Subcortical Correlates of Craving in Recently Abstinent Alcoholic Patients
Abstract
OBJECTIVE: This study investigated functional cerebral correlates of craving in alcoholic patients and examined the state/trait characteristics of the regional cerebral network implicated in craving. METHOD: Functional magnetic resonance imaging (fMRI) was used to map cerebral response elicited by ethanol odor in 10 male patients with alcohol dependence who had undergone detoxification and 10 matched nonpatients. After 3 weeks, during which the patients underwent standardized behavioral therapy with psychopharmacological intervention, all subjects were studied a second time with fMRI to evaluate the effects of therapy on the functional cerebral correlates of craving. RESULTS: In the alcoholic patients, cue-induced craving before treatment elicited activation primarily in the subcortical-limbic region of the right amygdala/hippocampal area and in the cerebellum. After treatment, activation was found in the superior temporal sulcus, while subcortical or cerebellar participation was no longer present. Comparison subjects showed no comparable amygdala or cerebellar activation during ethanol stimulation and demonstrated no change in activation pattern between measurements. CONCLUSIONS: This investigation points to state-dependent neurobiological correlates of cue-induced craving in alcoholic patients and suggests that these correlates can be influenced by therapeutic interventions. The presence of emotional aspects of craving is suggested by amygdala activation.
Craving is a motivational state associated with a strong desire to consume a drug. Alcohol-related cues have the potential to elicit craving by classic conditioning processes (1). It is currently widely accepted that craving is a multidimensional phenomenon that may vary in quality and intensity across environmental conditions and between individuals. In light of the role craving plays in triggering compulsive drug use and relapse in abstinent patients, there is a need for further investigation of the underlying neurobiological mechanisms. Presentation of a drug-related stimulus has been found to induce craving in addicts (2) and to act as a conditioned stimulus with incentive value in animals and humans.
In rats, olfactory cues associated with ethanol, in contrast to water, reinstated conditioned responses after extinction (3). It is of interest that conditioned auditory cues failed to produce such effects, suggesting that the processes involved may be modality specific. Other animal studies have pointed to the influence of stress in the reinstatement of extinguished alcohol-seeking behavior (4). In alcoholics early in abstinence, memory of and imagery of an alcoholic beverage can induce equally strong craving effects (measured by subjective ratings and level of autonomic arousal) as actual alcohol exposure (5). Furthermore, stressors increase alcohol craving, autonomic changes, and subjective anxiety (6). Patients’ reactions to ethanol olfactory stimuli (cue reactivity) have been well documented: alcohol abusers reacted to the smell of alcoholic drinks with increased salivation and increased heart rate (7, 8). Stormark and colleagues (9) found that a high-potency alcohol odor (from beer) elicited a defense reaction (increased heart rate and skin conductance) in alcoholics. A low-potency alcohol odor (from vodka) was ineffective in eliciting a defense reaction. Therapy was shown to influence autonomic reactivity in response to alcoholic cues but not to influence subjective craving (10).
Recent neuroimaging studies of craving also point to limbic involvement during cue-induced cocaine craving. In particular, areas of activation associated with craving were suggested in the amygdala (11), the anterior cingulate (11), and the dorsolateral prefrontal cortex (12). These regions have extensive connections with each other and are involved in emotion and motivation. Orbitofrontal, insular cortex, and cerebellar activations, as well as increased subjective craving and autonomic indexes, were demonstrated during a cocaine-theme interview (13). The orbitofrontal cortex has been implicated in learning and extinction, suggesting that this area is involved in mediating reward-related behavior (14). The insular cortex is also closely related to the limbic system and is implicated in modulating autonomic responses. An important role of the cerebellum in memory and affect has recently been recognized (15).
The brain states associated with craving may be similar for alcohol and other drugs (16). One study using single photon emission computed tomography (SPECT) found increased blood flow in the right caudate nucleus during alcoholic craving (17). In a positron emission tomography (PET) investigation that used m-chlorophenylpiperazine to induce craving, increases in glucose metabolism were found in various brain regions in both the alcoholic and the comparison group, although subjects’ self-reports indicated no craving effect (18). In that study, alcoholic subjects demonstrated larger activation areas in the cerebellum and posterior cingulate gyrus. Smaller activations were noted in the thalamus, and no activations were observed in the orbital and dorsolateral prefrontal cortex or in the caudate, compared to healthy subjects. The findings suggested that craving may entail a subjective experience that subjects may be aware of but may not always consciously perceive.
To examine the neurobiological substrates of alcohol-induced craving, the present study used functional magnetic resonance imaging (fMRI) during olfactory stimulation with ethanol in alcohol-dependent patients who had recently undergone detoxification and in normal healthy subjects. Another element in our study was to investigate the state/trait characteristics of the regional cerebral network implicated in craving. We hypothesized that ethanol odor would induce craving in alcoholic patients but not in comparison subjects and that the induced craving would be reflected in an emotional response and in limbic (amygdala) activation. We also hypothesized that alcoholic patients’ participation in a 3-week therapeutic intervention would result in reduced craving that was represented by a quantifiable change in fMRI results.
