Toward a Rapidly Acting Antidepressant: The Normetanephrine and Extraneuronal Monoamine Transporter (Uptake 2) Hypothesis
Abstract
OBJECTIVE: The authors considered the possible role of the extraneuronal monoamine transporter (uptake 2) in accounting for the delay in clinical action of norepinephrine reuptake inhibitor antidepressant drugs. METHOD: Literature searches were performed by means of the MEDLINE and Current Contents databases with search terms such as “extraneuronal uptake,” “uptake 2,” “extraneuronal monoamine transporter,” and “organic cation transporter type-3.” RESULTS: The findings in this literature indicate that inhibition of glial uptake 2 by normetanephrine or other inhibitors of uptake 2 would enhance the accumulation of norepinephrine in the synapse. CONCLUSIONS: The authors propose the hypothesis that drugs or other agents that increase levels of normetanephrine or otherwise inhibit the extraneuronal monoamine transporter, uptake 2, in the brain will speed up the clinical effects of norepinephrine reuptake inhibitor antidepressant drugs.
A major problem in psychiatry is how to account for the delay (about 2–6 weeks) between the initial administration of norepinephrine reuptake inhibitor antidepressant drugs and the onset of clinical antidepressant effects. In studies involving both animals and depressed patients, we and other investigators have observed a gradual increase in the release of norepinephrine and the output of its O-methylated metabolite, normetanephrine, during long-term (e.g., starting around 3 weeks) but not short-term (e.g., less than 1 week) administration of tricyclic antidepressant drugs (1–4 and our unpublished data). Moreover, a gradual increase in urinary normetanephrine occurred during the period of clinical improvement (determined by a gradual and persistent decrease in Hamilton Depression Rating Scale scores) in a group of depressed patients who responded to the tricyclic antidepressant drug imipramine (1).
The in vitro pharmacological effects of normetanephrine (5) and the rapid in vivo metabolic inactivation of normetanephrine in the brain (6) both suggest that normetanephrine possesses intrinsic biological activity. However, the potential pharmacological effects of normetanephrine have not been widely explored. Understanding the physiological mechanisms that contribute to this gradual increase in the release and metabolism (O-methylation) of norepinephrine to normetanephrine during the administration of tricyclic antidepressant drugs might provide insights concerning the development of procedures to speed up the clinical antidepressant effects of these drugs (7).
Mechanisms of Noradrenergic Transmission
We performed an exhaustive review of specific aspects of the literature on noradrenergic neurotransmission by searching the MEDLINE and Current Contents databases. “Extraneuronal uptake,” “uptake 2,” “extraneuronal monoamine transporter,” “organic cation transporter type-3,” and similar phrases were used as search terms.
Changes in synaptic noradrenergic neurotransmission during treatment with noradrenergic reuptake inhibitors are depicted in Figure 1. The major mechanism for the inactivation of norepinephrine that is released into the synapse is reuptake of the released norepinephrine into the presynaptic neuron of origin, i.e., so-called uptake 1, which is blocked by norepinephrine reuptake inhibitor tricyclic antidepressant drugs (2, 8). Inhibition of presynaptic neuronal reuptake of norepinephrine (uptake 1) occurs quickly and initially produces an increase of norepinephrine at the synapse—compare part B with part A in Figure 1. But this increase is brief because it leads to the activation of α2-adrenergic receptors on noradrenergic nerve terminals and on cell bodies in the locus coeruleus, resulting in a decrease of locus coeruleus firing rates and a decreased release of norepinephrine from presynaptic noradrenergic neurons—compare part C with part B in Figure 1(9–11).
However, during continued administration of norepinephrine reuptake inhibitor antidepressants, there is a progressive decrease in the sensitivity (11) and/or possibly a blockade (12) of the α2-adrenergic receptors, leading to an increase in locus coeruleus firing rates and an increased release of norepinephrine from presynaptic neurons, thereby increasing synaptic levels of norepinephrine—compare part D with part C in Figure 1(3, 11)—and presumably promoting clinical antidepressant effects.
