dimensional cutting tool. Intracranial volume was defined as the sum of the automatic full-brain segmentation results for gray matter, white matter, and CSF (ventricles and subarachnoid space) volumes.
Diffusion Tensor Analysis
Each individual directional gradient image was screened off-line for motion artifacts by using an automatic diffusion tensor analysis quality control tool (DTIchecker) that calculates an average of the five repeated sequences after correction for motion and removal of outliers (28). Quantitative analysis of fiber tracts was accomplished by using a set of tools for computation of fractional anisotropy and mean diffusivity maps, quantitative tractography, fiber clustering, and parametrization as previously described (28). Tracts are initialized by drawing source and target regions of interest on fractional anisotropy images. The fiber tracking tool (FiberViewer; software download at http://www.ia.unc.edu/dev) reads the set of diffusion image channels, calculates the tensor field, reads the region of interest image, and performs the tracking. The resulting sets of streamlines are stored as list of polylines, which carry the full tensor information at each location. Cross-sectional regions of interest were defined in the left (21 mm) and right (−21 mm) cortical and central regions of the genu of the corpus callosum, in the left (24 mm) and right (−24 mm) cortical and central (0 mm) regions of the splenium of the corpus callosum, and in cortical (9 mm) and central (−12) regions of the left and right corticospinal tracts as previously described (28) (Figure 1).
Statistical Analysis
For baseline categorical variables, frequency distributions were calculated for categorical variables, and means and standard deviations were calculated for continuous variables. Differences between groups at baseline for continuous variables were assessed by means of t tests.
For examination of differences between the risk groups (high risk versus comparison) in the measurements of the regions of interest at the second trimester and third trimester, we used mixed models. We fit separate models for the second and third trimesters because many of the subjects were measured at only one of the time points. We report least squares means and standard errors along with a contrast examining the difference between the comparison and high-risk groups on these least squares means.
For a given region of interest at a given time point, the model included the block as a random effect and risk group as a fixed effect. Differences in least squares means were assessed through contrasts. The denominator value for degrees of freedom was calculated by using the Kenward and Roger method.
Results
Forty-seven women with psychosis were enrolled between May 2003 and December 2008. Four were excluded for having diagnoses other than schizophrenia and schizoaffective disorder, nine were lost to follow-up before the neonatal MRI, three refused the neonatal MRI, one experienced fetal demise, and four did not complete the MRI scan. Thus, the final study group included 26 women with schizophrenia or schizoaffective disorder and 26 matched comparison subjects. Diffusion tensor imaging scans were obtained for 19 of the women with schizophrenia or schizoaffective disorder. Second- and third-trimester ultrasounds were obtained for 11 and 16, respectively. Among the ill women, there was one set of twins and three sets of siblings. Analyses were run with and without these related high-risk subjects; the findings were not influenced by inclusion or exclusion of these subjects.
Table 1 summarizes the sociodemographic variables for the comparison and high-risk groups. There was a significant difference in maternal education between the groups; otherwise the groups were comparable. Table 2 summarizes the medication and substance exposure, as well as maternal medical problems and obstetric and perinatal complications. Twenty-three of the 26 high-risk infants had prenatal exposure to an antipsychotic; no comparison infants had antipsychotic exposure. The high-risk infants were also more likely to have been exposed to antidepressants and cigarette smoking during pregnancy. MRI scans showed that two high-risk and six comparison infants had small intracranial hemorrhages, which are common in the neonatal period and probably related to the birth process (29).
