Inhibition of Motor Responses in Siblings Concordant and Discordant for Attention Deficit Hyperactivity Disorder
Abstract
OBJECTIVE: The authors sought to determine whether nonaffected siblings of ADHD probands have a motor response inhibition deficit and to assess concordance for this inhibition deficit in ADHD-concordant and ADHD-discordant sibling pairs. METHOD: ADHD-concordant pairs (21 probands and their affected siblings), ADHD-discordant pairs (18 probands and their nonaffected siblings), and a group of unrelated, demographically balanced, healthy individuals (N=24) were compared on measures of response inhibition, ADHD behavior, impairment, and environmental risk. RESULTS: Concordant-pair probands, their affected siblings, and discordant-pair probands exhibited inhibitory control impairment relative to healthy comparison subjects. The performance of nonaffected siblings was intermediate between that of ADHD children and the healthy comparison subjects. Group differences persisted after age was controlled, and performance was not correlated with the number of ADHD symptoms. In ADHD-concordant sibling pairs, there was a significant relationship between proband and sibling inhibition deficit. In ADHD-discordant sibling pairs, inhibition deficit was evident in half of the nonaffected siblings of probands with an inhibition deficit. Groups did not differ in exposure to environmental risks. CONCLUSIONS: Impaired inhibitory control aggregates in the family members of individuals with ADHD and may serve as an indicator of genetic vulnerability to the disorder.
Attention deficit hyperactivity disorder (ADHD) is a common and impairing psychiatric illness that commences in childhood and often persists throughout life. There is a substantial multigenic component to the transmission of ADHD (1). Although existing diagnostic methods and criteria have facilitated identification of several susceptibility genes, the ADHD phenotype may be suboptimal for genetic analysis because of nongenetic phenocopies, incomplete penetrance, imprecision in measurement of the phenotype, and variation in thresholds and criteria for diagnosis. Therefore, there is considerable uncertainty about which individuals should receive an “ADHD” diagnosis in genetic research.
One solution to these problems is to identify characteristics known as phenotypic indicators or endophenotypes, which mark the presence or degree of genetic predisposition to a disorder (2). Endophenotypes mediate between genes at the causal level and behavioral phenotypes at the clinical level and are closer to the actual effect of susceptibility genes. Endophenotypes could increase power to detect genetic risks by identifying individuals with a spectrum phenotype, distinguishing genetically homogenous ADHD subtypes, or identifying a quantitative trait that marks genetic risk for the disorder. Valid endophenotypes should be biologically meaningful, evident in affected individuals and in their unaffected relatives, and more common in affected relatives of affected individuals than in unaffected relatives. Endophenotypes should not be highly correlated with the severity of overt clinical symptoms, otherwise they would be unable to detect asymptomatic genetic carriers.
Deficits in executive control processes are logical candidates for endophenotypes of ADHD. Lesion-deficit, structural, and functional imaging studies in animals and humans have indicated that executive processes are implemented in circuits involving the prefrontal cortex, basal ganglia, and thalamus (3). Genetic factors acting anywhere in these circuits could produce executive control deficits, but the specific site of the physiological or neurochemical dysfunction will determine the particular manifestations of the disorder.
Motor response inhibition refers to the voluntary act of stopping an ongoing response. Although it is only one of various forms of inhibition (compare with distractor inhibition, inhibition of return), it is a logical candidate for an endophenotype because of its strong link to ADHD and its link to the proposed genetic, neural, and pharmacological basis of the disorder. Motor inhibition activates the medial frontal and dorsolateral prefrontal cortex (4), areas of the brain that may be abnormal in ADHD (5). Inhibition varies in animals with levels of neurotransmitters implicated in ADHD and in humans with drugs that impact neurotransmission and improve behavior (6). An inhibition deficit in ADHD has been demonstrated and extensively replicated using the stop task (7–9). Inhibition deficit is not evident in subjects with other disorders such as anxiety or learning disorders or conduct disorder with no ADHD comorbidity (9), nor is it consistently evident in those with a reading disorder (10). The deficit is evident in children and adults with a diagnosis of ADHD (11) and is stable over time (12).