Method
Subjects
The study participants (10 patients and 10 healthy subjects) were male, right-handed, native German speakers. The patients met DSM-IV criteria for alcohol dependence. Their diagnoses were based on a semistructured clinical interview with the German translation of the Semi-Structured Assessment for the Genetics of Alcoholism (19). Written informed consent was obtained from subjects, and the protocol was approved by the institutional review board of the University of Düsseldorf School of Medicine. Patients with any DSM-IV psychiatric comorbidity were excluded. The study patients had no history of drug abuse. A urine drug screening for opioids, methadone, amphetamines, cannabinoids, and sedatives was done on the day of admission to the detoxification unit, and only patients with negative drug screening results were included in the study. The mean age of the patients was 41.4 years (SD=7.7, range=30–58), and they had a mean of 9.5 years of education (SD=1.7, range=8–10). Their mean lifetime duration of alcohol dependence was 8.9 years (SD=3.8, range=3–14). Before abstinence, the mean amount of alcohol they consumed was 11.9 drinks per day (SD=5.0, range=5–20). Nine of the 10 patients were current smokers, and they had smoked for a mean of 20.7 years (SD=8.0, range=4–34). On average, they smoked 22.2 cigarettes per day (SD=9.4, range=10–35). The comparison group included five smokers who smoked a mean of 15.8 cigarettes per day (SD=5.31, range=10–20) and who had smoked for a mean of 24.6 years (SD=9.58, range=11–36). Furthermore, computerized tomography (CT) scans for all patients were evaluated before their inclusion in the study. The CT scans of five patients were judged to be unremarkable. Three patients had slight enlargements of internal and/or external ventricles. One patient had a large sinus frontalis sphenoidalis, and another had single falx calcifications. After inspection of three-dimensional scans during the MRI measurements, one healthy subject was excluded due to neuronal atrophy with ventricular enlargement.
Age and gender-matched healthy subjects were screened by comprehensive assessment procedures for medical, neurological, and psychiatric history and were free from any medical problems. The mean age of the healthy subjects was 41.1 years (SD=7.5, range=29–56), and they had a mean of 12.7 years of education (SD=4.0, range=8–18).
For the patient group, fMRI data were acquired no later than 1 week after detoxification from alcohol and then again after a 3-week period during which they underwent a standardized cognitive behavioral group therapy program that included controlled abstinence. For the comparison group, fMRI measurements were performed at two time points separated by 3 weeks. Before inclusion in the study, the patients underwent inpatient detoxification consisting of up to 2 weeks of treatment with disulfiram or doxepin and, if necessary, carbamazepine and/or haloperidol. The patients voluntary entered an open or closed detoxification unit, depending on their severity of withdrawal. After detoxification, patients were transferred to an inpatient psychiatric day-clinic program for patients with alcohol-related problems. During the 3-week treatment period (day clinic), the patients were randomly selected for testing with a breath-alcohol analyzer (Dräger, Lübeck, Germany) once daily in the detoxification unit to control alcohol abstinence. The cognitive behavioral group therapy program was conducted by licensed and well-experienced clinical psychologists. The 5-hour-per-week program involved relapse prevention, stimulus control, and educational interventions. The study patients were medication free during the 3-week treatment period except for a daily dose of 150 mg of doxepin.
Apparatus and Measurements
MRI imaging was performed with a 1.5-T imager (Siemens, Erlangen, Germany). A high-resolution sagittal three-dimensional dataset was acquired (matrix=256 × 256 × 128, TR=11.4 msec, TE=4.4 msec, field of view=230 mm). The intercommissural line (anterior commissure–posterior commissure) served as a reference for the position of the subsequent transaxial functional images covering the whole brain (echo-planar imaging, 70 measurements, slice thickness=3 mm, slice gap=0.3 mm, TR=10 seconds, TE=66 msec, matrix=64 × 64, field of view=200 mm, 32 slices, alpha=90°, acquisition time=4 seconds).
The experiment included two conditions: odor stimulation with corn spirit/ethanol (corn spirits, containing 38% ethanol by volume) and neutral stimulation with unmanipulated room air. After each condition, subjective ratings were assessed with the Positive and Negative Affect Schedule (20) and the Craving Rating Scale, a modified version of a German questionnaire used to elicit current experience of alcohol craving (21). The Craving Rating Scale is a 10-point unipolar intensity rating scale of descriptors for seven dimensions of craving: 1) the urge for alcohol, 2) withdrawal symptoms, 3) obsessional thoughts, 4) anxiety and depression, 5) irritability and anger, 6) positive affect, and 7) the goal of maintaining abstinence.
