Pharmacological Modulation of Prefrontal Cortical Activity During a Working Memory Task in Young and Older Humans: A PET Study With Physostigmine
Abstract
OBJECTIVE: Age-associated cholinergic dysfunction may contribute to the cognitive decline observed during aging, including a decline in working memory. The current study was designed to determine how healthy aging influences the neural response to working memory before and during pharmacological potentiation of the cholinergic system. METHOD: In 13 young and 13 older healthy volunteers, regional cerebral blood flow (rCBF) was measured by using [15O]H2O and positron emission tomography across 10 scans that alternated between a working-memory-for-faces task and rest. In all subjects, the first two scans were obtained during intravenous saline infusion. Seven young and eight older subjects then received intravenous infusion of physostigmine, a cholinesterase inhibitor, and the remaining six young and five older subjects continued to receive saline. RESULTS: In the placebo condition, task-specific rCBF increases in prefrontal regions were observed in the right middle and inferior frontal cortices in young subjects and in more anterior and ventral prefrontal regions in older individuals. Physostigmine during the working memory task significantly improved performance in both age groups. The right prefrontal regions that were selectively recruited in each age group during the placebo condition showed significantly lower rCBF during physostigmine administration. CONCLUSIONS: Cholinergic enhancement does not affect structurally defined cortical regions but rather modulates neural activity in functionally defined regions, that is, in task-related prefrontal cortical areas that are selectively and distinctively recruited in young and older subjects.
Working memory refers to a cognitive process that temporarily maintains an active representation of information for further processing or recall (1, 2). Visual working memory utilizes a widely distributed neural system that includes occipital and temporal visual processing areas and critically relies on regions in the prefrontal cortex for maintaining and manipulating information (3–6).
Given the pivotal role of the cholinergic system in modulating memory and attention (7), in previous studies we examined the effects of pharmacological potentiation of cholinergic activity on behavioral and neural responses during a task that tested visual working memory for faces in young healthy adults. We showed that cholinergic enhancement decreased task-related neural activity in the right prefrontal cortex (8). Moreover, cholinergic enhancement modulated much of the working memory system, including the anterior cingulate, hippocampus, and visual processing areas in occipitotemporal cortex, in a way that correlated with improvement in task performance (9). A subsequent study demonstrated that cholinergic enhancement selectively increased the processing of task-relevant information in visual cortex (10). Together, these findings suggest that the reduction of activity in the prefrontal cortex may be due to an indirect effect, as the enhanced processing in visual regions resulted in an improved representation of the visual percept that in turn facilitated working memory and thus diminished the need to recruit prefrontal cortical areas.
Aging is associated with anatomical, chemical, and functional changes in the brain (11–13). Among the most prominent changes are alterations in the cholinergic system, including decreases in the number of cholinergic neurons in the basal forebrain and in the number of cholinergic receptors and afferent projections to cortex (14–18). Although their significance has been questioned by some researchers (19, 20), these changes may contribute to age-associated deficits observed in working memory, attention, and other cognitive tasks (7, 11, 14, 15, 21–23). Indeed, the pharmacological potentiation of cholinergic neurotransmission improves performance on working memory tasks in elderly subjects (21, 24). Furthermore, chronic treatment with drugs that enhance the cholinergic system is used to ameliorate cognitive dysfunction (25, 26). Despite the well-documented clinical effects of cholinergic enhancement in elderly subjects, the underlying neural mechanisms remain to be elucidated.
Functional brain imaging studies have shown that different patterns of neural responses are elicited by the same visual working memory task in young and older individuals (27–29). These differences include reduced neural activity in the dorsolateral prefrontal cortex, a critical working memory region (28, 29), accompanied by increased activity in other prefrontal cortical areas (30) in older individuals, compared to younger individuals. These observations suggest that the aging brain undergoes a functional reorganization in an attempt to compensate for age-associated regional dysfunction (13, 22, 28, 30–33).
The present study was designed to determine how healthy aging influences neural responses to cholinergic potentiation during working memory. Given that enhancement of cholinergic activity causes a reduced involvement of the right prefrontal cortex in young subjects and that different prefrontal cortical areas are recruited during working memory in elderly subjects, we predicted that the pharmacological potentiation of cholinergic transmission would modulate selectively those prefrontal cortical regions differentially recruited in the two age groups. This prediction assumes that the reduction in right prefrontal cortical activity reflects a reduction in the need to recruit prefrontal areas, perhaps because of improved processing in visual cortical regions.