Compared with ADHD subjects with “good” inhibition, “poor” inhibitors have significantly higher rates of ADHD among family members (48% versus 19%) (13). The increase in rate of affected family members is specific to deficient inhibition: good and poor inhibitors do not differ in speed of response execution, sex, IQ, severity of inattentive or hyperactive-impulsive symptoms, or rate of associated conduct or reading disorder. This result suggests that inhibitory control can serve as a continuous measure of genetic risk for ADHD that cuts across ADHD subtype, comorbidity, intelligence, and sex.
This study aimed to determine whether a deficit in response inhibition as measured with the stop task is evident in siblings of ADHD individuals as would be expected if it were a valid endophenotype of ADHD. We compared two groups of ADHD probands and their siblings with a group of healthy comparison subjects. In one group, both the proband and the sibling met criteria for ADHD (concordant pairs). In the second group, the proband but not the sibling met criteria for ADHD (discordant pairs). We predicted that both siblings in the ADHD-concordant pairs would show a deficit in inhibition relative to the healthy comparison subjects. Such a finding would confirm previous observations of an inhibition deficit in ADHD. Furthermore, we predicted that ADHD probands with an inhibition deficit would be more likely than those without an inhibition deficit to have an ADHD sibling with an inhibition deficit, thus supporting the conclusion that the inhibition deficit is associated with a shared genetic risk. If only a subset of ADHD genes were required to cause inhibition deficit, then some of the nonaffected siblings of ADHD probands would show inhibition deficit similar to that of ADHD children (2). As a result, ADHD probands as a group would exhibit a deficit that was intermediate between that of affected individuals and that of healthy comparison subjects. We also predicted that nonaffected siblings with an inhibition deficit would have probands with an inhibition deficit.
Siblings share aspects of their environments as well as 50% of their genes on average. Consequently, we compared groups with respect to exposure to environmental risks (e.g., prematurity) and examined the association of these risks with inhibition. We assessed the relationship of ADHD symptom counts and psychosocial impairment with inhibition in order to determine whether an intermediate cognitive deficit in nonaffected siblings was a result of an intermediate behavioral phenotype. Finally, we took age, IQ, and ADHD subtype into account, since they could affect performance on cognitive tasks.
Method
Subjects
ADHD participants and their siblings were drawn from a clinic for children with attention and behavior problems. The sample was similar in socioeconomic status and ethnicity to that of the community from which it was drawn. Healthy comparison subjects were recruited through newspaper advertisements. They were assessed in the same way as ADHD cases. We accepted participants who had been treated or who were currently being treated with stimulant medication such as methylphenidate, but no other medications were permitted. We assessed participants free of medication for a minimum of 24 hours. The protocol was approved by the Research Ethics Board, and written informed consent was obtained.
Diagnostic Instruments
The Parent Interview for Child Symptoms and the Teacher Telephone Interview (unpublished instruments of A. Ickowicz et al. and R. Tannock et al., respectively; available from first author upon request) were our primary assessment measures for ADHD and all other axis I diagnoses as well as for family history of psychopathology and developmental, medical, and social history. The parent interview is highly reliable (e.g., 80% agreement for ADHD diagnosis; intraclass correlation >0.90 for ADHD symptoms). The teacher interview covers disruptive behavior disorders and screens for other disorders. For both measures, symptom presence was judged by the interviewer using specific criteria in an extensive manual. All interviewers were trained to a criterion of 90% symptom agreement before the study began, and all interviews were recorded so that we could maintain surveillance, assess reliability, and prevent criterion shift. Total ADHD symptom scores were calculated by summing ADHD items on the parent and teacher interviews. A social worker conducted the parent interview, and a research assistant with a master’s degree conducted the teacher interview.