Each condition included four resting baseline periods of 100 seconds each and three 100-second periods consisting of a series of odor stimulus presentations (Figure 1). Each 100-second period (both baseline periods and odor stimulus presentation periods) consisted of 10 intervals of 10 seconds each. Because the pattern of activation during ethanol odor stimulation was not well understood at the beginning of the study, we chose the 10-second length for the odor stimulus interval. Each odor stimulus interval of 10 seconds started with 4 seconds of fMRI data acquisition. During the first 2 seconds, the olfactory stimulus (ethanol or air) was presented. The 4-second period of data acquisition was followed by an interstimulus pause of 6 seconds without stimulus presentation or data acquisition. Each 10-second interval during the 100-second baseline resting periods consisted of a 4-second period of fMRI data acquisition followed by a 6-second pause. Therefore, each condition comprised 70 whole brain acquisitions.
Both stimuli were presented to the subjects in the scanner by using an olfactometer designed by the authors (22). Each stimulus was delivered to the right nostril of each subject through a separate tube. The two stimuli were applied at same temperature and the same pressure at a rate of 50 ml/second.
Data Analysis
Data analysis was performed with statistical parametric mapping software (SPM 97d) (Department of Cognitive Neurology, University College London, London). Movement correction was done by using the 10th image in the time series as the reference image. The images were coregistered and resliced, and the sampling errors were adjusted (sinc interpolation). This correction reduces motion artifacts by a rigid-body transformation (translation in the direction of the x, y, and z axes and rotation around these three main axes), minimizing the total square difference between the images and the reference image. Three different brain volumes were found to have a spatial offset of more than one voxel in three different subjects, respectively. Since each of these brain volumes was measured during different study conditions and at different time points of the scanning session and since only single scans during the time course were affected, we did not remove the whole time series for these three subjects from the analyses. The mean image of the realigned data set of each experimental condition was superimposed onto the anatomical slices and coregistered with MPITOOL (V2-59) (MPI for Neurological Research, Cologne, Germany). For the superposition, we used the anterior commissure as a reference point. Realigned images were low-pass filtered with a Gaussian function (width=400 Hz). After stereotaxic normalization (resolution=4 × 4 × 4 mm3) and smoothing (6 × 6 × 6 mm3 Gaussian filter), statistical parametric maps were calculated by using the boxcar function, with the time course of stimuli presentation for the correlation analysis (with temporal smoothing active). Considering both our a priori hypothesis with regard to amygdala activation and our goal of increasing sensitivity, Bonferroni corrections were not applied. We considered corrections for multiple comparisons as unnecessarily conservative with respect to type I errors.
In view of our findings (23, 24) and the observations of others (25) that amygdala activation is less robust than activation in other regions, we set a threshold of z=1.64 (p=0.05) and a minimum cluster size (extent) of 1 voxel to avoid overlooking small areas of activation in this already small region. We used analysis of variance (ANOVA) to perform a second-order analysis of the relative signal change for the region of interest (amygdala) to confirm our statistical parametric mapping results. For this analysis, signal courses were extracted at the maxima of activation in patients before and after treatment as well as at the same locations in the comparison group. The change in relative signal intensity was calculated from the mean values for the baseline and activation periods by using the following formula: [SIACT–SIBL]/SIBL, where SIACT and SIBL refer to mean signal intensity during activation and baseline, respectively. These relative signal changes served as dependent measures in an repeated measures ANOVA with time (time 0, before treatment, and time 1, after treatment) as a repeated factor and group as a between-subject factor.
Results
Subjective Ratings
A two-factor ANOVA of patients’ scores on the Craving Rating Scale demonstrated a significant interaction between odor and time (F=6.06, df=1, 8, p=0.03) (Figure 2). The patients’ mean craving ratings at time 0, before treatment, were 17.0 (SD=4.4) for the ethanol condition and 14.7 (SD=4.2) for the neutral room air condition. At time 1, after treatment, the mean rating for ethanol decreased to 14.5 (SD=2.5), whereas the mean rating for the neutral odor remained much the same (mean=15.7, SD=3.1). The comparison subjects showed no significant effects. Since the comparison subjects were not required to respond to questions about the seven dimensions of the Craving Rating Scale, data for nonpatients and patients were analyzed separately.
The dependent measure for the Positive and Negative Affect Schedule was derived from the ratings of 10 items contributing to a positive and a negative emotional score (Table 1). The four-factor ANOVA for the Positive and Negative Affect Schedule (N=20) with group as a between-subject factor, and odor, time, and scale/mood valence as repeated factors yielded a significant main effect for group (F=7.99, df=1, 18, p=0.01), odor (F=8.61, df=1, 18, p=0.009), and Positive and Negative Affect Schedule score (F=58.73, df=1, 18, p=0.0001). However, a similar meaningful interaction (group-by-time-by-odor or time-by-odor) as for the Craving Rating Scale ratings did not emerge.