Characteristic | Comparison Infants | High-Risk Infants | ||
---|---|---|---|---|
N | % | N | % | |
Gender | ||||
Male | 12 | 46.2 | 12 | 46.2 |
Female | 14 | 53.8 | 14 | 53.8 |
Ethnicity | ||||
Caucasian | 17 | 65.4 | 12 | 46.2 |
African American | 9 | 34.6 | 13 | 50.0 |
Other | 0 | 0.0 | 1 | 3.8 |
Mean | SD | Mean | SD | |
Maternal age (years) | 27.9 | 4.5 | 29.0 | 4.0 |
Infant age (weeks since mother's last menstrual period) | ||||
At ultrasound 1 (approximately 22 weeks after last menstrual period) | 22.0 | 1.1 | 22.3 | 3.0 |
At ultrasound 2 (approximately 32 weeks after last menstrual period) | 32.0 | 1.1 | 32.4 | 0.8 |
At birth | 38.5 | 2.3 | 38.1 | 3.1 |
At MRI | 42.6 | 2.4 | 43.2 | 3.3 |
Birth weight (g) | 3112.2 | 596.3 | 3179.1 | 916.4 |
Maternal education (years)a | 14.2 | 2.7 | 10.0 | 3.6 |
TABLE 1. Demographic Characteristics of Infants at High Genetic Risk for Schizophrenia and Comparison Infants
Pre- or Perinatal Condition | Number of Comparison Infants (N=26) | Number of High-Risk Infants (N=26) | Fisher's Exact Test (p) |
---|---|---|---|
Maternal medical problems | |||
Gestational diabetes | 2 | 6a | 0.25 |
Asthma | 2 | 2 | 1.00 |
Seizure disorder | 0 | 2 | 0.40 |
Hypertension | 1 | 2 | 1.00 |
Hypothyroidism | 1 | 1 | 1.00 |
Sickle cell trait | 1 | 0 | 1.00 |
Infectionb | 10 | 17 | 0.10 |
Medication exposure | |||
Antipsychoticc | 0 | 23 | <0.0001 |
Antidepressantd | 0 | 8 | 0.005 |
Lithium | 0 | 2 | 0.50 |
Other medication | 16 | 18 | 0.78 |
Substance exposure | |||
Tobacco smoking | 1 | 12 | 0.0001 |
Alcohol | 0 | 3 | 0.24 |
Marijuana | 0 | 4 | 0.14 |
Caesarean section | 7 | 12 | 0.25 |
Premature birth (<37 weeks) | 8 | 7 | 1.00 |
Stay in neonatal intensive care unit | 2 | 6 | 0.25 |
TABLE 2. Pre- and Perinatal Conditions of Infants at High Genetic Risk for Schizophrenia and Comparison Infants
Prenatal Ultrasound
Ultrasound recordings were obtained for 11 matched pairs (five male and six female infants per group) in the second trimester. As shown by F tests for difference, there were no significant differences between the high-risk and comparison infants in either lateral ventricle width (least squares mean=0.55 [SE=0.04] versus 0.49 [SE=0.04] cm, p=0.23) or head circumference (least squares mean=20.16 [SE=0.78] versus 19.82 [SE=0.78] cm, p=0.77). Ultrasound recordings were obtained for 16 matched pairs (six male and 10 female infants per group) in the third trimester. There were no significant differences between the high-risk and comparison infants in either lateral ventricle width (least squares mean=0.46 [SE=0.04] versus 0.50 [SE=0.04] cm, p=0.56), although the high-risk infants had a nonsignificantly larger head circumference (least squares mean=30.08 [SE=0.36] versus 29.20 [SE=0.36] cm, p=0.10).
Structural MRI
There were no significant group differences in the intracranial, lateral ventricle, and cerebellum volumes or in any tissue volume (Table 3). The genetically high-risk infants did have larger intracranial, CSF, and lateral ventricle volumes, although these differences did not reach statistical significance. Controlling for intracranial volume did not reveal any group differences for any analysis. There were no group differences for gray or white matter volume in any cortical region (online data supplement).