Method
Subjects
Thirteen young (six male, seven female; mean age=27 years, SD=6) and 13 older (eight male, five female; mean age=65, SD=11) healthy volunteers were included in the study. All were right-handed and normotensive and had no abnormalities on clinical examinations and on laboratory tests (including routine blood and urine tests, EKG, EEG, brain magnetic resonance imaging scan, and chest X-ray) and no history of any relevant medical, neurological, or psychiatric disorder. All were free of medication, including over-the-counter medications, for 4 weeks before the study (34). Written informed consent was obtained from all subjects before their participation in the study. The study was approved by the National Institutes of Health (NIH) Intramural Review Board (NIH protocol 93-AG-193).
Positron Emission Tomography Scan and Working Memory Task
Absolute regional cerebral blood flow (rCBF) was measured by using [15O]H2O and a Scanditronix PC2048–15B positron emission tomography (PET) scanner (Uppsala, Sweden) (full width at half maximum: 6.5 mm) at the PET Department of the Clinical Center at NIH in Bethesda, Maryland. Ten 4-minute scans were acquired; they alternated between a rest condition (awake subjects with their eyes open and ears unplugged in a dimly lit room) and a visual working-memory-for-faces task (Figure 1). Arterial blood sampling through the radial artery was initiated at time of injection of [15O]H2O and continued throughout each of the 4-minute scans (8, 35). Visual stimuli were presented in three squares of equal size, one centered above two that were positioned side by side. Each item began with a 4-second presentation of a novel face in the upper square, followed by a 6-second delay, and then by two test faces presented for 4 seconds in each of the lower two squares. Subjects were instructed to indicate which test face matched the face presented at the beginning of each trial by pressing a button in the corresponding hand. All subjects were unaware of whether they would receive placebo or drug. For all subjects, the first scan pair (including one rest and one task scan) was obtained during an intravenous infusion of saline (placebo condition). Subsequently, seven young subjects and eight older subjects received an intravenous loading dose of physostigmine before the third scan at a rate of 1.93 mg/hour for 10 minutes, followed by a maintenance solution that continued to completion of the study at the rate of 0.816 mg/hour for a total dose of 1 mg/hour of physostigmine (drug condition) (36). Before the infusion of physostigmine, 0.2 mg of glycopyrrolate, a peripheral cholinergic antagonist, was administered intravenously to reduce the potential for side effects (37). The remaining six young subjects and five older subjects continued to receive an infusion of saline (placebo groups). Heart rate and blood pressure were monitored continuously throughout each study (8).
Performance Data Analysis
Accuracy and reaction time data were analyzed in a repeated-measures two-way analysis of variance (ANOVA) (repeated reaction time/[accuracy by age group by infusion condition]). T tests were used to evaluate age-group differences at baseline. Drug effects on reaction time were assessed by using one-tailed tests on the basis of previously reported findings (8–10).
PET-rCBF Data Analysis
Using SPM 99 (Wellcome Department of Cognitive Neurology, Institute of Neurology, University College, London) (www.fil.ion. ucl.ac.uk/spm), PET data were registered to correct for between-scan movements; spatially normalized to the orientation, size, and shape specified in the brain atlas of Talairach and Tournoux (38); and smoothed using a 12×12×12 mm Gaussian filter.
The first scan pair (scans 1 and 2) was used to assess differences between the placebo and drug groups within each age group before drug administration, both at rest and during the working memory task. Values for rCBF at rest during the drug and placebo conditions were compared to determine any potential effect of physostigmine on rCBF at rest (8). Furthermore, to control for potential effects of multiple task repetition, the same analyses were performed within the two placebo groups that received saline throughout the examination (8).
Brain Response to Working Memory Task Before and During Physostigmine Administration
Brain regions showing rCBF increases during the task (scans 4, 6, 8, and 10), relative to rest (scans 3, 5, 7, and 9), were identified through pairwise t test comparisons separately in the young and old age groups. Brain regions with a significant (individual voxel level of p<0.05) rCBF change during the task, compared to rest, were identified across groups, and these regions were used to restrict the search volumes in all subsequent analyses for age group and/or drug interaction effects.
By contrasting task scans with rest scans within each drug group, brain regions that showed rCBF increases or decreases with the working memory task during drug administration were determined in the young subjects and the older subjects.
Statistical significance was assumed at an individual voxel level of p<0.01 and required cluster-level significance of p<0.05 uncorrected. Results that approached significance are reported only when individual voxels were significant at a p<0.01 level, but the correction for spatial extent was p<0.10.
Although these statistical thresholds may appear relatively less stringent, all the experiments were based on specific hypotheses from previous studies (8–10), and therefore a whole-brain correction would have been excessively conservative.