Measurements of Activation at Time 0
Within-group comparisons
In the alcoholic patients, the ethanol stimulus produced significant increases of the BOLD effect in the right amygdala/hippocampal area, the superior temporal gyrus, and the cerebellum (Table 2, Figure 3). The neutral room air stimulus produced no comparable activations. Only small activation clusters were seen in the medial frontal gyrus and the paracentral lobe. Comparison subjects demonstrated activation during the ethanol stimulus presentations only bilaterally in the superior temporal gyrus, without any evidence for subcortical-limbic activation (Figure 4). A smaller area of activation in the right temporal gyrus was also found during the perception of neutral room air.
The results of the second-order analysis for the amygdala revealed the expected group-by-time interaction for both local maxima (Table 2) (F=5.37, df=1, 18, p<0.04; and F=4.54, df=1, 18, p<0.05, respectively), confirming the results of the statistical parametric mapping analysis.
Between-group comparisons
Comparisons between groups were done by subtracting activation maps of the comparison subjects from those of the patients. For the ethanol condition, the comparison showed a small activation in the region of the amygdala and the cerebellum along with small activation areas in the superior and medial temporal lobe (Table 3). In a similar group comparison for neutral room air, activation was found only in the medial frontal gyrus and the paracentral lobule.
Measurements of Activation at Time 1
Within-group comparisons
In the patients, stimulation with ethanol no longer elicited activations in the amygdala or cerebellum (Figure 3). Brain activation was found in the superior temporal gyrus, similar to that of the first time point. Activity was additionally detected in the occipital cortex and the insula (Table 4). Neutral room air also showed signal increases in the superior temporal gyrus located more anterior. Further activations were observed in the occipital cortex, the frontal gyrus, and the precentral and cingulate gyrus. For comparison subjects, an increase in the extent and intensity of activation in the superior temporal lobe, compared with time 0, was found (Figure 4). Activations were also visible in the posterior cingulate. The neutral odor stimuli, on the other hand, showed activation only in the postcentral gyrus in comparison subjects.
Between-group comparisons
An analysis of activation maps of the differences between groups (patients minus comparison subjects) showed a greater left-lateralized activation in the parietal and occipital cortex and a greater left-sided temporal activation in patients than in the healthy subjects (Table 5>). Furthermore, activation in the right insula, left cerebellum, and left lenticular nucleus was found primarily in patients, compared to healthy subjects. Stimulation with neutral odor was associated with greater activation in the right medial frontal gyrus and the right superior temporal gyrus in patients than in the healthy subjects.
Discussion
To our knowledge, this is the first investigation illustrating specific neurobiological correlates of cue-induced alcohol craving in recently abstinent alcoholic patients. After detoxification but before treatment (time 0), the regions of the right amygdala/hippocampus complex and the cerebellum were specifically activated during olfactory stimulation with corn spirits/ethanol in the patients. Stimulation with neutral room air, on the other hand, did not show any activation in these regions in both patients and nonpatients. In addition, higher subjective ratings for craving were found during the ethanol condition at time 0 in the patients. Correspondingly, patients’ ratings on the Positive and Negative Affect Schedule showed higher positive and negative affect ratings for ethanol compared to neutral room air. However, these differences were not significant.
Correlates of Cue-Induced Craving
The amygdala represents a key structure within the limbic system. It is crucially involved in several forms of learning, as well as in the modulation and regulation of emotion. A number of functions have been attributed to the cerebellum, and there is growing evidence for its role in memory retrieval and learning (27). Hence, cerebellar participation may reflect aspects of memory and learning. We propose therefore that cue-induced craving, in this case ethanol-induced craving, may involve conditioned emotional reactions that are mediated by the amygdala, as well as learned memory associations that are mediated by the cerebellum. Alternatively, stimulation may have activated cerebellar functions involved in motor or multisensory coordination. Amygdala activation has also been found to represent a correlate of sad mood in healthy subjects (23, 24), and amygdala activation can be elicited with various emotional stimuli (25). In addition, the amygdala has been implicated in emotional learning (22, 28). The right lateralized activation found here corresponds to the proposed hemispheric lateralization of emotional processing suggested by the majority of reports. However, there is an ongoing discussion pertaining to laterality in the amygdala during affective processing.
Like studies of ethanol-induced craving in alcoholic patients, studies that have used cue-induced cocaine craving have suggested amygdala and cerebellum involvement. PET evaluation of cerebral blood flow in detoxified male cocaine users during cocaine-related videos showed increased activation in the amygdala and the anterior cingulate (11). Right amygdala activation has also been detected in response to visual smoking cues in smokers (29). Metabolic changes in the insula, the orbitofrontal cortex, and the cerebellum were, however, noted during a cocaine-theme interview (13). The lack of concordance generally found between studies of the neurobiological correlates of craving may be due to methodological issues, i.e., the variety of techniques for quantifying brain activation and for eliciting craving. Nevertheless, despite such inconsistencies, our results confirm some unifying features between cue-induced alcohol craving and cue-induced cocaine craving.