Tissue Type | Volume (mm3) | % Difference | F Test for Difference | |||||
---|---|---|---|---|---|---|---|---|
Comparison Infants (N=26) | High-Risk Infants (N=26) | |||||||
Least Squares Mean | SE | Least Squares Mean | SE | F | df | p | ||
Intracranial | 479,305 | 13,525 | 499,603 | 13,525 | 4.2 | 2.50 | 1, 25 | 0.13 |
Total gray matter | 249,755 | 7,160 | 256,975 | 7,160 | 2.9 | 1.60 | 1, 25 | 0.22 |
Total unmyelinated white matter | 160,361 | 5,016 | 168,113 | 5,016 | 4.8 | 1.74 | 1, 25 | 0.20 |
Total myelinated white matter | 9,460 | 881 | 9,495 | 881 | 0.4 | 0.00 | 1, 25 | 0.98 |
Total CSF | 59,728 | 2,856 | 65,019 | 2,856 | 8.9 | 3.45 | 1, 25 | 0.08 |
Lateral ventricle | 4,175 | 319 | 4,644 | 319 | 11.2 | 2.14 | 1, 25 | 0.16 |
Cortical gray matter | 206,157 | 6,103 | 212,494 | 6,103 | 3.1 | 1.52 | 1, 25 | 0.23 |
Cortical white matter | 154,319 | 3,894 | 157,185 | 3,894 | 1.9 | 0.56 | 1, 25 | 0.47 |
Cerebellum | 25,508 | 922 | 25,811 | 922 | 1.2 | 0.22 | 1, 25 | 0.65 |
TABLE 3. Brain Volumes of Infants at High Genetic Risk for Schizophrenia and Comparison Infantsa
In a secondary analysis, we explored volume differences by gender. The male high-risk infants had significantly larger intracranial volumes than the male comparison infants, as well as significantly larger total gray matter, total CSF, cortical gray matter, and lateral ventricle volumes (Table 4). These differences were not significant when adjusted for intracranial volume (online data supplement). There were no group differences for intracranial volume or any other tissue volume in the female infants. We also explored the effect of maternal smoking on brain volumes in the high-risk group; there were no significant differences in any brain volume between exposed and nonexposed neonates (data not shown).
Gender and Tissue Type | Volume (mm3) | % Difference | F Test for Difference | |||||
---|---|---|---|---|---|---|---|---|
Comparison Infants | High-Risk Infants | |||||||
Least Squares Mean | SE | Least Squares Mean | SE | F | df | p | ||
Male (N=24) | ||||||||
Intracranial | 497,216 | 19,111 | 533,850 | 19,111 | 7.4 | 5.88 | 1, 11 | 0.04 |
Total gray matter | 262,836 | 10,726 | 276,743 | 10,726 | 5.3 | 6.38 | 1, 11 | 0.03 |
Total unmyelinated white matter | 162,379 | 8,294 | 178,051 | 8,294 | 9.7 | 2.65 | 1, 11 | 0.14 |
Total myelinated white matter | 9,342 | 1,288 | 8,687 | 1,288 | −7.0 | 0.22 | 1, 11 | 0.65 |
Total CSF | 62,658 | 3,438 | 70,368 | 3,438 | 12.3 | 5.51 | 1, 11 | 0.04 |
Lateral ventricle | 4,305 | 452 | 5,219 | 452 | 21.2 | 5.11 | 1, 11 | 0.05 |
Cortical gray matter | 216,741 | 9,283 | 229,592 | 9,283 | 5.9 | 5.55 | 1, 11 | 0.04 |
Cortical white matter | 160,887 | 5,193 | 166,817 | 5,193 | 3.7 | 1.62 | 1, 11 | 0.23 |
Cerebellum | 27,122 | 1,560 | 27,897 | 1,560 | 2.9 | 0.54 | 1, 11 | 0.48 |
Female (N=28) | ||||||||
Intracranial | 463,953 | 17,233 | 470,249 | 17,233 | 1.4 | 0.10 | 1, 13 | 0.76 |
Total gray matter | 238,544 | 8,079 | 240,032 | 8,079 | 0.6 | 0.03 | 1, 13 | 0.88 |
Total unmyelinated white matter | 158,632 | 5,801 | 159,595 | 5,801 | 0.6 | 0.02 | 1, 13 | 0.90 |
Total myelinated white matter | 9,561 | 1,235 | 10,188 | 1,235 | 6.6 | 0.18 | 1, 13 | 0.69 |
Total CSF | 57,216 | 4,276 | 60,435 | 4,276 | 5.6 | 0.51 | 1, 13 | 0.49 |
Lateral ventricle | 4,063 | 439 | 4,151 | 439 | 2.2 | 0.03 | 1, 13 | 0.86 |
Cortical gray matter | 197,085 | 6,765 | 197,839 | 6,765 | 0.4 | 0.01 | 1, 13 | 0.93 |
Cortical white matter | 148,689 | 5,125 | 148,928 | 5,125 | 0.2 | 0.00 | 1, 13 | 0.97 |
Cerebellum | 24,123 | 918 | 24,022 | 918 | −0.4 | 0.02 | 1, 13 | 0.91 |
TABLE 4. Brain Volumes of Male and Female Infants at High Genetic Risk for Schizophrenia and Comparison Infantsa
Diffusion Tensor Imaging Tractography
Diffusion tensor imaging scans were obtained for 19 matched pairs (six male and 13 female infants per group). There were no group differences in fractional anisotropy or mean diffusivity for any region in the genu and splenium of the corpus callosum or in the left and right corticospinal tracts (Table 5).