Task-by-drug interactions were identified within each age group by contrasting regions with increased or decreased rCBF during the task in the placebo and drug groups. Drug effects within each age group also were determined by contrasting task activations during drug administration (scans 3–10) with rCBF measures acquired during the first scan pair (during placebo infusion). In these contrasts, t tests were used to confirm differences in drug effects between the placebo and drug groups.
Effects of Age
Interaction of age group and task during placebo and physostigmine administration
Interactions of age group and task were identified by contrasting the regions showing significant rCBF changes during the task between the young and old age groups. For all two-way ANOVA interactions, statistical significance was assumed at an individual voxel level of p<0.05 and required 100 contiguous significant voxels.
Interaction of age group, task, and drug
Task-by-drug-by-age interactions were identified by contrasting regions with rCBF changes during the task between the placebo and drug groups and between the young and old age groups. For all three-way ANOVA interactions, statistical significance was assumed at an individual voxel level of p<0.05 and required 50 contiguous significant voxels.
Task-associated rCBF decreases during placebo and physostigmine administration
Brain regions showing rCBF decreases during the task (scans 4, 6, 8, and 10), relative to rest (scans 3, 5, 7, and 9), were identified through t test analyses separately in young and old age groups.
Results
Behavioral Results
Performance accuracy did not differ between young and older subjects in the placebo condition (young subjects: mean=97.2%, SD=4.5%; older subjects: mean=97.9%, SD=3.0%), and physostigmine administration had no significant effect on performance accuracy (young subjects: mean=97.4%, SD=1.6%; older subjects: mean=94.3%, SD=4.6%). During placebo infusion, reaction times in young subjects were significantly shorter than in older subjects at baseline (young subjects: mean=1374 msec, SD=327; older subjects: mean=1650 msec, SD=408) (t=1.9, df=24, p<0.03). Physostigmine administration significantly decreased the reaction time across all subjects (F=3.8, df=4, 88, p<0.01); the effect of physostigmine on this reduction did not differ between the two age groups (F=0.5, df=4, 88, p=0.75) (Figure 2).
Regional Cerebral Blood Flow
Significant increases and decreases in rCBF during the working memory task during placebo and physostigmine infusion in young and older subjects are summarized in Table 1. Task-associated rCBF increases were superimposed on the right hemisphere of a brain template and on four coronal sections selected to illustrate the findings in the prefrontal cortex for young and older groups (Figure 3).
No difference was observed in the drug groups in resting state rCBF (for main effects of group [drug versus placebo] and time [pre- versus postinfusion] and for their interaction). No significant change in rCBF was observed in the placebo group with comparable within- and between-group subtractions.
Brain response to working memory task before and during physostigmine administration
Young subjects
During placebo infusion, task-specific rCBF increases were observed in the right dorsolateral prefrontal cortex, with loci in the middle frontal gyrus and inferior frontal gyrus/insula, and in the occipital and temporal visual extrastriate regions (Table 1, Figure 3, and Figure 4). During physostigmine infusion, rCBF increases were observed in several loci in the right prefrontal cortex, including the middle frontal gyrus, in the inferior frontal gyrus/insula, and in the occipital and temporal visual extrastriate regions (Table 1 and Figure 3). Significantly lower task-specific rCBF during physostigmine infusion, compared to placebo infusion, was present in the right prefrontal cortex, with loci in dorsal middle frontal gyrus and inferior frontal gyrus/insula, as well as in the occipital and temporal visual regions (Table 1, Figure 3, and Figure 4)
Older subjects
During placebo infusion, task-specific rCBF increases were seen in the right dorsolateral prefrontal cortex, including the right middle frontal gyrus and inferior frontal gyrus/insula, and in the occipital and temporal visual extrastriate regions, similar to the pattern shown by the young group (Table 1, Figure 3, and Figure 4). However, the older subjects also had rCBF increases that were not present in the young group; these increases were located in more anterior and ventral loci of middle and inferior frontal gyri (Table 1). In addition, an increase that approached significance was found in the inferior frontal gyrus in the left hemisphere (p=0.051, cluster level). During physostigmine administration, rCBF increases were observed in the occipital and temporal visual extrastriate regions (Table 1 and Figure 3). No region in the right prefrontal cortex showed significant task-related rCBF increases during physostigmine administration. Brain regions showing significantly less activity during physostigmine infusion, compared to placebo infusion, comprised loci in the right prefrontal cortex that were more anterior and more ventral than the regions modulated by the drug in young subjects. Reduced rCBF also was seen in the ventral temporal cortex during drug administration (Table 1, Figure 3, and Figure 4)
Within-group comparisons
Within the young and older groups that received physostigmine, rCBF during the working memory task was compared between the scans during drug infusion (scans 3 to 10) and the scans during placebo infusion (scans 1 and 2). These comparisons confirmed the differences found in the right prefrontal cortex and in the ventral temporal cortex in the between-group (drug versus placebo) analyses (data not shown).