Cerebral Processing of Olfactory Stimuli
Activity in the bilateral superior temporal cortex was seen during ethanol stimulation in both healthy comparison subjects and alcohol-dependent patients. A similar, albeit slightly smaller activation was also observed in the superior temporal gyrus during stimulation with neutral room air in the comparison group. This activation may very well be a response to the olfactory stimulation. Our assumption is based on findings from magnetoencephalographic recordings that showed bilateral superior temporal cortex activation during olfactory stimulation (30). Observations in macaque monkeys have demonstrated a connection between the superior temporal cortex and the inferior orbitofrontal cortex, which is linked to the primary olfactory cortex (31). Contrary to other studies (32), participation of the orbitofrontal cortex could not be confirmed here. A susceptibility-related signal loss in the inferior frontal area or habituation effects due to our relatively long stimulation periods (100 seconds) are possible explanations for the difference in findings. Odor-induced activation may vary depending on olfactory stimulation parameters. For example, rapid habituation has been demonstrated after 30–40 seconds in the primary olfactory cortex despite continued olfactory stimulation, although continuous activation has been found in the orbitofrontal gyrus (33). However, our stimulation parameters were not designed with the intent of eliciting orbitofrontal activation.
Time-Dependent fMRI Effects
After behavioral therapy with antidepressant medication (time 1), the mapping of brain activity during ethanol stimulation disclosed a pattern of activation more similar to that in the nonpatient group. After therapy, regional activations were found in the left superior temporal sulcus and the right insula and, to a lesser extent, in the right occipital cortex. Specific activations in the amygdala or cerebellum were no longer evident. These findings suggest that the correlates of cue-induced alcohol craving (activation in the amygdala and cerebellum) are state dependent and may be influenced by abstinence from alcohol and/or therapeutic interventions. Assuming that conditioning processes of emotion and memory play a role in cue-induced craving, our results strongly suggest extinction of conditioned responses. Additional activation in the insula, which is strongly associated with the limbic system and is functionally relevant in visceral function, olfaction, taste, and emotion, may represent a more cortical influence in the processing of alcohol-related cues. It may also represent a lasting correlate of craving, as has been reported earlier (13). Although visual stimulation was not included in this experiment, a state of increased attention may have elicited the occipital activation in the patient group.
Antidepressants have been shown to reduce depressive symptoms and to decrease alcohol consumption among alcoholic patients (34). Serotonergic dysfunction in alcoholism and symptoms of depression during withdrawal have been supported in SPECT studies (35). Whether the change in activation pattern in our patient group was due to the effect of the pharmacological or psychological intervention or of abstinence itself cannot be clearly defined. A combination of the two may have been involved. Future studies with unmedicated patients are required to differentiate between the effects of psychological and pharmacological intervention on craving.
The superior temporal cortex activation at time 1 in the comparison group corresponded to the measurements at time 0, albeit with increased intensity and extent at time 1. We believe that this response reflects a sensitization or recognition effect. Earlier fMRI evidence had shown an overall heightened activation with repeated testing within 1 week of odorant stimulation of the trigeminal nerve, which was similarly noticed in the superomedial temporal lobe (32). Odorants that stimulate the trigeminal nerve may subject an individual to enhanced irritation, danger, or discomfort, compared to odorants that do not stimulate the trigeminal nerve. One might theorize that this evolutionary adaptation triggers and incorporates additional cortical resources. The alcohol concentration used in our odorant stimulus was seemingly high. Although the threshold for nasal pungency for ethanol is high compared to other, longer-chained alcohols (log of nasal pungency threshold=3.9 [36]), nasal irritation was nevertheless caused. The nasal pungency threshold had been determined in anosmic patients, rendering it free from an olfactory component. Hence, it appears that the larger activation seen at time 1 was brought on by the irritating quality of the stimulus. Alternatively, preexposing subjects to the stimulus at time 0 may have led to recognition of the ethanol odor. Previous stimulus exposure may have thus evoked activation in neighboring areas involved in memory as well as olfactory processing. On the other hand, a similar heightened activation was not shown in the patients. One explanation may be that ethanol stimuli produce different cognitive associations with different modes of processing in the patients. Also, the stimuli may have led to different attention processes with differential activation patterns in the patients and nonpatients.
Some variations in activation between subject groups and measurement time points were recognized during neutral air stimulation. This is probably due to the expectations that arise from an odorant stimulus that is perceived but is difficult to identify.