Intellectual ability (WISC-III [14]), reading ability (word identification and word attack tasks from the Woodcock Reading Mastery Test—Revised [15]), language skills (Clinical Evaluation of Language Fundamentals, 3rd ed. [16]), pure tone hearing, and vision were assessed. The parent, teacher, and child assessments were conducted without knowledge of the screening diagnosis or the results of other portions of the assessment.
Environmental Risk
The Ontario Child Health Survey Scales—Family and Household Form (17) was used to record exposure to environmental risks (e.g., pregnancy and perinatal complications, drug or alcohol use). Risk factors were examined in several ways. First, we computed a risk factor score by giving the presence of each risk equal weighting and summing all risks (full index). Second, we identified all risk factors that were more common in a large clinic sample of 208 ADHD cases than in a comparison group. We then computed a factor score by summing these items (refined index). Finally, we identified individuals who had more than four risks present to identify a group of subjects who had exposure to multiple risk factors.
Impairment
Impairment in the home, school, and community was assessed with the parent and teacher ratings on the Ontario Child Health Study scales (18). Impairment scores were standardized by age and gender using general population norms.
Inclusion and Exclusion Criteria
Probands and affected siblings were 7 to 16 years of age and attending a primary or high school in order that both a teacher and a parent could act as informants. Children met DSM-IV criteria for ADHD (at least six of nine inattentive symptoms, six of nine hyperactive-impulsive symptoms, or both). Children were at least moderately impaired in two settings as necessitated by DSM-IV: they met criteria for ADHD according to either the parent or the teacher interview, exhibited a minimum of four symptoms according to the second informant, and had at least moderate impairment on the parent or the teacher impairment scale. Participants were categorized into ADHD subtypes on the basis of all information.
Participants were excluded if any of the following were present: 1) IQ below 80 on both verbal and performance scales of the WISC-III; 2) pervasive developmental disorder, psychosis, obsessive-compulsive disorder, Tourette syndrome, serious medical illness, substance abuse, or history of loss of consciousness; 3) concurrent treatment with medication other than a stimulant or treatment with medication within 24 hours of testing; 4) language impairment (Clinical Evaluation of Language Fundamentals score < 85); 5) a history of abuse; or 6) the sibling was a step-sibling or was adopted. About 10% of cases were excluded. Participants were assigned to the ADHD-concordant pairs group if both proband and sibling met criteria for ADHD. If both siblings met ADHD criteria, the participant who was initially referred was designated as the proband. Participants were assigned to the ADHD-discordant pairs group if the proband met criteria for ADHD and the sibling did not.
Motor Response Inhibition
The stop task is a laboratory paradigm that measures the speed with which one can voluntarily inhibit a speeded motor response (19). The stop task involved two concurrent tasks. The go task was a choice reaction time task (discriminating an X from an O presented on a computer screen) that participants performed as quickly and as accurately as possible. The stop task involved a tone emitted from the computer on 25% of the go task trials that signaled participants to withhold their response on that trial. The delay between the onset of the go and stop task was manipulated in 50-msec steps to converge on the delay at which individuals were able to stop 50% of the time. We could estimate the latency of the unobserved inhibition process (stop signal reaction time) by subtracting mean delay (at which the subject inhibits 50% of the time) from mean go reaction time. Calculated in this way, stop signal reaction time is distinct from the speed of response execution (19).
The task involved 128 trials of which 32 involved stop signals and 96 did not. The go task stimulus was presented for 1000 msec immediately following a fixation point of 500 msec. The stop tone was a 1000-Hz tone emitted by the computer and presented by headphones at a comfortable listening level. Dependent measures were accuracy of go task performance, mean go task reaction time (msec), standard deviation of correct go task reaction time (msec), and probability of successful inhibition. Stop signal reaction time was estimated by subtracting mean delay at which the subject inhibits 50% of the time from mean go reaction time (19).