Effects of Age
Interaction of age group and task during placebo and physostigmine administration
During placebo infusion, larger increases in rCBF in response to the working memory task were observed in the insular cortex and in the ventral temporal cortex in the young subjects, compared to the older subjects. Conversely, the older subjects had significantly larger task-specific rCBF increases in the right ventral and left inferior frontal gyri and in the ventral occipital cortex.
During physostigmine administration, young subjects, compared to older subjects, showed greater activation during the working memory task in the right inferior frontal cortex and in the right ventral temporal cortex (Table 1). Older subjects showed greater activation in the left inferior frontal cortex and in the right occipital and left ventral temporal cortices, compared to the younger group.
Significantly larger rCBF increases during task and physostigmine administration, relative to placebo administration, were observed only in older subjects in the bilateral medial occipital cortex (Talairach coordinates: x=–3, y=–81, z=–19 [z=5.26, p<0.01]; x=8, y=–96, z=–2 [z=3.92, p<0.01]).
Interaction of age group, task, and drug
When the effects of physostigmine administration on the brain response to working memory were compared directly between young and older subjects, physostigmine significantly decreased task-related activations in the right inferior and middle frontal gyri and in the right anterior ventral temporal cortex to a greater extent in the older subjects than in the younger subjects. In addition, physostigmine reduced the magnitude of task-related response in the ventral occipital and temporal regions and in the right posterior ventral temporal cortices significantly less in the older subjects than in the younger subjects (Table 1).
Task-associated rCBF decreases during placebo and physostigmine administration
During placebo administration, rCBF decreases during the working memory task, compared to rest, were observed in some frontal and temporal cortical regions and limbic structures, as detailed in Table 1. Physostigmine administration did not change significantly the pattern of task-related rCBF reductions observed before drug administration, with the exception of a greater reduction in the left insula in young subjects and in the medial frontal gyrus in older subjects (data not shown).
Discussion
Cholinergic potentiation induced by acute intravenous administration of the anticholinesterase physostigmine modulated neural activity throughout the distributed visual working memory system, including both the prefrontal cortex and the visual processing areas, and improved task performance similarly in young and older healthy individuals. In the two age groups, some brain regions were commonly modulated and some areas were differentially affected by cholinergic potentiation. Specifically, in the presence of similar behavioral improvement, cholinergic enhancement modulated selectively the prefrontal cortical regions that were recruited differentially by young and older individuals during a working memory task before drug administration.
During placebo administration, young subjects activated more dorsal regions of the right prefrontal cortex, and older subjects recruited more anterior and ventral regions and areas in the left prefrontal cortex. This pattern, including the involvement of the ventrolateral and left prefrontal cortices, is consistent with previous findings and further supports the hypothesis that the recruitment of additional prefrontal cortical areas during working memory and other cognitive tasks in elderly subjects may reflect a functional reorganization to compensate for age-associated changes in neural function (13, 22, 28, 30, 33).
During the working memory task, in the presence of the drug, compared with placebo, the younger subjects showed reduced activation in the dorsal middle frontal and inferior frontal gyri, and the older subjects had reduced activation in the right prefrontal cortex, with loci that were more anterior and more ventral than in the young subjects.
Previously, we demonstrated that cholinergic potentiation with physostigmine improved working memory efficiency and reduced task-related neural activity in the right prefrontal cortex and that the degree of improvement correlated with the magnitude of reduction in prefrontal cortical activity (8). Physostigmine also modulated neural processing in a manner that correlated with behavioral improvement in multiple task-related cortical areas, including visual cortical regions (9, 10). Overall, these results suggest that cholinergic potentiation enhances memory performance by augmenting attention in perceptual representation of the visual cortex, thereby improving information processing efficiency and reducing the need for prefrontal cortical participation (10). Consistent with and further supporting this hypothesis (8–10), in the current study cholinergic enhancement modulated neural activity in visual cortical areas and reduced prefrontal cortical involvement in both young and older individuals.