Methodological Constraints
The limitations of the study are related to methodological constraints. The experimental design did not include randomization of the ethanol and neutral odor stimuli within one stimulation condition because the signal course of ethanol stimulation was not obvious at the time the study was initiated. Moreover, we were interested in avoiding a crossover effect of the two stimuli. Therefore, we used a fixed-effect model for the analysis, making it difficult to rule out the possibility that the subjects might have developed expectancies with regard to the stimuli. Furthermore, because this was a pilot study that did not include a second treatment comparison group, we cannot exclude the likelihood that our results reflected a time factor rather than treatment effects.
Conclusions
The study findings support the existence of specific neurobiological substrates of cue-induced alcohol craving in alcohol-abstinent patients. Moreover, these substrates appear to be state dependent. The amygdalar and cerebellar activations observed before therapy in only the alcohol-abstinent patients may represent aspects of emotion, motivation, and memory in cue-induced craving.
![]() |
![]() |
![]() |
![]() |
![]() |
Received July 20, 2000; revision received Jan. 5, 2001; accepted Jan. 11, 2001. From the Department of Psychiatry and Psychotherapy, University of Düsseldorf; the Department of Psychiatry, University of Bonn, Bonn, Germany; and the Institute of Medicine, Research Center Jülich, Jülich, Germany. Address reprint requests to Dr. Schneider, Department of Psychiatry, University of Düsseldorf, Bergische Landstr. 2, 40629 Düsseldorf, Germany; [email protected] (e-mail). Supported in part by a grant from the Deutsche Forschungsgemeinschaft (Schn 362/7-1). The authors thank S. Albrecht and E. Sohne for clinical support and B. Elghawaghi and M. Grosse-Ruyken for assistance in data acquisition.
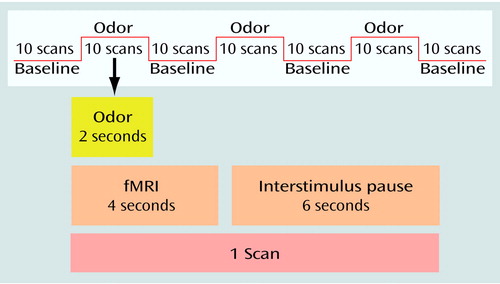
Figure 1. Sequence of Stimuli Presentation and Whole Brain Scans in an fMRI Study of Cerebral Correlates of Craving in 10 Recently Abstinent Alcoholic Patients and 10 Healthy Comparison Subjectsa
aEach baseline period and odor stimulus presentation period consisted of 10 10-second intervals that included a 4-second fMRI scan and a 6-second interstimulus pause. In the experimental condition, ethanol odor (corn spirits containing 38% ethanol by volume) was presented for the first 2 seconds of each odor stimulus interval. In the control condition, neutral room air was presented for the first 2 seconds of the odor stimulus interval.
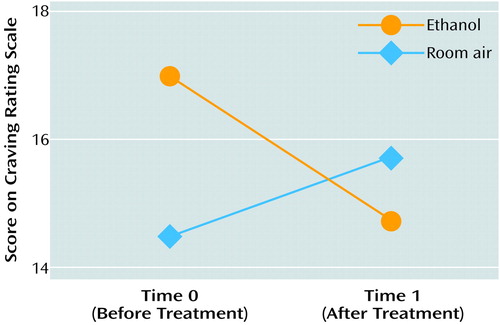
Figure 2. Mean Scores on the Craving Rating Scale of 10 Recently Abstinent Alcoholic Patients During Olfactory Stimulation With Ethanol Odor and Neutral Room Air Before and After Treatmenta
aThree weeks of inpatient treatment that included 5 hours per week of standardized group behavior therapy and 150 mg/day of doxepin.
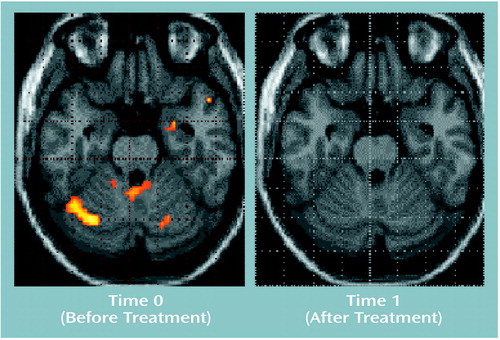
Figure 3. Areas of Activationa in Amygdala and Cerebellum in 10 Recently Abstinent Alcoholic Patients During Olfactory Stimulation With Ethanol Odor Before and After Treatmentb
aImages are statistical parametric maps showing regions of activation during olfactory stimulation with ethanol odor (z score=1.64, p<0.05).
bThree weeks of inpatient treatment that included 5 hours per week of standardized group behavior therapy and 150 mg/day of doxepin.