Statistical Analyses
Group differences were assessed in univariate analyses of variance with five study groups (ADHD concordant-pair probands, their affected siblings, ADHD discordant-pair probands, their nonaffected siblings, and unrelated healthy comparison subjects) serving as the between-subject factors using Statistical Package for the Social Sciences Version 9 (20). Square root transformed stop signal reaction time was used in the analyses to stabilize variance. Specific hypotheses about ADHD-concordant and ADHD-discordant sibling pairs were tested with one-tailed planned comparisons: ADHD probands versus affected siblings, ADHD probands versus nonaffected siblings, and all these groups together versus healthy comparison subjects. In addition, we compared nonaffected siblings with healthy subjects. Univariate analysis of covariance was used to examine the effect of covariates (age) on intergroup differences in inhibition. Odds ratios were used to test the significance of the relationship between proband and sibling executive control deficits in concordant and discordant pairs. To classify participants as having an inhibition deficit or not we conducted a sensitivity/specificity analysis to identify the stop signal reaction time score (233.7 msec) that best distinguished the performance of 208 ADHD and 92 comparison subjects from a larger database (sensitivity=0.71, specificity=0.66). In order to test the specificity of any observed relationship of stop signal reaction time and group membership, we examined the relationship between siblings on go reaction time in the stop task. We defined go task accuracy deficit (i.e., slow response execution speed) as go task accuracy longer than mean plus one standard deviation on the basis of healthy comparison subject performance (712.3 msec). The association of ADHD symptom scores and stop signal reaction time was examined to determine whether the “intermediate” inhibitory control performance of nonaffected siblings was a function of their “intermediate” behavioral phenotype.
Results
Groups did not differ in terms of mean intelligence or word identification performance (Table 1), but significant differences were seen for age (F=3, df=4, 97, p<0.05); parent and teacher ratings of impairment (F=10.7, df=4, 75, p<0.001 and F=4.3, df=4, 67, p<0.001, respectively); parent and teacher ratings of inattentiveness (F=21.6, df=4, 97, p<0.001 and F=31.3, df=4, 83, p<0.001); parent and teacher ratings of hyperactivity-impulsiveness (F=24.9, df=4, 97, p<0.001 and F=13.7, df=4, 83, p<0.001); and word attack performance (F=3.52, df=4, 90, p<0.05). The pattern of these differences was similar: on measures of ADHD behavior and impairment, the nonaffected siblings of ADHD probands had scores that were intermediate between those of the other ADHD groups and the healthy comparison group. On the word attack measure, nonaffected siblings of ADHD probands performed similar to healthy comparison subjects and were significantly better than their ADHD siblings. ADHD groups did not differ in subgroup composition or in rate of comorbid oppositional defiant disorder, conduct disorder, or reading disability. There were significantly more girls in the healthy comparison group than in the ADHD groups (χ2=4.30, df=1, p<0.04).
Groups did not differ in the probability of inhibiting a response (indicating that the tracking algorithm was effective in all groups), go reaction time, or variability of go task accuracy (Table 2). However, groups differed significantly in mean stop signal reaction time (F=3.9, df=4, 97, p=0.005) (Figure 1). A power calculation for this analysis indicated 89% power. Planned comparisons indicated that ADHD probands did not differ from their affected siblings, that ADHD probands did not differ from their unaffected siblings, and that all four ADHD groups (ADHD concordant-pair probands, affected siblings, ADHD discordant-pair probands, nonaffected siblings) differed from healthy comparison subjects. Nonaffected siblings had worse inhibitory control than the healthy comparison subjects, although the planned comparison just failed to reach conventional significance level (effect size=0.45; p=0.055).
Stop signal reaction time was correlated with age (r=–0.36, df=100, p<0.001) but not with intelligence (r=–0.08) or performance on the word attack (r=–0.19) or word identification (r=–0.20) tasks. Consequently, group scores for stop signal reaction time were compared in an analysis of covariance taking age into account. The effect of age was significant (F=14.7, df=1, 96, p<0.001), but the effect of study group remained (F=3.6, df=4, 96, p<0.05).