Measure and Position Tract | Position Track | Comparison Infants | High-Risk Infants | F Test for Difference | ||||
---|---|---|---|---|---|---|---|---|
Least Squares Mean | SE | Least Squares Mean | SE | F | df | p | ||
Mean diffusivity (10−4 mm2/sec) | ||||||||
Right cortical genu | −21 | 14.38 | 0.37 | 14.40 | 0.37 | 0.00 | 1, 18 | 0.96 |
Central genu | 0 | 13.44 | 0.28 | 13.48 | 0.28 | 0.02 | 1, 18 | 0.89 |
Left cortical genu | 21 | 13.97 | 0.39 | 14.17 | 0.39 | 0.19 | 1, 18 | 0.67 |
Right cortical splenium | −24 | 14.88 | 0.29 | 15.18 | 0.29 | 0.88 | 1, 18 | 0.37 |
Central splenium | 0 | 14.28 | 0.25 | 13.95 | 0.25 | 0.61 | 1, 18 | 0.45 |
Left cortical splenium | 24 | 14.48 | 0.34 | 15.30 | 0.34 | 4.90 | 1, 17 | 0.05a |
Left central corticospinal tract | −12 | 9.95 | 0.17 | 9.89 | 0.17 | 0.08 | 1, 18 | 0.79 |
Left cortical corticospinal tract | 9 | 12.28 | 0.38 | 12.68 | 0.38 | 0.89 | 1, 18 | 0.36 |
Right central corticospinal tract | −12 | 9.96 | 0.18 | 10.13 | 0.19 | 0.68 | 1, 18 | 0.43 |
Right cortical corticospinal tract | 9 | 12.04 | 0.30 | 12.56 | 0.32 | 2.14 | 1, 18 | 0.17 |
Fractional anisotropy | ||||||||
Right cortical genu | −21 | 0.25 | 0.01 | 0.25 | 0.01 | 0.13 | 1, 18 | 0.73 |
Central genu | 0 | 0.51 | 0.02 | 0.52 | 0.02 | 0.47 | 1, 18 | 0.51 |
Left cortical genu | 21 | 0.24 | 0.01 | 0.26 | 0.01 | 1.52 | 1, 18 | 0.24 |
Right cortical splenium | −24 | 0.29 | 0.02 | 0.29 | 0.02 | 0.06 | 1, 18 | 0.81 |
Central splenium | 0 | 0.55 | 0.01 | 0.57 | 0.01 | 1.31 | 1, 18 | 0.27 |
Left cortical splenium | 24 | 0.27 | 0.01 | 0.29 | 0.01 | 0.58 | 1, 17 | 0.46 |
Left central corticospinal tract | −12 | 0.50 | 0.02 | 0.48 | 0.02 | 0.48 | 1, 18 | 0.50 |
Left cortical corticospinal tract | 9 | 0.31 | 0.02 | 0.30 | 0.02 | 0.40 | 1, 18 | 0.54 |
Right central corticospinal tract | −12 | 0.51 | 0.01 | 0.52 | 0.01 | 0.24 | 1, 18 | 0.64 |
Right cortical corticospinal tract | 9 | 0.30 | 0.02 | 0.30 | 0.02 | 0.00 | 1, 18 | 0.98 |
TABLE 5. White Matter Tract Diffusion Properties of 19 Matched Pairs of Infants at High Genetic Risk for Schizophrenia and Comparison Infants
Discussion
To our knowledge, this is the first study of neonatal brain structure in children at high genetic risk for schizophrenia. And while this study has limitations, we unexpectedly found that male high-risk neonates have significantly larger intracranial, CSF, gray matter, and lateral ventricle volumes than male comparison infants, while female high-risk neonates have brain volumes similar to those of female comparison infants. Our findings suggest that prenatal and early neonatal brain development is abnormal in males at genetic risk for schizophrenia and that some brain structure endophenotypes associated with risk for schizophrenia may be present at birth.