Other studies that used different cognitive tasks have demonstrated that the functional recruitment of the right prefrontal cortex is proportional to the effort required to perform the task (35, 39, 40). For instance, recognition of progressively degraded faces was associated with a parallel rCBF increase in the right prefrontal cortex in young healthy subjects (35). The physostigmine-associated decrease in the neural response in the right prefrontal cortex may be secondary to a reduction in the effort needed to perform the working memory task, consistent with the observed improvement in task performance. The relation between cholinergic function, prefrontal cortical activity, and cognitive effort might be further investigated by varying the difficulty of the working memory task, such as by modifying visuoperceptual integrity (35) or modulating the length of the working memory retention interval (39, 41).
The cholinergic system undergoes structural and functional changes associated with cognitive impairment in elderly persons (21, 25, 42) and more severe changes in patients with Alzheimer’s disease. Indeed, cholinergic enhancement strategies remain a primary approach for treating cognitive dysfunction in Alzheimer’s disease. Our results suggest that, despite age-associated changes in the cholinergic system, the mechanism by which cholinergic enhancement modulates working memory is similar in younger and older individuals. However, our results also suggest the critical finding that the effects of cholinergic enhancement on prefrontal cortical activity are not specific for structurally defined cortical regions but rather that they affect functionally defined regions, that is, the task-related prefrontal cortical areas that are selectively recruited by younger and older subjects.
To our knowledge, this study provides the first demonstration of a selective age-dependent effect of cholinergic modulation on prefrontal cortical neural activity during working memory.
The results of this study may have important general implications, as they suggest that structural and functional brain changes that take place during the physiological aging process shape the way a given pharmacological agent interacts with distinct brain structures. As these modifications are far more remarkable in patients affected by neurodegenerative disorders such as Alzheimer’s disease, these results may also provide some novel ground for developing and evaluating new therapeutic agents for these patients.
![]() |
Preliminary data presented at the 6th Annual Meeting of the Organization for Human Brain Mapping, June 12–16, 2000, San Antonio, Tex., and at the 8th National Meeting of the Italian Society for Psychopathology, February 25–March 1, 2003, Rome. Received Aug. 27, 2004; revision received Feb. 15, 2005; accepted March 7, 2005. From the Department of Neurology and Psychiatry and the Department of Anesthesiology, University of Padua, Padua, Italy; the Laboratory of Clinical Biochemistry and Molecular Biology, Department of Experimental Pathology, Medical Biotechnologies, Infectivology, and Epidemiology, University of Pisa, Pisa, Italy; Sant’Anna School of University Studies and Doctoral Research, Pisa, Italy; Children’s Hospital Medical Center, Cincinnati; the Brain Physiology and Metabolism Section, National Institute on Aging, Bethesda, Md.; and the Mood and Anxiety Disorders Program, NIMH, Bethesda, Md. Address correspondence and reprint requests to Dr. Pietrini, Laboratory of Clinical Biochemistry, Medical School, University of Pisa, Via Roma, 55, I-56126 Pisa, Italy; [email protected] (e-mail). Supported by the National Institute on Aging intramural program, the Italian Ministry of Health (SIALPU project), and in part by grants from the GIO.I.A. Foundation (Pisa, Italy) and the I.R.I.S. Foundation (Livorno, Italy). The authors thank P. Herscovitch and the technologists of the NIH positron emission tomography program.
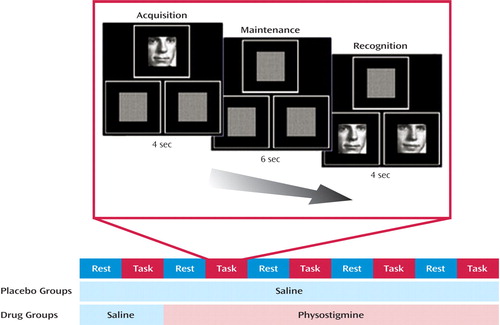
Figure 1. Experimental Paradigm Sequence in a Study of Pharmacological Modulation of the Neural Response to a Visual Working Memory Task in Healthy Younger and Older Subjectsa
aTen 4-minute positron emission tomography scans alternated between a resting condition and a visual working memory (face recognition) task. Saline solution was infused during the first scan pair for both age groups; subsequently, the placebo group in each age group continued to receive saline infusion, while the drug group in each age group received intravenous physostigmine infusion for the duration of the session. During the match-to-sample working memory task, faces were presented in three squares of equal size, one centered above two positioned side by side.