Stop signal reaction time was not correlated with parent and teacher symptom counts of inattentiveness or hyperactivity/impulsiveness, presence of oppositional defiant disorder or conduct disorder, impairment ratings, or reading scores in any study groups. No difference was observed between boys and girls in stop task performance.
There was little concordance for subtype among the ADHD-concordant sibling pairs (<30%), which suggests that concordance at the cognitive level was not a function of concordance in ADHD subtype. Moreover, ADHD subtypes did not differ in stop signal reaction time, go task accuracy, go task accuracy variability, or mean go task accuracy.
Groups did not differ in environmental risk factor scores. Only one of the 23 individual risk factors investigated, breech birth, showed a group difference (χ2=11.78, df=4, p<0.10). The result showed a higher incidence of breech birth in nonaffected siblings in the ADHD-discordant pairs compared with all other groups.
There was a significant association between proband and sibling inhibitory control deficit in concordant pairs (odds ratio=12.0, 95% confidence interval=1.02–141.3; χ2=4.89, df=1, p<0.05). In the ADHD-concordant group, 16 of 21 sibling pairs (76%) were concordant for inhibition performance (Table 3). If a proband had an inhibition deficit it was likely that their sibling would have a deficit. In the ADHD-discordant group, 10 of 18 sibling pairs (55.6%) were concordant for an inhibition deficit. Typically, nonaffected siblings with an inhibition deficit had an affected sibling with inhibition deficit (seven of nine pairs [77.8%]). About half of the ADHD probands in the discordant pairs group with an inhibition deficit had a nonaffected sibling with an inhibition deficit. The association among siblings for go task accuracy deficit was not significant (data not shown).
Discussion
Individuals with a diagnosis of ADHD had longer stop signal reaction times than healthy comparison subjects, confirming a large body of previous research (9). As predicted, nonaffected siblings of ADHD probands had an inhibition deficit that was intermediate between that of their ADHD siblings and healthy comparison subjects. Moreover, we observed the predicted concordance for inhibitory control deficit in sibling pairs: 76% of the ADHD-concordant sibling pairs were concordant for the presence or absence of an inhibitory control deficit. In ADHD-discordant sibling pairs, concordance for the inhibitory control deficit was lower than in the ADHD-concordant pairs but nevertheless was in keeping with the hypothesis of shared genetic risk for inhibition and ADHD. Of ADHD probands with an inhibitory control deficit, half had a nonaffected sibling with a deficit, and the majority of nonaffected siblings with an inhibition deficit had an ADHD proband sibling with a deficit.
The greater concordance of inhibition deficit in concordant siblings than in discordant siblings supports the genetic link between the ADHD phenotype and the inhibition deficit. If ADHD and inhibition were genetically independent, concordance of inhibition deficit would have been similar in siblings regardless of their ADHD status. Moreover, previous research has shown a strong and specific association between ADHD and deficient inhibition and increased risk for ADHD among relatives of ADHD probands with poor inhibition compared with those with good inhibition (13). Furthermore, we found that all unaffected relatives showed greater deficit than comparison subjects (albeit that the difference was significant at the 0.055 level). Taken together, available research indicates that inhibition deficit is familial, that there is genetic overlap of ADHD and deficient inhibition, and that inhibition may be a marker of genetic vulnerability to ADHD that is evident both in affected individuals and in those who are at genetic risk for ADHD even in the absence of the behavioral phenotype.
The observed role of deficient inhibition as a marker for ADHD vulnerability appears to be specific to inhibition. We did not observe any difference among the diagnostic groups in intelligence, in speed of response execution, or in sibling concordance for reaction time. Moreover, intelligence was not correlated with inhibition. It should be noted that stop signal reaction time is independent of go task accuracy in the stop signal paradigm.