In the last several years, however, glia have been recognized to be actively involved in the process of synaptic transmission in the central nervous system (13, 14), and norepinephrine present in the synapse or other extraneuronal spaces can also be taken up into adjacent glia by a mechanism known as uptake 2, the extraneuronal monoamine transporter (5, 15–18). In glia, norepinephrine is converted by catechol O-methyltransferase (COMT) to normetanephrine, which is a potent inhibitor of uptake 2 (5, 19). Thus, the formation of normetanephrine following the uptake of norepinephrine by the extraneuronal monoamine transporter, uptake 2, inhibits further uptake of norepinephrine by uptake 2—compare part E with part D in Figure 1—producing a marked increase in levels of norepinephrine in the synapse.
Findings
In a recent study of depressed patients treated with desipramine, the sum of urinary levels of norepinephrine plus normetanephrine, expressed in micrograms per 24 hours, at baseline (mean=278, SD=90) and after 1 week (mean=241, SD=79), 4 weeks (mean=333, SD=97), and 6 weeks (mean=353, SD=125) of treatment was significantly increased over time according to a random effects regression analysis (J. Hennen, personal communication). Compared to baseline values, this sum was slightly decreased after 1 week of treatment but was significantly increased (p<0.01, postmodeling two-tailed t test) after both 4 and 6 weeks of treatment with desipramine.
Moreover, we observed that, when compared to baseline values, the ratio of urinary norepinephrine to normetanephrine was significantly increased (p<0.001, postmodeling two-tailed t test) after 4 and 6 weeks of treatment with desipramine but not after 1 week of treatment (our unpublished data).
These findings are compatible with the hypothesis that normetanephrine is indeed blocking uptake 2, thereby decreasing the access of norepinephrine to COMT and, thus, the inactivation of norepinephrine by enzymatic conversion to normetanephrine—compare part E with part D in Figure 1.
Conclusions
Therefore, we propose the hypothesis that drugs or other agents that increase levels of normetanephrine or otherwise inhibit the extraneuronal monoamine transporter, uptake 2, in the brain will produce a marked increase in levels of norepinephrine in the synapse and thus speed up the clinical effects of norepinephrine reuptake inhibitor antidepressant drugs (7).
Received March 20, 2003; revision received Sept. 16, 2003; accepted Oct. 16, 2003. From the Department of Psychiatry, Harvard Medical School at Massachusetts Mental Health Center. Address reprint requests to Dr. Schildkraut, 35 Jefferson Rd., Chestnut Hill, MA 02467; [email protected] (e-mail).
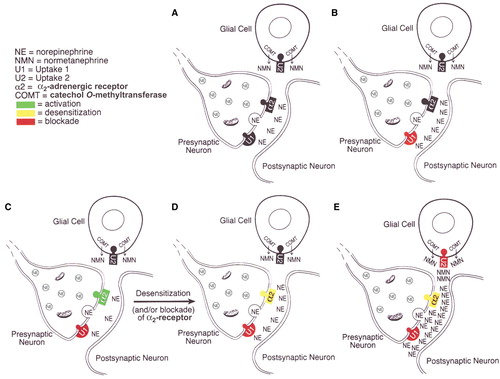
Figure 1. Sequential Changes in the Noradrenergic Synapse During Long-Term Treatment With a Noradrenergic Reuptake Inhibitor, Such as Desipramine
1. Schildkraut JJ, Green R, Gordon EK, Durell J: Normetanephrine excretion and affective state in depressed patients treated with imipramine. Am J Psychiatry 1966; 123:690–700Link, Google Scholar
2. Schildkraut JJ, Dodge GA, Logue MA: Effects of tricyclic antidepressants on the uptake and metabolism of intracisternally administered norepinephrine-H3 in rat brain. J Psychiatr Res 1969; 7:29–34Crossref, Medline, Google Scholar
3. Schildkraut JJ, Winokur A, Applegate CW: Norepinephrine turnover and metabolism in rat brain after long-term administration of imipramine. Science 1970; 168:867–869Crossref, Medline, Google Scholar