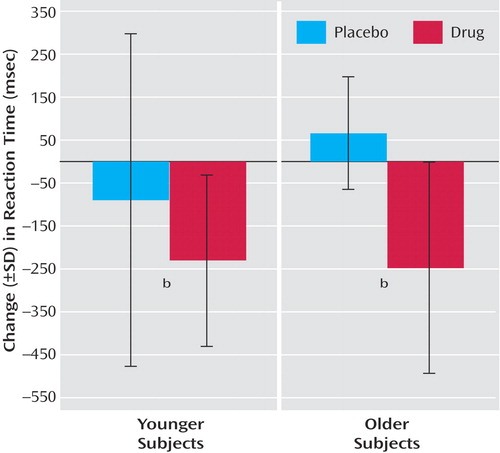
Figure 2. Effects of Physostigmine on Change in Reaction Time on a Visual Working Memory Task in Healthy Younger Subjects (N=13) and Healthy Older Subjects (N=13)a
aThe effect of physostigmine on reaction time was determined by calculating a difference score between reaction time acquired during the last task scan and the first task scan.
bSignificant difference between placebo and drug conditions (p<0.01, t test, one-tailed).
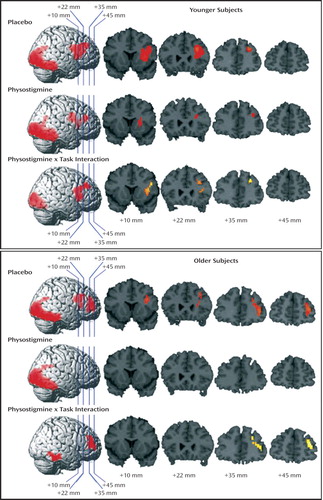
Figure 3. Significant Increases in Regional Cerebral Blood Flow (rCBF) During a Working Memory Task With Placebo and Physostigmine Infusion in Healthy Younger Subjects (N=13) and Healthy Older Subjects (N=13)a
aResults are projected onto the right hemisphere of a brain template, which indicates the locations for the four coronal sections located at 10, 22, 35, and 45 mm anterior to the anterior commissure. Increases for which the z score exceeded a threshold of 2.35 (p=0.01, two-tailed, before correction for multiple comparisons on the basis of spatial extent) are shown.
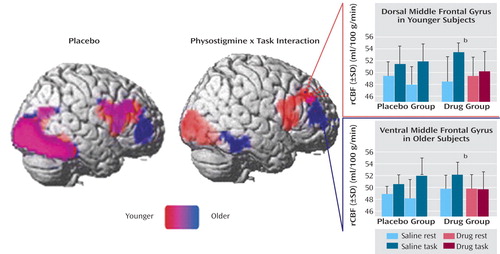
Figure 4. Effects of Physostigmine on Brain Activity During a Visual Working Memory Task in Healthy Younger Subjects (N=13) and Healthy Older Subjects (N=13)a
aBrain regions showing significant rCBF increases during the visual working memory task in younger and older subjects are superimposed onto the right hemisphere of a brain template. The brain template on the left shows cortical areas commonly and distinctively activated in response to the working memory task during saline infusion. The brain template on the right shows brain regions with significant rCBF reductions during the working memory task in the presence of physostigmine, relative to placebo. The graphs show that younger individuals had a significant reduction in the magnitude of the response to the task in the presence of physostigmine, relative to placebo, in middle frontal gyrus (Talairach coordinates: x=22, y=34, z=33); older subjects showed reductions in response magnitude in the presence of physostigmine, relative to placebo, in more ventral and anterior aspects of middle frontal gyrus (Talairach coordinates: x=33, y=41, z=12).
bSignificant difference between rest and task conditions during physostigmine infusion (p<0.05, t test).