Schizophrenia is increasingly considered a disorder of cortical connectivity, evidenced by lower than normal levels of cortical synaptic markers (30), spine density (31), and dendritic complexity (32) in postmortem studies and by low gray matter volume in imaging studies. It is unclear when the abnormalities observed in patients with schizophrenia or their unaffected first-degree relatives arise. In the human cortex, synapse development occurs rapidly in the first years of life; the number of synapses plateaus prior to puberty and then regresses after puberty (33, 34). This general pattern is evident in the development of cortical gray matter volume, which increases rapidly in the first 2 years of life (26, 35) and decreases after puberty (36). Our finding of larger cortical gray matter volumes in male neonates at risk for schizophrenia suggests that abnormalities of gray matter may arise early in brain development. In the first 2 years of life, there is enormous growth of cortical gray matter, which increases 185% from birth (35). This period of rapid growth of synaptic connections and dendritic complexity would seem a likely period in which gray matter abnormalities might become evident in high-risk children.
There is evidence in our study that male high-risk children have larger brains than comparison subjects. This is a somewhat unexpected finding and must be considered in light of the small number of subjects. However, this pattern of enlargement is somewhat reminiscent of the larger neonatal brain volumes, especially larger gray matter volumes associated with larger lateral ventricle volumes, observed in children with prenatal mild ventriculomegaly (37). The larger cortical gray matter volumes are also reminiscent of the macrocephaly observed in autism (38), perhaps implicating similar early developmental trajectories in these disorders, which have some overlapping behavioral features. A recent twin study we conducted indicates that in the neonatal period, the heritability of gray matter volume is 0.56, less than that observed in adults, while the heritability of lateral ventricle volume is 0.71, higher than that observed in adults (39). The larger gray matter volumes observed in this cohort may reflect genetic and environmental risk factors, while the enlarged ventricles may reflect more genetic risk.
It is tempting to speculate that in some forms of schizophrenia, larger than normal neonatal gray matter is an early indication of abnormal connectivity in the developing cortex, an abnormal connectivity that persists throughout brain development and may ultimately result in low cortical gray matter volumes as developmental trajectories play out over childhood. For example, low gray matter volumes are seen not only in adults with schizophrenia but also in children with early-onset schizophrenia (24). Of course, much larger groups and longitudinal studies are needed to determine this with any certainty. Adolescence, a period of significant synaptic reorganization and elimination (33, 34), also represents another period of brain development and a period in which abnormalities in gray matter may likely emerge. Alternatively, gray matter abnormalities could also occur after the onset and during the course of the illness (36). This question turns on whether gray matter abnormalities occur as a consequence of altered neurodevelopment or pathophysiologic processes inherent in the disorder.
Several of the genes that may increase risk for schizophrenia, including NRG1, erbB4, DISC1, and DTNBP1, have roles in synapse development and plasticity (7, 8), the effects of which might become apparent in the period of rapid postnatal synapse development. Finally, studies in twins suggest that the heritability of gray matter volume increases with age (39), additional evidence that genetic effects on gray matter development would become more evident through postnatal brain development.
Diffusion tensor imaging has revealed widespread abnormalities of white matter in people with schizophrenia and those at genetic high risk; these abnormalities include major white matter tracts of the corpus callosum and internal capsule (11, 12). We detected no group differences in diffusion tensor imaging properties in any of the white matter tracts studied. This suggests that white matter abnormalities may emerge after the neonatal period. It appears that major white matter tracts are established at birth, while myelination and maturation of diffusion properties in these tracts occur rapidly over the first 2 years of life, with a more gradual attainment of adult levels thereafter (28, 40, 41). The first 2 years of life, with their rapid growth and maturation, may be a period when white matter abnormalities associated with schizophrenia risk emerge.