As is typical of other studies, age and inhibition were significantly associated. However, group differences in inhibition remained after taking age into account. The magnitude of the inhibition deficit was not associated with the extent of ADHD symptoms or impairment in the nonaffected group. Therefore, the presence of an intermediate inhibition deficit in nonaffected siblings cannot be attributed solely to their intermediate behavioral phenotype. Comparison of extremely discordant pairs should be undertaken.
The weak correlation between the severity of behavioral manifestations of ADHD and inhibition might indicate that current measures of the ADHD phenotype are unreliable, invalid, or insensitive to cognitive deficit. Behavioral phenotypes can be difficult to measure and can be influenced by many factors such as the perceptions and biases of the informant. If there were a substantial correlation between the behavioral manifestations of the disorder and the putative endophenotype, that endophenotype would not be able to detect behaviorally asymptomatic or subthreshold carriers of the relevant genotype. Consequently, there would be no advantage to measuring an endophenotype to gauge the genetic risk to an apparently unaffected individual.
Environmental factors were not correlated with inhibition, and groups did not differ in exposure to environmental risks. This result is not consistent with previous reports of increased exposure to these risks among ADHD children (21) or of increased risk for ADHD among children with known exposure to risks such as head injury, perinatal complications, or fetal alcohol (21, 22). It is unlikely that the absence of an association between neurobiological environmental risks and risk for ADHD reflects insensitivity of our risk measures (23). Rather, it is more likely that environmental factors play only a small role in the development of ADHD (24) or that those participants with marked environmental risk adversity were not referred to our study.
There are several other important qualifications to these results. For example, we do not know whether concordance for cognitive deficit depends on whether sibling pairs are drawn from densely affected families. By definition, we know that ADHD-concordant sibling pairs came from families in which two members were affected. However, we do not know whether ADHD-discordant sibling pairs had other family members with ADHD.
There have been several other sibling studies in ADHD. Slaats-Willemse et al. (25) found an intermediate deficit in distractor inhibition, sustained attention, and impulsive responding in unaffected siblings of ADHD probands but did not examine concordance of these deficits. By contrast, Seidman et al. (26) did not identify any cognitive deficit in the unaffected siblings of ADHD probands on a range of laboratory measures. Apparently, some executive control processes are more sensitive than others to the vulnerability to ADHD.
There is a small but growing body of evidence for the heritability of specific executive control processes: cognitive interference (27), digit span (28), sustained attention (28), attention span (29), and phonological working memory (30). However, few studies have examined the putative heritability of executive control deficit in ADHD. Heritability of inhibition would provide strong support for its potential role as an endophenotype of ADHD and is an important area of future research.
It seems prudent to conduct further twin and family studies of motor inhibition and other executive control processes. Each may be under the influence of a partially distinct set of genes, only some of which affect executive control or contribute to ADHD. Quantitative traits that are derived from performance on multiple executive control measures may prove to be more useful for genetic research than endophenotypes based on a single cognitive process.
The power to detect genetic susceptibility factors can be increased by identifying heritable, specific, and biologically plausible cognitive deficits in ADHD. These endophenotypes could delineate subtypes of ADHD with increased genetic homogeneity, identify affected family members who cannot be identified as “at risk” using existing behavioral measures, or ascertain a quantitative trait for genetic study. Laboratory measures of cognitive deficit could afford a method of estimating the genetic risk for ADHD that is more consistent across sites and less subject to informant bias than are the current measures of the ADHD phenotype.
![]() |
![]() |
![]() |
Received Nov. 4, 2003; revisions received Jan. 20, April 6, and June 23, 2004; accepted July 19, 2004. From the Research Institute, the Department of Psychiatry, and the Division of Neurology, The Hospital for Sick Children, University of Toronto. Address correspondence and reprint requests to Dr. Schachar, The Hospital for Sick Children, 555 University Ave., Toronto M5N 2L1, Canada; russell.schachar@ sickkids.ca (e-mail). This research was funded by a grant from the Canadian Institutes of Health Research. The authors thank Derek Stephen for statistical consultation.