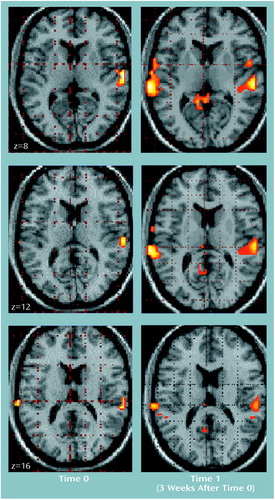
Figure 4. Superior Temporal Areas of Activation in 10 Healthy Comparison Subjects During Olfactory Stimulation With Ethanol Odor at Two Time Pointsa
aImages are statistical parametric maps showing regions of activation during olfactory stimulation with ethanol odor (z score=1.64, p<0.05); z values (8, 12, and 16) are coordinates in Talairach space (26).
1. Ludwig AM, Wikler A, Stark LH: The first drink: psychobiological aspects of craving. Arch Gen Psychiatry 1994; 30:539-547Crossref, Google Scholar
2. McLellan AT, Childress AR, Ehrman R, O’Brien CP, Pashko S: Extinguishing conditioned responses during opiate dependence treatment turning laboratory findings into clinical procedures. J Subst Abuse Treat 1986; 3:33-40Crossref, Medline, Google Scholar
3. Katner SN, Magalong JG, Weiss F: Reinstatement of alcohol-seeking behavior by drug-associated discriminative stimuli after prolonged extinction in the rat. Neuropsychopharmacology 1999; 20:471-479Crossref, Medline, Google Scholar
4. Le AD, Quan B, Juzytch W, Fletcher PJ, Joharchi N, Shaham Y: Reinstatement of alcohol-seeking by priming injections of alcohol and exposure to stress in rats. Psychopharmacology (Berl) 1998; 135:169-174Crossref, Medline, Google Scholar
5. Weinstein A, Lingford-Hughes A, Martinez-Raga J, Marshall J: What makes alcohol-dependent individuals early in abstinence crave for alcohol: exposure to the drink, images of drinking, or remembrance of drinks past? Alcohol Clin Exp Res 1998; 22:1376-1381Google Scholar
6. Sinha R, Krishnan-Sarin S, O’Malley S: Stress response and stress-induced craving in alcohol dependent individuals (abstract). Alcohol Clin Exp Res 1997; 21(suppl):96Google Scholar
7. Cooney NL, Baker LH, Pomerleau OF, Josephy B: Salivation to drinking cues in alcohol abusers: toward the validation of a physiological measure of craving. Addict Behav 1984; 9:91-94Crossref, Medline, Google Scholar
8. Cooney NL, Gillespie RA, Baker LH, Kaplan RF: Cognitive changes after alcohol cue exposure. J Consult Clin Psychol 1987; 55:150-155Crossref, Medline, Google Scholar
9. Stormark KM, Laberg JC, Bjerland T, Nordby H, Hugdahl K: Autonomic cued reactivity in alcoholics: the effect of olfactory stimuli. Addict Behav 1995; 20:571-584Crossref, Medline, Google Scholar
10. McCusker CG, Brown K: Cue-exposure to alcohol-associated stimuli reduces autonomic reactivity, but not craving and anxiety, in dependent drinkers. Alcohol Alcohol 1995; 30:319-327Medline, Google Scholar
11. Childress AR, Mozley PD, McElgin W, Fitzgerald J, Reivich M, O’Brien CP: Limbic activation during cue-induced cocaine craving. Am J Psychiatry 1999; 156:11-18Link, Google Scholar
12. Grant S, London ED, Newlin DB, Villemagne VL, Liu X, Contereggi C, Phillips RL, Kimes AS, Margolin A: Activation of memory circuits during cue-elicited cocaine craving. Proc Natl Acad Sci USA 1996; 93:12040-12045Google Scholar
13. Wang GJ, Volkow ND, Fowler JS, Cervany P, Hitzemann RJ, Pappas NR, Wong CT, Felder C: Regional brain metabolic activation during craving elicited by recall of previous drug experiences. Life Sci 1999; 64:775-784Crossref, Medline, Google Scholar
14. Rolls ET: The orbitofrontal cortex and reward. Cereb Cortex 2000; 10:284-294Crossref, Medline, Google Scholar
15. Schmahmann JD: The role of the cerebellum in affect and psychosis. J Neurolinguistics 2000; 13:189-214Crossref, Google Scholar
16. Hommer DW: Functional imaging of craving. Alcohol Res Health 1999; 23:187-196Medline, Google Scholar
17. Modell JG, Mountz JM: Focal cerebral blood flow change during craving for alcohol measured by SPECT. J Neuropsychiatry Clin Neurosci 1995; 7:15-22Crossref, Medline, Google Scholar
18. Hommer D, Andreason P, Rio D, Williams W, Ruttimann U, Momenan R, Zametkin A, Rawlings R, Linnoila M: Effects of m-chlorophenylpiperazine on regional brain glucose utilization: a positron emission tomographic comparison of alcoholic and control subjects. J Neurosci 1997; 17:2796-2806Google Scholar