A previous retrospective prenatal ultrasound study showed that lateral ventricle width in the offspring of mothers with schizophrenia was not greater than normal (42). We found no differences in prenatal ventricle width, supporting the findings of Clarke et al. (42), although we did find larger than normal neonatal ventricle volume in males. This suggests that the higher lateral ventricle volume observed in high-risk neonates may arise after the 32-week ultrasound or that the two-dimensional ultrasound is not a sensitive measure of prenatal lateral ventricle volume.
This study has several limitations. While the number of subjects is large in terms of the difficulty in recruiting and imaging this high-risk group, it is small given the heterogeneity of schizophrenia (and risk for schizophrenia) and the relative inconsistencies of imaging findings in older subjects. With our study group size, we had 0.8 power to detect differences in gray matter volume of about 10% (43). The magnitude of volume differences in adults with schizophrenia is typically less than 10%, and it is even less in studies of unaffected siblings at genetic risk. Most of the mothers of the high-risk subjects in this study took antipsychotics during pregnancy. Previous studies have shown that antipsychotics do not appear to significantly increase the risk of malformations or significant pregnancy outcomes (44), and studies in nonhuman primates suggest that chronic antipsychotic exposure can decrease cortical gray matter volumes (45), although the effect of antipsychotics on prenatal cortical development in humans is unknown. Our finding of no large differences in neonatal brain structure or white matter integrity in the female offspring of mothers with schizophrenia suggests that antipsychotics during pregnancy do not have a major impact on brain structure, although gender-specific effects cannot be ruled out. The mothers with schizophrenia were also more likely to have smoked tobacco and to have used illicit substances during pregnancy, and each of these factors could have confounded the results, although the expected direction of effects would be toward smaller overall head and brain sizes (46). As already noted, we did not find significant differences in volumes between the high-risk neonates exposed to maternal smoking and those who were not exposed. Otherwise, the high-risk and comparison groups were reasonably well matched for pre- and perinatal factors. The absence of detectable abnormalities in the female high-risk neonates in this study does not mean prenatal brain development is normal, as more subtle, undetectable differences in gray and white matter development may be present. Finally, we would expect only about 10% of the high-risk neonates to ultimately develop schizophrenia, although observed abnormalities may represent intermediate phenotypes of risk.
In summary, male neonates at high risk for schizophrenia appear to have larger than normal gray matter, CSF, and lateral ventricle volumes, and this appears to be the first evidence that neonatal brain development is abnormal in individuals at genetic risk for schizophrenia. It is suggested that the period of rapid cortical synapse development and gray matter volume growth in the first 2 years of postnatal life represents an important period in which the abnormalities of “connectivity” thought to underlie risk for schizophrenia may emerge. Neuropsychological studies of children genetically at risk for schizophrenia have revealed a variety of neuropsychological and motor abnormalities that are apparent early in life (47–49); the structural abnormalities that underlie them may also arise in the first years of life. We are following this cohort through this period of rapid postnatal brain development to study how these structural brain abnormalities progress after birth.