4. Schildkraut JJ, Winokur A, Draskóczy PR, Hensle JH: Changes in norepinephrine turnover in rat brain during chronic administration of imipramine and protriptyline: a possible explanation for the delay in onset of clinical antidepressant effects. Am J Psychiatry 1971; 127:1032–1039Link, Google Scholar
5. Burgen ASV, Iversen LL: The inhibition of noradrenaline uptake by sympathomimetic amines in the rat isolated heart. Br J Pharmacol 1965; 25:34–49Google Scholar
6. Schanberg SM, Schildkraut JJ, Breese GR, Kopin IJ: Metabolism of normetanephrine-H3 in rat brain—identification of conjugated 3-methoxy-4-hydrophenylglycol as the major metabolite. Biochem Pharmacol 1968; 17:247–254Crossref, Medline, Google Scholar
7. Schildkraut JJ, Mooney JJ: Antidepressant effect of norepinephrine uptake 2 inhibitors and combined medications including them: US Patent Number 6,403,645, June 11, 2002Google Scholar
8. Glowinski J, Axelrod J: Effects of drugs on the disposition of H3–norepinephrine in the rat brain. Pharmacol Rev 1966; 18:775–785Medline, Google Scholar
9. Nybäck HV, Walters JR, Aghajanian GK, Roth RH: Tricyclic antidepressants: effects on the firing rate of brain noradrenergic neurons. Eur J Pharmacol 1975; 32:302–312Crossref, Medline, Google Scholar
10. Mateo Y, Pineda J, Meana JJ: Somatodendritic α2 adrenoceptors in the locus coeruleus are involved in the in vivo modulation of cortical noradrenaline release by the antidepressant desipramine. J Neurochem 1998; 71:790–798Crossref, Medline, Google Scholar
11. Linnér L, Arborelius L, Nomikos GG, Bertilsson L, Svensson TH: Locus coeruleus neuronal activities and noradrenaline availability in the frontal cortex of rats chronically treated with imipramine: effect of α2–adrenoceptor blockade. Biol Psychiatry 1999; 46:766–774Crossref, Medline, Google Scholar
12. Lenz T, Werle E, Strobel G, Weicker H: O–Methylated and sulfoconjugated catecholamines: differential activities at human platelet α2–adrenoceptors. Can J Physiol Pharmacol 1991; 69:929–937Crossref, Medline, Google Scholar
13. Coyle JT, Schwarcz R: Mind glue: implications of glial cell biology for psychiatry. Arch Gen Psychiatry 2000; 57:90–93Crossref, Medline, Google Scholar
14. Haydon PG: Glia: listening and talking to the synapse. Nat Rev Neurosci 2001; 2:185–193Crossref, Medline, Google Scholar
15. Hendley ED, Taylor KM, Snyder SH: 3H-Normetanephrine uptake in rat brain slices: relationship to extraneuronal accumulation of norepinephrine. Eur J Pharmacol 1970; 12:167–179Crossref, Medline, Google Scholar
16. Henn FA, Hamberger A: Glial cell function: uptake of transmitter substances. Proc Natl Acad Sci USA 1971; 68:2686–2690Crossref, Medline, Google Scholar
17. Streich S, Brüss M, Bönisch H: Expression of the extraneuronal monoamine transporter (uptake2) in human glioma cells. Naunyn Schmiedebergs Arch Pharmacol 1996; 353:328–333Crossref, Medline, Google Scholar
18. Gründemann D, Schechinger B, Rappold GA, Schömig E: Molecular identification of the corticosterone-sensitive extraneuronal catecholamine transporter. Nat Neurosci 1998; 1:349–351Crossref, Medline, Google Scholar
19. Männistö PT, Kaakkola S: Catechol-O–methyltransferase (COMT): biochemistry, molecular biology, pharmacology, and clinical efficacy of the new selective COMT inhibitors. Pharmacol Rev 1999; 51:593–628Medline, Google Scholar