1. Baddeley A, Logie R, Bressi S, Della Sala S, Spinnler H: Dementia and working memory. Q J Exp Psychol A 1986; 38:603–618Crossref, Medline, Google Scholar
2. Baddeley AD, Bressi S, Della Sala S, Logie R, Spinnler H: The decline of working memory in Alzheimer’s disease: a longitudinal study. Brain 1991; 114(part 6):2521–2542Google Scholar
3. Haxby JV, Horwitz B, Ungerleider LG, Maisog JM, Pietrini P, Grady CL: The functional organization of human extrastriate cortex: a PET-rCBF study of selective attention to faces and locations. J Neurosci 1994; 14(11, part 1):6336–6353Google Scholar
4. Courtney SM, Ungerleider LG, Keil K, Haxby JV: Object and spatial visual working memory activate separate neural systems in human cortex. Cereb Cortex 1996; 6:39–49Crossref, Medline, Google Scholar
5. McCarthy G, Blamire AM, Puce A, Nobre AC, Bloch G, Hyder F, Goldman-Rakic P, Shulman RG: Functional magnetic resonance imaging of human prefrontal cortex activation during a spatial working memory task. Proc Natl Acad Sci USA 1994; 91:8690–8694Crossref, Medline, Google Scholar
6. Petrides M, Alivisatos B, Meyer E, Evans AC: Functional activation of the human frontal cortex during the performance of verbal working memory tasks. Proc Natl Acad Sci USA 1993; 90:878–882Crossref, Medline, Google Scholar
7. Everitt BJ, Robbins TW: Central cholinergic systems and cognition. Annu Rev Psychol 1997; 48:649–684Crossref, Medline, Google Scholar
8. Furey ML, Pietrini P, Haxby JV, Alexander GE, Lee HC, VanMeter J, Grady CL, Shetty U, Rapoport SI, Schapiro MB, Freo U: Cholinergic stimulation alters performance and task-specific regional cerebral blood flow during working memory. Proc Natl Acad Sci USA 1997; 94:6512–6516Crossref, Medline, Google Scholar
9. Furey ML, Pietrini P, Alexander GE, Schapiro MB, Horwitz B: Cholinergic enhancement improves performance on working memory by modulating the functional activity in distinct brain regions: a positron emission tomography regional cerebral blood flow study in healthy humans. Brain Res Bull 2000; 51:213–218Crossref, Medline, Google Scholar
10. Furey ML, Pietrini P, Haxby JV: Cholinergic enhancement and increased selectivity of perceptual processing during working memory. Science 2000; 290:2315–2319Crossref, Medline, Google Scholar
11. Creasey H, Rapoport SI: The aging human brain. Ann Neurol 1985; 17:2–10Crossref, Medline, Google Scholar
12. Park D: The basic mechanism accounting for age-related decline in cognitive function, in Aging and Cognition: A Primer. Edited by Park D. Philadelphia, Psychology Press, 2000, pp 3–21Google Scholar
13. Pietrini P, Rapoport SI: Functional brain imaging: cerebral blood flow and glucose metabolism in healthy human aging, in Textbook of Geriatric Neuropsychiatry, vol 2. Edited by Coffey CE, Cumming JL. Washington, DC, American Psychiatric Press, 2000, pp 239–265Google Scholar
14. Gibson GE, Peterson C, Jenden DJ: Brain acetylcholine synthesis declines with senescence. Science 1981; 213:674–676Crossref, Medline, Google Scholar
15. Altavista MC, Rossi P, Bentivoglio AR, Crociani P, Albanese A: Aging is associated with a diffuse impairment of forebrain cholinergic neurons. Brain Res 1990; 508:51–59Crossref, Medline, Google Scholar
16. Mihailescu S, Drucker-Colin R: Nicotine, brain nicotinic receptors, and neuropsychiatric disorders. Arch Med Res 2000; 31:131–144Crossref, Medline, Google Scholar
17. Geula C, Mesulam MM: Cortical cholinergic fibers in aging and Alzheimer’s disease: a morphometric study. Neuroscience 1989; 33:469–481Crossref, Medline, Google Scholar
18. Mesulam M: The cholinergic lesion of Alzheimer’s disease: pivotal factor or side show? Learn Mem 2004; 11:43–49Crossref, Medline, Google Scholar
19. DeKosky ST, Ikonomovic MD, Styren SD, Beckett L, Wisniewski S, Bennett DA, Cochran EJ, Kordower JH, Mufson EJ: Upregulation of choline acetyltransferase activity in hippocampus and frontal cortex of elderly subjects with mild cognitive impairment. Ann Neurol 2002; 51:145–155Crossref, Medline, Google Scholar
20. Davis KL, Mohs RC, Marin D, Purohit DP, Perl DP, Lantz M, Austin G, Haroutunian V: Cholinergic markers in elderly patients with early signs of Alzheimer disease. JAMA 1999; 281:1401–1406Crossref, Medline, Google Scholar
21. Bartus RT, Dean RL III, Beer B, Lippa AS: The cholinergic hypothesis of geriatric memory dysfunction. Science 1982; 217:408–414Crossref, Medline, Google Scholar