1. : Is schizophrenia a neurodevelopmental disorder? Br Med J (Clin Res Ed) 1987; 295:681–682Crossref, Medline, Google Scholar
2. : Implications of normal brain development for the pathogenesis of schizophrenia. Arch Gen Psychiatry 1987; 44:660–669Crossref, Medline, Google Scholar
3. : Prenatal and perinatal factors, in American Psychiatric Publishing Textbook of Schizophrenia. Edited by Lieberman JAStroup TSPerkins DO. Washington, DC, American Psychiatric Publishing, 2006, pp 55–67Google Scholar
4. : Child development risk factors for adult schizophrenia in the British 1946 birth cohort. Lancet 1994; 344:1398–1402Crossref, Medline, Google Scholar
5. : Evidence for early-childhood, pan-developmental impairment specific to schizophreniform disorder: results from a longitudinal birth cohort. Arch Gen Psychiatry 2002; 59:449–456Crossref, Medline, Google Scholar
6. : Neurobehavioral deficits in offspring of schizophrenic parents: liability indicators and predictors of illness. Am J Med Genet 2000; 97:65–71Crossref, Medline, Google Scholar
7. : Neurodevelopmental mechanisms of schizophrenia: understanding disturbed postnatal brain maturation through neuregulin-1-ErbB4 and DISC1. Trends Neurosci 2009; 32:485–495Crossref, Medline, Google Scholar
8. : The role of genes involved in neuroplasticity and neurogenesis in the observation of a gene-environment interaction (G×E) in schizophrenia. Curr Mol Med 2009; 9:506–518Crossref, Medline, Google Scholar
9. : Meta-analysis of regional brain volumes in schizophrenia. Am J Psychiatry 2000; 157:16–25Link, Google Scholar
10. : Brain volume in first-episode schizophrenia: systematic review and meta-analysis of magnetic resonance imaging studies. Br J Psychiatry 2006; 188:510–518Crossref, Medline, Google Scholar
11. : A review of diffusion tensor imaging studies in schizophrenia. J Psy-chiatr Res 2007; 41:15–30Crossref, Medline, Google Scholar
12. : Recent diffusion tensor imaging findings in early stages of schizophrenia. Curr Opin Psychiatry 2009; 22:168–176Crossref, Medline, Google Scholar
13. : A controlled study of brain structure in monozygotic twins concordant and discordant for schizophrenia. Biol Psychiatry 2004; 56:454–461Crossref, Medline, Google Scholar
14. : Cortex mapping reveals regionally specific patterns of genetic and disease-specific gray-matter deficits in twins discordant for schizophrenia. Proc Natl Acad Sci USA 2002; 99:3228–3233Crossref, Medline, Google Scholar
15. : Regional gray matter, white matter, and cerebrospinal fluid distributions in schizophrenic patients, their siblings, and controls. Arch Gen Psychiatry 1998; 55:1084–1091Crossref, Medline, Google Scholar
16. : Association of genetic risks for schizophrenia and bipolar disorder with specific and generic brain structural endophenotypes. Arch Gen Psychiatry 2004; 61:974–984Crossref, Medline, Google Scholar
17. : Regionally specific cortical thinning and gray matter abnormalities in the healthy relatives of schizophre-nia patients. Cereb Cortex 2007; 17:415–424Crossref, Medline, Google Scholar
18. : Brain volumes in relatives of patients with schizophrenia: a meta-analysis. Arch Gen Psychiatry 2007; 64:297–304Crossref, Medline, Google Scholar
19. : Is gray matter volume an intermediate phenotype for schizophrenia? a voxel-based morphometry study of patients with schizophrenia and their healthy siblings. Biol Psychia-try 2008; 63:465–474Crossref, Medline, Google Scholar
20. : Widespread reductions of cortical thickness in schizophrenia and spectrum disorders and evidence of heritability. Arch Gen Psychiatry 2009; 66:467–477Crossref, Medline, Google Scholar
21. : Brain structure, genetic liability, and psychotic symptoms in subjects at high risk of developing schizophrenia. Biol Psychiatry 2001; 49:811–823Crossref, Medline, Google Scholar
22. : Grey matter changes over time in high risk subjects developing schizophrenia. Neuroimage 2005; 25:1023–1030Crossref, Medline, Google Scholar
23. : A DTI study of white matter microstructure in individuals at high ge-netic risk for schizophrenia. Schizophr Res 2008; 106:115–124Crossref, Medline, Google Scholar
24. : Cortical brain development in non-psychotic siblings of patients with childhood-onset schizophrenia. Arch Gen Psychiatry 2007; 64:772–780Crossref, Medline, Google Scholar