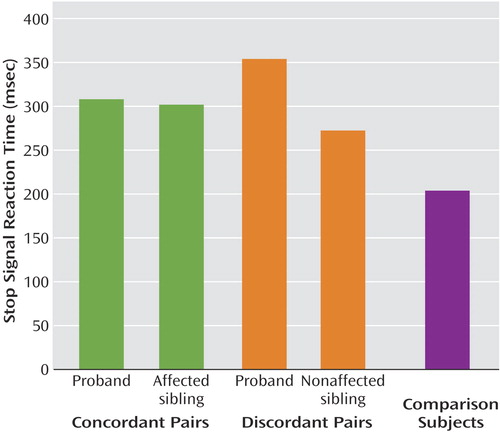
Figure 1. Motor Response Inhibition in ADHD-Concordant and ADHD-Discordant Sibling Pairs and Healthy Comparison Subjects
1. Thapar A, Holmes J, Poulton K, Harrington R: Genetic basis of attention deficit and hyperactivity. Br J Psychiatry 1999; 174:105–111Crossref, Medline, Google Scholar
2. Tsuang MT, Faraone SV, Lyons MJ: Identification of the phenotype in psychiatric genetics. Eur Arch Psychiatry Clin Neurosci 1993; 243:131–142Crossref, Medline, Google Scholar
3. Miller EK, Cohen JD: An integrative theory of prefrontal cortex function. Ann Rev Neurosci 2001; 24:167–202Crossref, Medline, Google Scholar
4. Rubia K, Overmeyer S, Taylor E, Brammer M, Williams SCR, Simmons A, Bullmore ET: Hypofrontality in attention deficit hyperactivity disorder during higher-order motor control: a study with functional MRI. Am J Psychiatry 1999; 156:891–896Link, Google Scholar
5. Giedd JN, Blumenthal J, Molloy E, Castellanos FX: Brain imaging of attention deficit/hyperactivity disorder. Ann NY Acad Sci 2001; 931:33–49Crossref, Medline, Google Scholar
6. Tannock R, Schachar R, Logan G: Methylphenidate and cognitive flexibility: dissociated dose effects in hyperactive children. J Abnorm Child Psychol 1995; 23:235–266Crossref, Medline, Google Scholar
7. Schachar R, Mota VL, Logan GD, Tannock R, Klim P: Confirmation of an inhibitory control deficit in attention-deficit/hyperactivity disorder. J Abnorm Child Psychol 2000; 28:227–235Crossref, Medline, Google Scholar
8. Schachar R, Logan GD: Impulsivity and inhibitory control in normal development and childhood psychopathology. Dev Psychol 1990; 26:710–720Crossref, Google Scholar
9. Oosterlaan J, Logan GD, Sergeant JA: Response inhibition in AD/HD, CD, comorbid AD/HD + CD, anxious, and control children: a meta-analysis of studies with the stop task. J Child Psychol Psychiatry 1998; 39:411–425Crossref, Medline, Google Scholar
10. Nigg JT, Hinshaw SP, Carte ET, Treuting JJ: Neuropsychological correlates of childhood attention-deficit/hyperactivity disorder: explainable by comorbid disruptive behavior or reading problems? J Abnorm Psychol 1998; 107:468–480Crossref, Medline, Google Scholar
11. Murphy P: Inhibitory control in adults with attention-deficit/hyperactivity disorder. J Atten Disord 2002; 6:1–4Crossref, Medline, Google Scholar
12. Kindlon D, Mezzacappa E, Earls F: Psychometric properties of impulsivity measures: temporal stability, validity and factor structure. J Child Psychol Psychiatry 1995; 36:645–661Crossref, Medline, Google Scholar
13. Crosbie J, Schachar R: Deficient inhibition as a marker for familial ADHD. Am J Psychiatry 2001; 158:1884–1890Link, Google Scholar
14. Wechsler D: Wechsler Intelligence Scale for Children, 3rd ed. San Antonio, Tex, Harcourt Brace, 1991Google Scholar
15. Woodcock RW: Woodcock Reading Mastery Test—Revised. Circle Pines, Minn, American Guidance Service, 1987Google Scholar
16. Semel E, Wiig EI, Secord WA: CELF 3: Clinical Evaluation of Language Fundamentals. San Antonio, Tex, Psychological Corp, 1995Google Scholar