19. Bucholz KK, Cadoret R, Cloninger CR, Dinwiddie SH, Hesselbrock VM, Nurnberger JI, Reich T, Schmidt I, Schuckit MA: A new, semi-structured psychiatric interview for use in genetic linkage studies: a report on the reliability of the SSAGA. J Stud Alcohol 1994; 55:149-158Crossref, Medline, Google Scholar
20. Watson D, Clark LA, Tellegen A: Development and validation of brief measures of positive and negative affect: the PANAS scales. J Pers Soc Psychol 1988; 54:1063-1070Google Scholar
21. Szegedi A, Lörch B, Scheurich A, Ruppe A, Hautzinger M, Wetzel H: Cue exposure in alcohol dependent patients: preliminary evidence for different types of cue reactivity. J Neural Transm 2000; 107:721-730Crossref, Medline, Google Scholar
22. Schneider F, Weiss U, Kessler C, Müller-Gärtner H-W, Posse S, Salloum JB, Grodd W, Himmelmann F, Gaebel W, Birbaumer N: Subcortical correlates of differential classical conditioning of aversive emotional reactions in social phobia. Biol Psychiatry 1999; 45:863-871Crossref, Medline, Google Scholar
23. Schneider F, Weiss U, Kessler C, Salloum JB, Posse S, Grodd W, Müller-Gärtner H-W: Differential amygdala activation in schizophrenia during sadness. Schizophr Res 1998; 34:133-142Crossref, Medline, Google Scholar
24. Schneider F, Habel U, Kessler C, Salloum JB, Posse S: Gender differences in regional cerebral activity during sadness. Hum Brain Mapp 2000; 7:226-238Crossref, Google Scholar
25. Irwin W, Davidson RJ, Lowe MJ, Mock BJ, Sorenson JA, Turski PA: Human amygdala activation detected with echo-planar functional magnetic resonance imaging. Neuroreport 1996; 7:1765-1769Google Scholar
26. Talairach J, Tournoux P: Co-Planar Stereotaxic Atlas of the Human Brain: Three-Dimensional Proportional System. Stuttgart, Germany, Georg Thieme, 1988Google Scholar
27. Buckner RL, Raichle ME, Miezin FM, Petersen SE: Functional anatomic studies of memory retrieval for auditory words and visual pictures. J Neurosci 1996; 16:6219-6235Google Scholar
28. LaBar KS, Gatenby JC, Gore JC, LeDoux JE, Phelps EA: Human amygdala activation during conditioned fear acquisition and extinction: a mixed-trial fMRI study. Neuron 1998; 20:937-945Crossref, Medline, Google Scholar
29. Due DL, Hall WG, Rubin DC: Smoking cues induce neural activation in deprived smokers (abstract). Neuroimage 2000; 11:S37Google Scholar
30. Kettenmann B, Jousmaki V, Portin K, Salmelin R, Kobal G, Hari R: Odorants activate the human superior temporal sulcus. Neurosci Lett 1996; 203:143-145Crossref, Medline, Google Scholar
31. Carmichael ST, Price LJ: Connectional networks within the orbital and medial prefrontal cortex of macaque monkeys. J Comp Neurol 1996; 371:179-207Crossref, Medline, Google Scholar
32. Yousem DM, Williams SC, Howard RO, Andrew C, Simmons A, Allin M, Geckle RJ, Suskind D, Bullmore ET, Brammer MJ, Doty RL: Functional MR imaging during odor stimulation: preliminary data. Radiology 1997; 204:833-838Crossref, Medline, Google Scholar
33. Sobel N, Prabhakaran V, Zhao Z, Desmond JE, Glover GH, Sullivan E, Gabrieli DE: Time course of odorant-induced activation in the human primary cortex. J Neurophysiol 2000; 83:537-551Crossref, Medline, Google Scholar
34. Naranjo CA, Poulos CX, Lanctôt KL, Bremner KE, Kwok M, Umana M: Ritanserin, a central 5-HT2 antagonist, in heavy social drinkers: desire to drink, alcohol intake and related affects. Addiction 1995; 90:893-905Crossref, Medline, Google Scholar
35. Heinz A, Ragan P, Jones DW, Hommer D, Williams W, Knable MB, Gorey JG, Doty L, Geyer C, Lee KS, Coppola R, Weinberger DR, Linnoila M: Reduced central serotonin transporters in alcoholism. Am J Psychiatry 1998; 155:1544-1549Google Scholar
36. Cometto-Muniz JE, Cain WS: Thresholds for odor and nasal pungency. Physiol Behav 1990; 48:719-725Crossref, Medline, Google Scholar