25. : Biometry of the fetal brain, in Ultrasonography of the Prenatal and Neonatal Brain. Edited by Timor-Tritsch IEMonteagudo ACohen HL. Stamford, Conn, Appleton & Lange, 1996, pp 89–146Google Scholar
26. : Regional gray matter growth, sexual dimorphism, and cerebral asymmetry in the neonatal brain. J Neurosci 2007; 27:1255–1260Crossref, Medline, Google Scholar
27. : User-guided 3D active contour segmentation of anatomical structures: significantly improved efficiency and reliability. Neuroimage 2006; 31:1116–1128Crossref, Medline, Google Scholar
28. : Early postnatal development of corpus callosum and corticospinal white matter assessed with quantitative tractography. AJNR Am J Neuroradiol 2007; 28:1789–1795Crossref, Medline, Google Scholar
29. : Intracranial hemorrhage in asymptomatic neonates: prevalence on MR images and relationship to obstetric and neonatal risk factors. Radiology 2007; 242:535–541Crossref, Medline, Google Scholar
30. : Reduction of synaptophysin immunoreactivity in the prefrontal cortex of subjects with schizophrenia: regional and diagnostic specificity. Arch Gen Psychiatry 1997; 54:943–952Crossref, Medline, Google Scholar
31. : Decreased dendritic spine density on prefrontal cortical pyramidal neurons in schizophrenia. Arch Gen Psychiatry 2000; 57:65–73Crossref, Medline, Google Scholar
32. : Pathology of layer V pyramidal neurons in the pre-frontal cortex of patients with schizophrenia. Am J Psychiatry 2004; 161:742–744Link, Google Scholar
33. : Regional differences in synaptogenesis in human cerebral cortex. J Comp Neurol 1997; 387:167–178Crossref, Medline, Google Scholar
34. : Synaptophysin and postsynaptic density protein 95 in the human prefrontal cortex from mid-gestation into early adulthood. Neuroscience 2007; 149:582–591Crossref, Medline, Google Scholar
35. : A structural MRI study of human brain develop-ment from birth to 2 years. J Neurosci 2008; 28:12176–12182Crossref, Medline, Google Scholar
36. : Neurodevelopmental trajectories of the human cerebral cortex. J Neurosci 2008; 28:3586–3594Crossref, Medline, Google Scholar
37. : Prenatal mild ventriculomegaly predicts abnormal development of the neonatal brain. Biol Psychiatry 2008; 64:1069–1076Crossref, Medline, Google Scholar
38. : An MRI and head circumference study of brain size in autism: birth through age two years. Arch Gen Psychiatry 2005; 62:1366–1376Crossref, Medline, Google Scholar
39. : Genetic and environmental contributions to neonatal brain structure: a twin study. Hum Brain Mapp (in press)Google Scholar
40. : Maturation of white matter in the human brain: a review of magnetic resonance studies. Brain Res Bull 2001; 54:255–266Crossref, Medline, Google Scholar
41. : Diffusion-tensor MR imaging of gray and white matter development during normal human brain maturation. AJNR Am J Neuroradiol 2002; 23:1445–1456Medline, Google Scholar
42. : Foetal brain development in offspring of women with psychosis. Br J Psychiatry 2007; 190:445–446Crossref, Medline, Google Scholar
43. :
44. : Use and safety of antipsychotic drugs during pregnancy. J Psychiatr Pract 2009; 15:183–192Crossref, Medline, Google Scholar
45. : The influence of chronic exposure to antipsychotic medications on brain size before and after tissue fixation: a comparison of haloperidol and olanzapine in macaque monkeys. Neuropsychopharmacology 2005; 30:1649–1661Crossref, Medline, Google Scholar
46. : Volumetric MRI study of brain in children with intrauterine exposure to cocaine, alcohol, tobacco, and marijuana. Pediatrics 2008; 121:741–750Crossref, Medline, Google Scholar
47. : Neurological dysfunctioning in offspring of schizophrenics in Israel and Denmark: a replication analysis. Arch Gen Psychiatry 1985; 42:753–761Crossref, Medline, Google Scholar
48. : Infants at risk for schizophrenia: sequelae of a genetic neurointegrative defect: a review and replication analysis of pandysmaturation in the Jerusalem Infant Development Study. Arch Gen Psychiatry 1992; 49:221–235Crossref, Medline, Google Scholar
49. : Neuropsychological risk indicators for schizophrenia: a review of family stud-ies. Schizophr Bull 1994; 20:103–119Crossref, Medline, Google Scholar