17. Boyle MH, Offord DR, Racine Y, Szatmari P, Fleming JE, Sanford M: Identifying thresholds for classifying childhood psychiatric disorder: issues and prospects. J Am Acad Child Adolesc Psychiatry 1996; 35:1440–1448Crossref, Medline, Google Scholar
18. Boyle MH, Offord DR, Racine Y, Fleming JE, Szatmari P, Sanford M: Evaluation of the revised Ontario Child Health Study scales. J Child Psychol Psychiatry 1993; 34:189–213Crossref, Medline, Google Scholar
19. Logan GD: On the ability to inhibit thought and action: a user’s guide to the stop signal paradigm, in Inhibitory Processes in Attention, Memory, and Language. Edited by Dagenbach D, Carr TH. San Diego, Academic Press, 1994, pp 189–239Google Scholar
20. SPSS 8.0 for Windows. Chicago, SPSS, 1997Google Scholar
21. Ernst M, Moolchan ET, Robinson ML: Behavioral and neural consequences of prenatal exposure to nicotine. J Am Acad Child Adolesc Psychiatry 2001; 40:630–641Crossref, Medline, Google Scholar
22. Max JE, Lindgren SD, Knutson C, Pearson CS, Ihrig D, Welborn A: Child and adolescent traumatic brain injury: correlates of injury severity. Brain Inj 1998; 12:31–40Crossref, Medline, Google Scholar
23. Buka SL, Goldstein JM, Seidman LJ, Tsuang MT: Maternal recall of pregnancy history: accuracy and bias in schizophrenia research. Schizophr Bull 2000; 26:335–350Crossref, Medline, Google Scholar
24. Thapar A, Harrington R, Ross K, McGuffin P: Does the definition of ADHD affect heritability? J Am Acad Child Adolesc Psychiatry 2000; 39:1528–1536Crossref, Medline, Google Scholar
25. Slaats-Willemse D, Swaab-Barneveld H, de Sonneville L, van der Meulen E, Buitelaar J: Deficient response inhibition as a cognitive endophenotype of ADHD. J Am Acad Child Adolesc Psychiatry 2003; 42:1242–1248Crossref, Medline, Google Scholar
26. Seidman LJ, Biederman J, Monuteaux MC, Weber W, Faraone SV: Neuropsychological functioning in nonreferred siblings of children with attention deficit/hyperactivity disorder. J Abnorm Psychol 2000; 109:252–265Crossref, Medline, Google Scholar
27. Finkel D, McGue M: Age differences in the nature and origin of individual differences in memory: a behavior genetic analysis. Int J Aging Hum Dev 1998; 47:217–239Crossref, Medline, Google Scholar
28. Swan GE, Carmelli D, Reed T, Harshfield GA, Fabsitz RR, Eslinger PJ: Heritability of cognitive performance in aging twins: the National Heart, Lung, and Blood Institute Twin Study. Arch Neurol 1990; 47:259–262Crossref, Medline, Google Scholar
29. Bartfai A, Pedersen NL, Asarnow RF, Schalling D: Genetic factors for the Span of Apprehension Test: a study of normal twins. Psychiatry Res 1991; 38:115–124Crossref, Medline, Google Scholar
30. Bishop DV, Bishop SJ, Bright P, James C, Delaney T, Tallal P: Different origin of auditory and phonological processing problems in children with language impairment: evidence from a twin study. J Speech Lang Hear Res 1999; 42:155–168Crossref, Medline, Google Scholar