22. Grady CL, Craik FI: Changes in memory processing with age. Curr Opin Neurobiol 2000; 10:224–231Crossref, Medline, Google Scholar
23. Li SC, Lindenberger U, Sikstrom S: Aging cognition: from neuromodulation to representation. Trends Cogn Sci 2001; 5:479–486Crossref, Medline, Google Scholar
24. Drachman DA, Sahakian BJ: Memory and cognitive function in the elderly: a preliminary trial of physostigmine. Arch Neurol 1980; 37:674–675Crossref, Medline, Google Scholar
25. Bartus RT: On neurodegenerative diseases, models, and treatment strategies: lessons learned and lessons forgotten a generation following the cholinergic hypothesis. Exp Neurol 2000; 163:495–529Crossref, Medline, Google Scholar
26. Terry AV Jr, Buccafusco JJ: The cholinergic hypothesis of age and Alzheimer’s disease-related cognitive deficits: recent challenges and their implications for novel drug development. J Pharmacol Exp Ther 2003; 306:821–827Crossref, Medline, Google Scholar
27. Zacks RT, Hasher L, Li KZH: Human memory, in Handbook of Aging and Cognition. Edited by Craik FIM, Salthouse TA. Mahwah, NJ, Lawrence Erlbaum Associates, 2000, pp 293–357Google Scholar
28. Rypma B, Prabhakaran V, Desmond JE, Gabrieli JD: Age differences in prefrontal cortical activity in working memory. Psychol Aging 2001; 16:371–384Crossref, Medline, Google Scholar
29. Rypma B, D’Esposito M: Isolating the neural mechanisms of age-related changes in human working memory. Nat Neurosci 2000; 3:509–515Crossref, Medline, Google Scholar
30. Cabeza R: Hemispheric asymmetry reduction in older adults: the HAROLD model. Psychol Aging 2002; 17:85–100Crossref, Medline, Google Scholar
31. Grady CL, McIntosh AR, Horwitz B, Maisog JM, Ungerleider LG, Mentis MJ, Pietrini P, Schapiro MB, Haxby JV: Age-related reductions in human recognition memory due to impaired encoding. Science 1995; 269:218–221Crossref, Medline, Google Scholar
32. Pietrini P, Alexander GE, Furey ML, Hampel H, Guazzelli M: The neurometabolic landscape of cognitive decline: in vivo studies with positron emission tomography in Alzheimer’s disease. Int J Psychophysiol 2000; 37:87–98Crossref, Medline, Google Scholar
33. Cabeza R, Anderson ND, Locantore JK, McIntosh AR: Aging gracefully: compensatory brain activity in high-performing older adults. Neuroimage 2002; 17:1394–1402Crossref, Medline, Google Scholar
34. Pietrini P, Furey ML, Graff-Radford N, Freo U, Alexander GE, Grady CL, Dani A, Mentis MJ, Schapiro MB: Preferential metabolic involvement of visual cortical areas in a subtype of Alzheimer’s disease: clinical implications. Am J Psychiatry 1996; 153:1261–1268Link, Google Scholar
35. Grady CL, Horwitz B, Pietrini P, Mentis MJ, Ungerleider LG, Rapoport SI, Haxby JV: Effect of task difficulty on cerebral blood flow during perceptual matching of faces. Hum Brain Mapp 1996; 4:227–239Crossref, Medline, Google Scholar
36. Furey ML, Pietrini P, Alexander GE, Mentis MJ, Szczepanik J, Shetty U, Greig NH, Holloway HW, Schapiro MB, Freo U: Time course of pharmacodynamic and pharmacokinetic effects of physostigmine assessed by functional brain imaging in humans. Pharmacol Biochem Behav 2000; 66:475–481Crossref, Medline, Google Scholar
37. Oduro KA: Glycopyrrolate methobromide, 2: comparison with atropine sulphate in anaesthesia. Can Anaesth Soc J 1975; 22:466–473Crossref, Medline, Google Scholar
38. Talairach J, Tournoux P: Co-Planar Stereotaxic Atlas of the Human Brain: Three-Dimensional Proportional System. New York, Thieme Medical, 1988Google Scholar
39. Haxby JV, Ungerleider LG, Horwitz B, Rapoport SI, Grady CL: Hemispheric differences in neural systems for face working memory: a PET-rCBF study. Hum Brain Mapp 1995; 3:68–82Crossref, Google Scholar
40. Koechlin E, Corrado G, Pietrini P, Grafman J: Dissociating the role of the medial and lateral anterior prefrontal cortex in human planning. Proc Natl Acad Sci USA 2000; 97:7651–7656Crossref, Medline, Google Scholar
41. Grady CL, McIntosh AR, Bookstein F, Horwitz B, Rapoport SI, Haxby JV: Age-related changes in regional cerebral blood flow during working memory for faces. Neuroimage 1998; 8:409–425Crossref, Medline, Google Scholar
42. Drachman DA, Leavitt J: Human memory and the cholinergic system: a relationship to aging? Arch Neurol 1974; 30:113–121Crossref, Medline, Google Scholar